Bone Density
A Key Determinant for Treatment Planning
Carl E. Misch
Available bone is particularly important in implant dentistry and describes the external architecture or volume of the edentulous area considered for implants.1 Historically, the available bone was not modified in the implant candidate. Instead, the existing bone volume was the primary factor used to develop a treatment plan. Short implants and fewer implants were used in less available bone, and long implants in greater numbers were inserted in larger bone volumes.2 Today, the treatment plan should first consider the final prosthesis options and determine the prosthesis type indicated for the specific patient in question. The key implant positions for the prosthesis may then be determined by a biomechanical perspective. The additional number of implants required to support the specific restoration may then be established related to the amount of force generated by the patient (patient force factors) because stress equals force divided by area. The next consideration to determine the additional implant number and size to support the restoration is the bone density in the sites of the implant abutments.3
The external (cortical) and internal (trabecular) structure of bone may be described in terms of quality or density, which reflects a number of biomechanical properties, such as strength, modulus of elasticity, bone–implant contact (BIC) percent, and stress distribution around a loaded endosteal implant (Box 11-1). In other words, the external and internal architecture of bone controls virtually every facet of the practice of implant dentistry. The density of available bone in an edentulous site is a determining factor in treatment planning, surgical approach, implant design, healing time, and the need for initial progressive bone loading during prosthetic reconstruction.4–7 This chapter presents the aspects of bone density related to overall planning of an implant prosthesis.
Influence of Bone Density on Implant Success Rates
The quality of bone often depends on the arch position.4,8,9 The most dense bone is usually observed in the anterior mandible followed by the anterior maxilla and posterior mandible, and the least dense bone is typically found in the posterior maxilla (Box 11-2). A range of implant survival has been found relative to arch location. Following a standard surgical and prosthetic protocol, Adell et al.2 reported an approximate 10% greater success rate in the anterior mandible as compared with the anterior maxilla. In a 15-year follow-up study, Snauwaert et al.10 reported that early annual and late failures were also more frequently found in the maxilla.
In addition to arch location, several independent groups have reported different failure rates related to the quality of the bone. In fact, reduced implant survival most often is more related to bone density than arch location. Engquist et al.11 observed that 78% of all reported implant failures were in soft bone types when they supported overdentures. Friberg et al.12 observed that 66% of their group’s implant failures occurred in the resorbed maxilla with soft bone. Jaffin and Berman,13 in a 5-year study, reported a 44% implant failure when poor-density bone was observed in the maxilla and 35% implant loss in any region of the mouth when bone density was poor, with 55% of all implant failures in the soft bone type. Johns et al.14 reported 3% failure of implants in moderate bone densities but a 28% implant failure in the poorest bone type. Smedberg et al.15 reported a 36% failure rate in the poorest bone density. Herrmann et al.16 found that implant failures were strongly correlated to patient factors, including bone quality, especially when coupled with poor bone volume (65% of these patients experienced failure). These reported failures are not primarily related to surgical healing but instead occur after prosthetic loading. Therefore, over the years, many independent clinical groups, following a standardized surgical and prosthetic protocol, documented the indisputable influence of bone density on clinical success17–19 (Figure 11-1).
A protocol established by the author in 1988, which adapts the treatment plan, implant selection, surgical approach, healing regimen, and initial prosthetic loading, has resulted in similar implant success rates in all bone densities and all arch positions.4 Multicenter clinical reports by Misch et al. and Kline et al. confirm implant success rates with this protocol may be similar in all bone density types and arch locations20–23 (Figure 11-2). This chapter proposes a scientific rationale for the modification of a treatment plan in function of bone density to achieve comparable success rates in all bone types.
Etiology of Variable Bone Density
Bone is an organ that is able to change in relation to a number of factors, including hormones, vitamins, and mechanical influences. However, biomechanical parameters, such as the amount of strain transmitted to bone, are predominant.24 Awareness of this adaptability in the skeletal system has been reported for more than a century. In 1887, Meier qualitatively described the architecture of trabecular bone in the femur.25 In 1888, Kulmann noticed the similarity between the pattern of trabecular bone in the femur and tension trajectories in construction beam concepts used by Eiffel26 (Figure 11-3). Wolff, in 1892, further elaborated on these concepts and published: “Every change in the form and function of bone or of its function alone is followed by certain definite changes in the internal architecture, and equally definite alteration in its external conformation, in accordance with mathematical laws.”27 The modified function of bone and the definite changes in the internal and external formation of the vertebral skeleton as influenced by mechanical load were also reported more recently by Murry.28 Therefore, it has been widely reported in the structural skeleton that the external architecture of bone (cortical bone) changes in relation to function, and the internal bony structure (trabecular bone) is also modified.
The structural changes in bone as a consequence of mechanical influences have also been noted in the jaws. As an example, both MacMillan and Parfitt have reported on the structural characteristics and variation of trabeculae in the alveolar regions of the jaws.29,30 The maxilla and mandible have different biomechanical functions (Figure 11-4). The mandible, as an independent structure, is designed as a force absorption unit. Therefore, when teeth are present, the outer cortical bone is denser and thicker, and the trabecular bone is more coarse and dense (Figure 11-5). On the other hand, the maxilla is a force distribution unit. Stresses to the maxilla are transferred by the zygomatic arch and palate away from the brain and orbit. As a consequence, the maxilla has a thin cortical plate and fine trabecular bone surrounding the teeth (Figure 11-6). Neufeld noted that the bone is most dense around the teeth (cribriform plate) and more dense around the teeth at the crest compared with the regions around the apices31 (Figure 11-7) Alveolar bone resorption associated with orthodontic therapy also illustrates the biomechanical sensitivity of the alveolar processes.32
Orban demonstrated a decrease in the trabecular bone pattern around a maxillary molar with no opposing occlusion compared with a tooth with occlusal contacts on the contralateral side33 (Figure 11-8). Bone density in the jaws also decreases after tooth loss. This loss is primarily related to the length of time the region has been edentulous and not loaded appropriately, the initial density of the bone, flexure and torsion in the mandible, and parafunction before and after tooth loss.34–37 In general, the density change after tooth loss is greatest in the posterior maxilla and least in the anterior mandible.
Cortical and trabecular bone throughout the body are constantly modified by either modeling or remodeling.38 Modeling has independent sites of formation and resorption and results in the change of the shape or size of bone. Remodeling is a process of resorption and formation at the same site that replaces previously existing bone and primarily affects the internal turnover of bone, including that region where teeth are lost or the bone next to an endosteal implant. These adaptive phenomena have been associated with the alteration of the mechanical stress and strain environment within the host bone.39
Stress is determined by the magnitude of force divided by the functional area over which it is applied. Strain is defined as the change in length of a material divided by the original length. The greater the magnitude of stress applied to the bone, the greater the strain observed in the bone.40 Bone modeling and remodeling are primarily controlled, in part or whole, by the mechanical environment of strain. Overall, the density of alveolar bone evolves as a result of mechanical deformation from microstrain.
Frost proposed a model of modeling/remodeling patterns for compact bone as it relates to mechanical adaptation to strain.41 Spontaneous fracture, the pathologic overload zone, mild overload zone, adapted window, and acute disuse window were described for bone in relation to the amount of the microstrain experienced (Box 11-3). These categories also may be used to describe the trabecular bone response next to a dental implant in the jaws.42–44
The bone in the acute disuse window loses mineral density, and disuse atrophy occurs because modeling for new bone is inhibited and remodeling is stimulated, with a gradual net loss of bone (Figure 11-9). The microstrain of bone for trivial loading is reported to be 0 to 50 microstrain.41 This phenomenon may occur throughout the skeletal system, as evidenced by a 15% decrease in the cortical plate and extensive trabecular bone loss consequent to immobilized limbs for 3 months.45 A cortical bone density decrease of 40% and a trabecular bone density decrease of 12% also have been reported with disuse of bone.46,47 Interestingly, bone loss similar to disuse atrophy has been associated with microgravity environments in outer space because the microstrain in bone resulting from the earth’s gravity is not present in the “weightless” environment of space.48 In fact, an astronaut aboard the Russian Mir space station for 111 days lost nearly 12% of his bone minerals.49,50
The adapted window (50–500 microstrain) represents an equilibrium of modeling and remodeling, and bone conditions are maintained at this level.41 Bone in this strain environment remains in a steady state, and this may be considered the homeostatic window of health. The histologic description of this bone is primarily lamellar or load-bearing bone. Approximately 18% of trabecular bone and 2% to 5% of cortical bone is remodeled each year in the physiologic loading zone, which corresponds to the adapted window.24 This is the range of strain ideally desired around an endosteal implant after a stress equilibrium has been established. Bone turnover is required in the adapted window; Mori and Burr provide evidence of remodeling in regions of bone microfracture from fatigue damage within the physiologic range.51
The mild overload zone (1500–3000 microstrain) causes a greater rate of fatigue microfracture and increase in the cellular turnover rate of bone.41 As a result, the bone strength and density decrease. The histologic description of bone in this range is usually woven or repair bone. Woven bone is able to form faster but is less mineralized and less organized than lamellar bone. This may be the state for bone when an endosteal implant is overloaded and the bone interface attempts to adapt to the greater strain environment.44 During the repair process, the woven bone is weaker than the more mature, mineralized lamellar bone.42 Therefore, although bone is loaded in the mild overload zone, care must be taken because the “safety range” for bone strength is reduced during the repair.
Pathologic overload zones are reached when microstrains are greater than 3000 units.41 Cortical bone fractures occur at 10,000 to 20,000 microstrain (1%–2% deformation). But pathologic overload may begin at microstrain levels of only 20% to 40% of the ultimate strength or physical fracture of cortical bone. The bone may resorb and form fibrous tissue or, when present, repair woven bone is observed in this zone because a sustained turnover rate is necessary. The marginal bone loss evidenced during implant overloading may be a result of the bone in the pathologic overload zone. Implant failure from overload may also be a result of bone in the pathologic overload zone.
Bone Classification Schemes Related to Implant Dentistry
An appreciation of bone density and its relation to oral implantology has existed for more than 25 years. Linkow, in 1970, classified bone density into three categories52:
Linkow stated that class III bone results in a loose fitting implant, class II bone was satisfactory for implants, and class I bone was the most ideal foundation for implant prostheses.
In 1985, Lekholm and Zarb listed four bone qualities found in the anterior regions of the jawbone53 (Figure 11-10). Quality 1 was composed of homogeneous compact bone. Quality 2 had a thick layer of compact bone surrounding a core of dense trabecular bone. Quality 3 had a thin layer of cortical bone surrounding dense trabecular bone of favorable strength. Quality 4 had a thin layer of cortical bone surrounding a core of low-density trabecular bone. Irrespective of the different bone qualities, all bone was treated with the same implant design and standard surgical and prosthetic protocol.2 Following this protocol, Schnitman et al. and others observed a 10% difference in implant survival between quality 2 and quality 3 bone and a 22% lower survival rate in the poorest bone density.54 As previously presented, many authors also experienced a greater failure in soft bone.11–19,54–56 It is obvious that a standardized surgical, prosthetic, and implant design protocol does not yield similar results in all bone densities. In addition, these reports are for implant survival, not the quality of health of surviving implants. The amount of crestal bone loss also has been related to bone density and further supports a different protocol for soft bone.57–59
In 1988, Misch proposed four bone density groups independent of the regions of the jaws based on macroscopic cortical and trabecular bone characteristics.4,5 The different anterior and posterior regions of the jaws often had densities related from one patient to another, but ranges of bone density may be found in any location. Suggested treatment plans, implant design, surgical protocol, healing, and progressive loading time spans have been described for each bone density type.3,6 Following this regimen, similar implant survival rates have been observed for all bone densities.20–23
Misch Bone Density Classification
Dense or porous cortical bone is found on the outer surfaces of bone and includes the crest of an edentulous ridge. Coarse and fine trabecular bone types are found within the outer shell of cortical bone and occasionally on the crestal surface of an edentulous residual ridge. These four macroscopic structures of bone may be arranged from the most dense to the least dense, as first described by Frost and by Roberts: dense cortical bone, porous cortical bone, coarse trabecular bone, and fine trabecular bone41,42 (Figure 11-11).
In combination, these four macroscopic densities constitute the four bone categories described by Misch (D1, D2, D3, and D4) located in the edentulous areas of the maxilla and mandible (Table 11-1; Figure 11-12). The regional locations of the different densities of cortical bone are more consistent than the highly variable trabecular bone.
TABLE 11-1
Misch Bone Density Classification Scheme
Bone Density | Description | Tactile Analog | Typical Anatomical Location |
D1 | Dense cortical | Oak or maple wood | Anterior mandible |
D2 | Porous cortical and coarse trabecular | White pine or spruce wood | Anterior mandible Posterior mandible Anterior maxilla |
D3 | Porous cortical (thin) and fine trabecular | Balsa wood | Anterior maxilla Posterior maxilla Posterior mandible |
D4 | Fine trabecular | Styrofoam | Posterior maxilla |
D1 bone is primarily dense cortical bone. D2 bone has dense to porous cortical bone on the crest and lateral to the implant site. The bone within this cortical housing has coarse trabecular bone (Figure 11-13). D3 bone types have a thinner porous cortical crest and facial/lingual regions, with fine trabecular bone in the region next to the implant (Figure 11-14). D4 bone has almost no crestal cortical bone and porous cortical lateral plates. The fine trabecular bone comprises almost all of the total volume of bone next to the implant (Figure 11-15). A very soft bone, with incomplete mineralization and large intratrabecular spaces, may be addressed as D5 bone (Figure 11-16). This bone type is found often in the immature bone of a developing bone graft site. The bone density may be determined by the general location, tactile sense during surgery, or computerized radiographic evaluation.
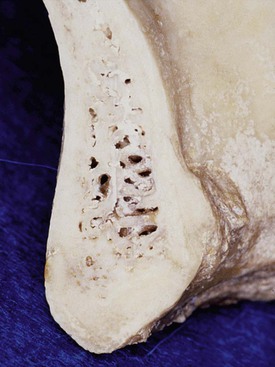
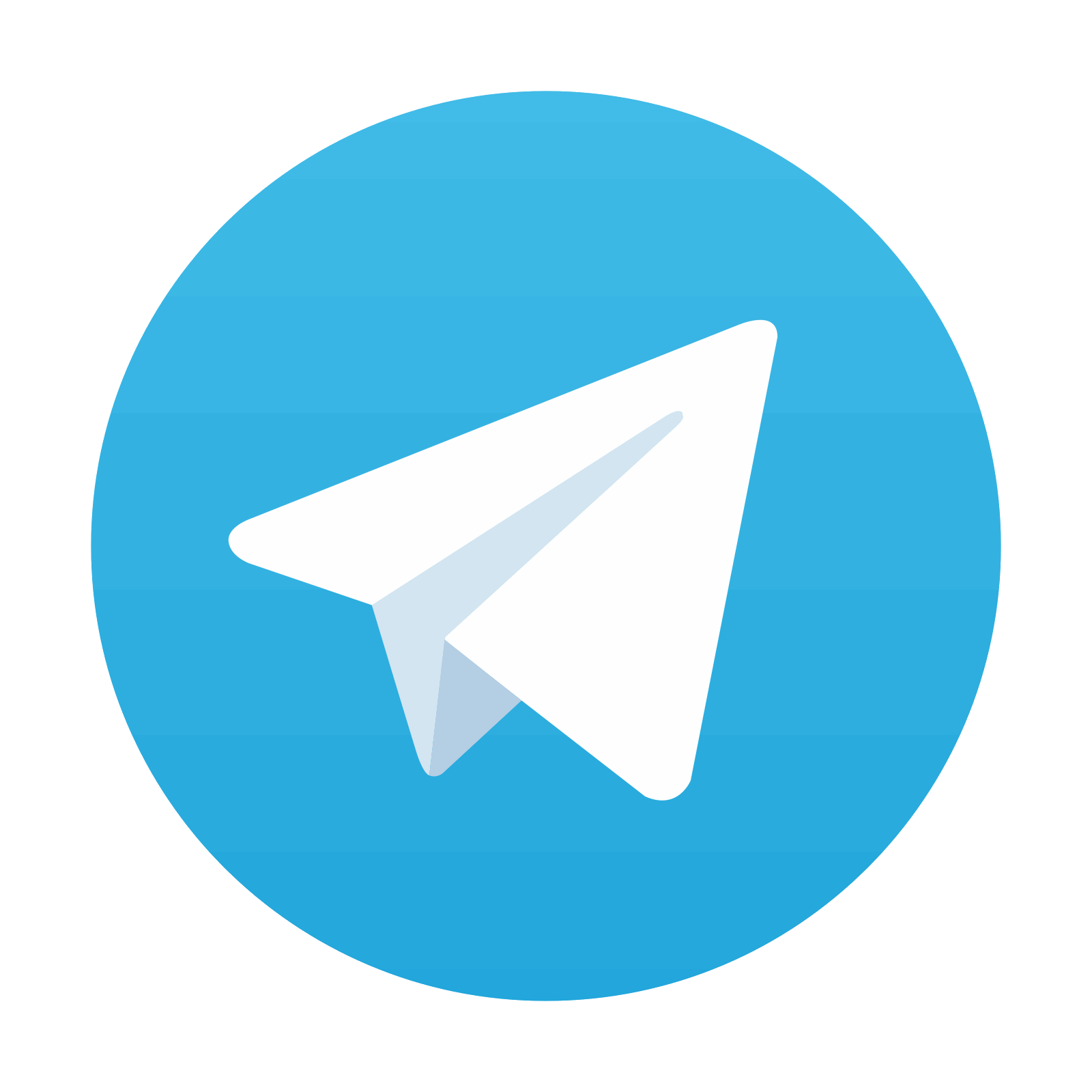
Stay updated, free dental videos. Join our Telegram channel

VIDEdental - Online dental courses
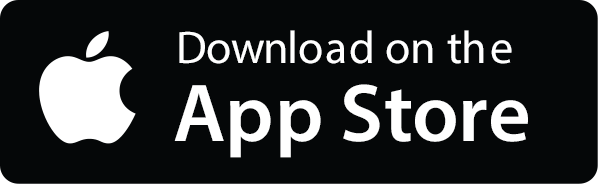
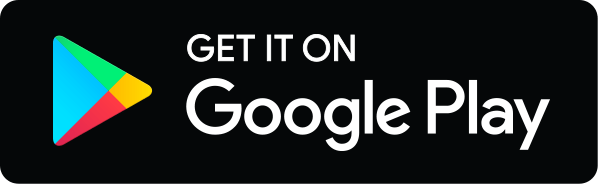