1
The Endodontium
Structure
The endodontium comprises the dentin and pulp of the tooth. Both tissues develop from the dental papilla, and although the dentin mineralizes and the pulp remains a soft tissue, they maintain an intimate structural and functional relationship throughout the life of the tooth.
All the cells of the endodontium are located in the pulp and only cellular extensions, odontoblast processes, and nerve endings are found in the dentin. Thus, tissue reactions in the dentin are dependent to a great extent on the activity of cells in the pulp. Conversely, pulpal reactions may be significantly modified by tissue changes in the dentin.
The Dentin
Composition and Morphology
The dentin is composed of approximately 70% inorganic material in the form of hydroxyapatite crystals. The organic matrix, about 15–20%, consists of collagen. Noncollagenous proteins constitute 1–2% of the tissue, whereas the remaining 10–12% is water.
The dentin of the fully formed tooth is called primary dentin. It constitutes the bulk of the tooth and is especially characterized by the presence of dentinal tubules (Fig. 1.1). The tubules generally extend from the area of the dentin–enamel and the dentin–cementum junctions to the pulp. The tubules are surrounded by peritubular dentin, which is a dense, highly mineralized tissue with a noncollagenous matrix. Between the tubules we find the intertubular dentin, which consists of mineralized collagen. Unmineralized predentin lines the pulpal aspect of the dentin.
Fig. 1.1 Scanning electron micrographs of fractured coronal dentin from an impacted canine in a 13-year-old.
a Dentin from the middle area of the crown with crosscut dentinal tubules (diameter: 2 μm). Fibers are seen leaving some of the tubules (× 1700).
b Dentin near the enamel–dentin junction (mantle dentin) with crosscut dentinal tubules (arrows). Note small diameter (0.5 μm) of the tubules (× 1700).
c Predentinal surface with intertubular fibrous matrix (× 2600).
Unmineralized matrix may also be seen inside the mineralized primary dentin. Well known is interglobular dentin, which occurs when mineralizing globules fail to coalesce. From a clinical point of view, it is more important that the buccal and lingual portions of the incisal dentin not always unite, but leave an unmineralized central streak or a soft tissue-containing space, sometimes extending all the way to the incisal dentin–enamel junction (Fig. 1.2). Clearly, in such teeth, an apparent uncomplicated crown fracture will cause exposure of the pulp.
Dentinogenesis continues, but at a slower rate, even after the teeth are fully formed. This dentin is called physiologic secondary dentin and it differs from the primary dentin in that its structure and composition may vary within the tooth and from one tooth to the next. As will be discussed later (see p. 26), increased secondary dentin formation in localized areas of the tooth may occur in response to external irritation. The structure of this tissue will depend on the severity of the irritation and the degree of tissue injury in the pulp and appears to be completely unpredictable (Fig. 1.3). As a rule, the secondary dentin formed in response to external irritants is more irregular than the physiologic secondary dentin.
Fig. 1.2 Microradiograph of an incisor from an 11-year-old. Accentuated incremental lines of buccal and lingual dentin (arrows) meet at the radiopaque central streak, but do not join because of a slit in the dentin at this location.
Dentinal Tubules
It is well established that the dentinal tubules may serve as portals of entry for external irritants into the pulp. Thus, from a clinical point of view, the tubules are the most important and interesting component of the dentin.
In the crown of the tooth, the dentinal tubules generally extend from the area of the dentin–enamel junction to the pulp. In the root, the most peripheral dentin is atubular and the tubules thus begin in an area slightly pulpal to the dentin–cementum junction and extend to the pulp. The diameter of the tubules varies from 0.5 μm in the peripheral dentin to 3–4 μm near the pulp. In the bulk of the dentin they have a diameter of about 2 μm. Due to the much larger peripheral than pulpal surface of the dentin, the number of tubules per square millimeter area increases dramatically in a pulpal direction. Thus, at the dentin–enamel junction, the number of tubules is about 8000 per mm2, halfway between the dentin–enamel junction and the pulp it is 20 000–30 000 per mm2, and near the pulp the number may be as high as 50 000–60 000 per mm2. Similarly, the total volume of the dentinal tubules increases in a pulpal direction and may constitute up to 80% of the total volume of the coronal dentin near the pulp.
The dentinal tubules contain tissue fluids (dentin liquor) which is fluid from the pulp tissue filling out the hollows of the dentin. Odontoblastic processes are present in most tubules and they are especially well visualized close to the pulp. Unmyelinated nerve endings may be present as well, usually in intimate contact with the odontoblastic processes (Fig. 1.4). In addition, unmineralized and mineralized collagen fibers are seen in many tubules at all levels of the dentin (Fig. 1.5).
Mineralized deposits of various structure and appearance occur in the dentinal tubules under various clinical conditions. Sometimes these deposits have the appearance of peritubular dentin, and have generally been regarded as resulting from a continuous formation of the peritubular dentin (Fig. 1.6). This is probably a misconception since peritubular dentin is a developmental and not an acquired structure and since it forms in full thickness concomitantly with the intertubular dentin. Toward the lumen of the tubule, the peritubular dentin appears to be lined by an organic sheath which has been termed the lamina limitans (Fig. 1.6). A proper term for tubular deposits inside the lamina limitans would therefore be intratubular dentin.
Fig. 1.3 Localized secondary dentin formation as a result of external irritation (hematoxylin-eosin).
a Secondary dentin (SD) with regular tubular structure indicating mild stimulation of odontoblasts.
b Secondary dentin with an atubular, cell-containing zone at the dentin–secondary dentin interface due to severe disturbance of odontoblast function. The cells have recovered and have continued to lay down tubular dentin.
c Secondary dentin with few tubules due to death of most odontoblastic cells.
Fig. 1.4 Scanning electron micrograph of a tubule from circumpulpal dentin in a 21-year-old. In addition to the odontoblastic process, a slim fiber which divides into two branches and which is interpreted as a nerve fiber is present (× 9000).
Fig. 1.5 Scanning electron micrograph of a tubule from the coronal dentin of a 42-year-old. A fiber consisting of fibrils with the cross-banding typical of collagen visible on their surface is present in the tubule (× 10 000).
Age Changes
Both macroscopic and microscopic changes occur in the dentin with increasing age, and both types of changes are of considerable clinical importance.
The macroscopic age changes are characterized by the lifelong formation of physiologic secondary dentin which continually modifies the size and to some extent the shape of the pulp chamber and the root canal. At first these changes are beneficial in that they give the root canal a size and form that enhances the possibilities for successful endodontic treatment. However, in old age, the root canals may be obliterated to such an extent that necessary endodontic treatment becomes extremely difficult. Physiologic secondary dentin formation also occurs on the walls of lateral and accessory root canals, often causing a complete occlusion of these narrow spaces. This is the reason why accessory canals, for instance, in the furcation area of molar teeth which may be readily demonstrable in young teeth are evident only in rare instances during endodontic treatment of adult patients.
Fig. 1.6
a Electron micrograph of a cross-cut tubule from partly demineralized root dentin. An electron-dense line (lamina limitans) is recognized between the peritubular dentin matrix and the material beginning to occlude the tubule (×20 000).
b Crosscut tubule from undemineralized dentin occluded with material (intratubular dentin) with electron density different from that of peritubular dentin (PD) (×19 000).
The microscopic age changes of the dentin are characterized by the fact that an increasing number of dentinal tubules become obliterated by mineralized tissue. The occluding material is homogenous and consists of a noncollagenous matrix and small hydroxyapatite crystals. Its appearance is similar to that of peritubular dentin, but as a rule it can be distinguished from this tissue by a difference in density or by the presence of the lamina limitans (Fig. 1.6). From a clinical point of view, it is important to know that the formation of the age-related intratubular dentin starts at the apex of the tooth and continues in a coronal direction with increasing age. In the coronal dentin, the intratubular mineralization will not lead to a complete obliteration of the tubules until the patient is in his 70 s. The process is so closely related to age that the coronal extent of the tubular occlusion is used in forensic dentistry for age determination purposes.
Thus, the microscopic dentinal changes that occur as a result of aging render the root of the tooth homogenous with few patent dentinal tubules. Conceivably, this may facilitate endodontic treatment of nonvital teeth where dentinal tubule infection is a definite problem. The tubules of the coronal dentin, on the other hand, will not be significantly affected by the aging process until the patient is old.
The Dental Pulp
Morphology
The dental pulp consists of a richly vascularized and highly innervated connective tissue (Fig. 1.7). It is surrounded by dentin and has a form that mimics the outer contour of the various teeth (Fig. 1.8). The pulp tissue is in communication with the periodontium and the rest of the body through the apical foramen and accessory canals near the apex of the root (Fig. 1.9). Accessory canals are also found laterally in the root and in the furcation area of molar teeth. However, from a practical–clinical point of view, the pulp is an end organ without collateral circulation.
Cells, Fibers, and Ground Substance
The most characteristic element of the dental pulp is the dentin-forming cell, the odontoblast. The odontoblasts are tightly packed, regularly aligned, polarized cells located at the periphery of the pulp with cytoplasmic processes extending into the tubules of the predentin and dentin. This continuous sheet of odontoblasts at the pulp periphery has been termed the odontoblastic layer (Fig. 1.10). Ultrastructurally the odontoblasts are shown to be similar to other connective tissue cells and their identity is mainly determined by their location. The odontoblasts are static post-mitotic cells, apparently incapable of further cell division. Also, the rate of repopulation of the odontoblast layer is extremely slow under physiological conditions. Probably most and possibly all odontoblasts seen in teeth in older individuals are the original cells. The cell bodies of the odontoblasts are united in certain areas by cell-to-cell junctions. This may allow the odontoblasts to function as a syncytium, a continuous layer of cells.
Fig. 1.7 Coronal pulp in the incisor tooth of a monkey. The odontoblast layer bordering the lightly stained predentin as well as cell-free and cell-rich subodontoblastic zones can be recognized (hematoxylin-eosin).
Fig. 1.8 Overview of the tooth with enamel (E), dentin (D), coronal pulp (CP), and root pulp (RP). Note that the pulpal cavity has a shape which mimics the outer contour of the tooth.
Fig. 1.9 Apical part of the root of a human incisor (R) with pulp (P), periodontal ligament (PDL), and alveolar bone (AB). Note that the apical foramen (arrow) is located laterally to the anatomical apex of the tooth (hematoxylin-eosin).
Fig. 1.10 Dentin–pulp interface in a monkey incisor. Adjacent to the dentin (D), the unmineralized (and unstained) predentin, the odontoblast layer, the cell-free zone, and the cell-rich zone can be seen (hematoxylin-eosin).
Another trait of pulp identity is the subodontoblastic region. In the coronal pulp this region is characterized by a cell-free zone and a cell-rich zone beneath the odontoblastic layer (Figs. 1.7, 1.10). The cells of the subodontoblastic region differ from the odontoblasts in that they have a bipolar, sometimes multipolar arrangement. Structurally, the cells resemble fibroblasts of the central pulp, and like the odontoblasts, they are identified by their location. It has been speculated that the subodontoblastic cells have specific functions, for instance, that they are “preodontoblasts” capable of proliferation and differentiation into new odontoblasts. This theory and others have not been substantiated. However, it is known that they are involved in the elaboration of collagen and ground substance like the cells of the rest of the pulp.
In the bulk of the pulp tissue, three main types of cells are seen: inactive mesenchymal cells, fibroblasts, and fibrocytes. The mesenchymal cells are thought to be multipotential in that when they are stimulated and undergo cell division, their daughter cells may develop into any of the mature connective tissue cells, including odontoblasts. The fibroblasts are the most numerous cells in the pulp and are responsible for ground substance and collagen production, collagen degradation, and turnover. The fibrocytes possibly play a role in the maintenance of collagen fibers.
As will be discussed in some detail later, the pulp has cells that under certain circumstances can develop into hard tissue–producing cells. Thus, after pulp capping, the dentin bridge is formed by new odontoblasts (see Fig. 5.11). Cementum- and bone-like tissues as well as more structureless hard tissues may form in the pulp as well. However, it is not quite clear at present which of the cells in the pulp are capable of differentiation into hard tissue-producing cells.
Other stable cellular elements in the pulp either belong to the vascular or the neural system. In addition, inflammatory cells such as lymphocytes, plasma cells, and macrophages are occasionally seen. Mast cells appear to be a rare occurrence in the healthy pulp.
The ground substance of the pulp has a mucoid consistency. It serves as a matrix in which cells, fibers, and blood vessels are embedded. It is organized as a heterogenous colloid with soluble and insoluble components. The main molecular components are proteoglycans which consist of a glycosaminoglycan linked to a protein molecule. Their major functions have been recognized to be the protection of the cellular elements and capillaries of the pulp, their interaction with collagen to form aggregates possibly involved in dentin matrix formation, as well as control or inhibition of mineralization.
The pulp has two main types of fibers, collagen fibers and elastic fibers, the latter always being confined to the walls of larger blood vessels. Thus, the fibers of the intercellular matrix of the pulp are collagenous in nature. The fibers of the young pulp are small and far from numerous. They are distributed diffusely within the tissue and are often covered by a glycosaminoglycan sheath. In the mature pulp, larger fiber bundles can be seen as well, especially along blood vessels in the root pulp. These fibers are usually devoid of a glycosaminoglycan sheath.
Vascular Supply
Blood vessels the size of arterioles branch off the dental artery and enter the pulp through the apical foramen and possibly through accessory canals (Fig. 1.11). Inside the pulp the main arterioles are seen in a central location extending to the coronal pulp. They give off branches that spread in the tissue, diminish in size, and finally become capillaries. An extensive capillary network is formed in the subodontoblast and odontoblast areas of the pulp. The capillaries provide the odontoblasts and other cells of the pulp with an adequate supply of nutrients. The blood then passes from the capillaries to postcapillary venules and to gradually larger venules toward the central region of the pulp where they are seen alongside the arterioles. Two to three venules leave the pulp through the apical foramen and possibly through accessory canals. Outside the tooth the pulp venules join with vessels that drain the periodontal ligament and alveolar bone.
Multiple arteriovenous anastomoses exist in the pulp. These direct connections between arterioles and venules make it possible for the circulating blood to bypass the capillary plexus. They play an important role in the regulation of pulpal blood flow.
Fig. 1.11 Microangiograph showing vasculature in the pulp of a dog incisor. The main vessels are located centrally in the pulp and an extensive capillary network is seen, especially in the subodontoblast and odontoblast regions.
The vascular pattern described above is basically found in all single-rooted teeth and in each root of multirooted teeth. Thus, in multirooted teeth, an alternate blood supply is generally available, resulting in extensive anastomoses in the coronal pulp. However, the main venous drainage sometimes occurs through one root in these teeth. Blood vessels which communicate with the pulp through accessory or lateral canals do not contribute significantly as a source of collateral circulation, except possibly in the apical 1–2mm of the root canal.
The existence of lymphatic vessels in the pulp is a matter of dispute due to limitations in available investigative techniques. Recent ultrastructural studies suggest that lymphatic capillaries arise in the peripheral areas of the pulp and join other lymph vessels to form collecting channels that leave the pulp through the apical foramen. Moreover, there is evidence of anastomoses between lymphatics from the pulp, the periodontal ligament, and the alveolar bone in the periapical area.
Innervation
The innervation of the pulp comprises afferent nerves which conduct sensory impulses (A-fibers) and autonomic nerves (C-fibers) that are mainly involved in neurogenic modulation of the blood flow but also in transmission of pain (see p. 66). The A-fibers are of the trigeminal system. They are myelinated, surrounded by Schwann cells, and enter the pulp in bundles with the blood vessels through the apical foramen. The C-fibers constitute the majority of the pulpal nerves. They are unmyelinated and enclosed singly or in groups by Schwann cells, and enter the pulp with the sensory fibers. Some branching of the nerves occurs in the root pulp and the branching becomes extensive in the coronal pulp. Beneath the cell-rich zone is the plexus of Raschkow, which consists of a large number of both myelinated and unmyelinated nerve axons. From this plexus some sensory nerves without their myelin sheath but still inside their Schwann cells approach the odontoblastic layer. Near the odontoblasts, terminal axons leave their Schwann cells and pass between the odontoblasts to the predentin, and in some instances enter the dentinal tubules, where they end in close proximity of the odontoblastic process (Fig. 1.5). Nerve endings may also be trapped in the mineralized dentin between the tubules. However, most of the sensory nerves end in the odontoblastic layer or they pass between the odontoblasts and return to the area of Raschkow’s plexus.
The physiological need for the vast number of nerves in the periphery of the coronal pulp is not immediately understood. In all likelihood they have proprioceptive functions. Thus, it has been shown that patients readily bite three times as hard on pulpless teeth as on teeth with intact pulps.
Age Changes
As discussed above, continued formation of physiologic secondary dentin over the years will lead to a reduction in the size of the pulp chamber and the root canal. Certain changes which occur in the pulp tissue can be related to the aging process as well. A striking observation is a reduced or missing odontoblastic layer in teeth from older individuals (Fig. 1.12). Fibrosis of the pulp is commonly seen and a reduced number of cells is present between the fiber bundles. Arteriosclerotic changes may occur in the pulp vessels, and capillaries and precapillaries commonly calcify. Nerve endings regularly calcify as well. Intrapulpal mineralizations increase with age and especially diffuse calcifications along the vessels of the root pulp are considered to be an age-related phenomenon (Fig. 1.13).
Fig. 1.12
a Coronal pulp (P) from the incisor of a 71-year-old. Large amounts of secondary dentin (SD) have formed. Almost no odontoblasts are present and the pulp is generally poor in cells (hematoxylin-eosin).
b Root pulp poor in cells and rich in fibers (van Gieson stain).
Fig. 1.13
a Diffuse calcifications in root pulp (van Gieson stain).
b Cellular (cementum-like) hard tissue (H) in the root canal near the apical foramen (AF) (hematoxylineosin).
Age reduces the functional capacities of a tissue. It is conceivable, therefore, that the age of a patient may affect unfavorably the outcome of certain endodontic procedures like pulp capping and pulpotomy.
Reaction Patterns
Pulpitis
Like other connective tissues in the body, the pulp reacts to irritants with inflammation (Fig. 1.14). However, the pulp has certain characteristics that make it unique and that may alter this tissue response—sometimes dramatically. Tumor (swelling) is one of the cardinal signs of inflammation. When the pulp becomes inflamed, anatomical limitations, that is, the hard root-canal walls, will preclude an increase in tissue volume. In addition, the pulp almost completely lacks a collateral circulation. These two factors place the pulp at a considerable disadvantage in dealing with edema, necrotic tissue, and foreign material. On the other hand, the pulp is the only connective tissue that has the ability, at least to a certain extent, to protect itself from external irritants by the formation of intratubular and secondary dentin.
In the classic view of pulpal inflammation, immense importance was given to the influence of the anatomical environment on the pulp. It was assumed that with a local inflammation in the pulp there would be an increased blood flow to the inflamed area. The vasodilation and increased capillary pressure and permeability induced by the inflammation would result in an increased filtration from the capillaries into the tissue, which in turn would cause steadily increasing tissue pressure (Fig. 1.15). Gradually, as the pressure outside the vessels rose, the thin-walled vessels were compressed. This would lead to a decrease in blood flow as well as an increase in venous pressure, which in turn would result in increased capillary pressure and a further increase in the filtration from the capillaries to the tissue. Thus, a vicious circle seemed to develop, resulting in a steady increase in tissue pressure. Consequently, since the pulp lacks the possibility of expansion, the final result of the increase in tissue pressure was then thought to be a choking or strangulation of the pulpal vessels at the apical foramen, leading to a stagnation of the blood circulation with resultant ischemia and necrosis.
Fig. 1.14 Local inflammatory responses to external irritation in the pulp of monkey teeth (hematoxylin-eosin).
a Mild inflammation with filled capillaries in the odontoblast layer and few inflammatory cells.
b Moderate inflammation with accumulation of inflammatory cells in the affected area of the pulp.
c Severe inflammation with abscess formation.
However, modern research has not supported the strangulation theory. For example, in experiments where the tissue pressure of the pulp is measured, it has been found that a pressure increase in one area of the pulp does not cause a pressure increase in the rest of the pulp. It has also been shown that when local inflammation is induced in the pulp, the tissue pressure increases only in the inflamed area and not in the entire pulp cavity. Experiments with in vivo microscopy have confirmed that an injury in the coronal pulp results in local circulatory disturbances and, if the injury is severe enough, to complete stasis in the vessels in and near the injured area. The circulation in the root pulp, by contrast, is unaffected in these experiments. Experimental studies on the healing of pulpal inflammation have confirmed these findings (Fig. 1.16), and presently there is convincing evidence that even severe inflammatory changes in a limited area of the pulp do not result in a circulatory stoppage in the entire pulp.
Based on these and other studies, a modern theory on the hemodynamics of pulpitis has developed. The pulp normally has a relatively high blood flow which is/>
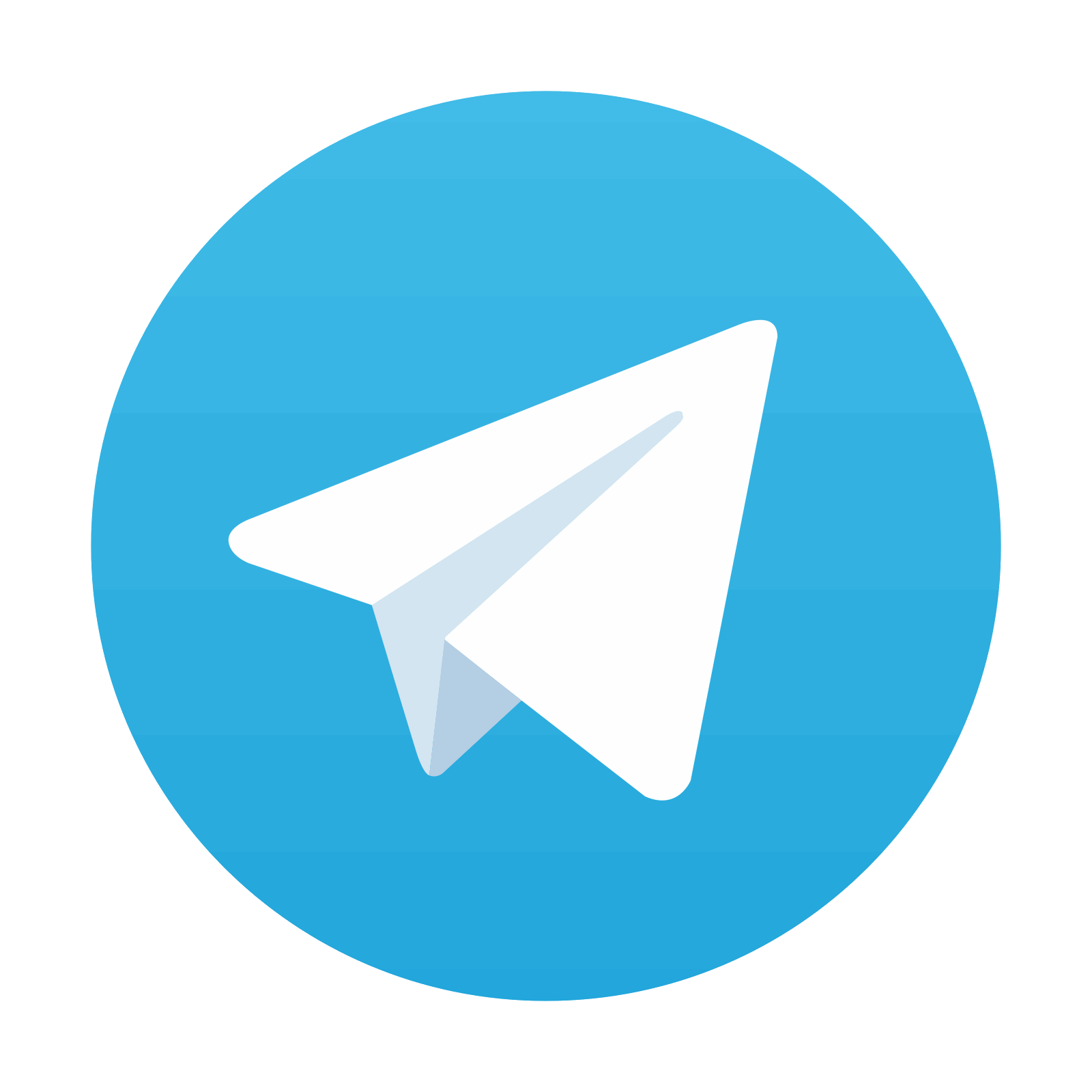
Stay updated, free dental videos. Join our Telegram channel

VIDEdental - Online dental courses
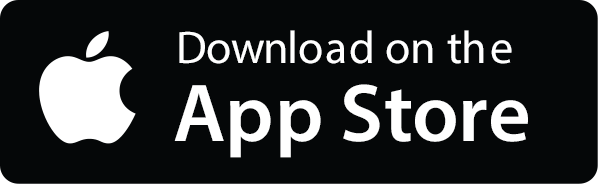
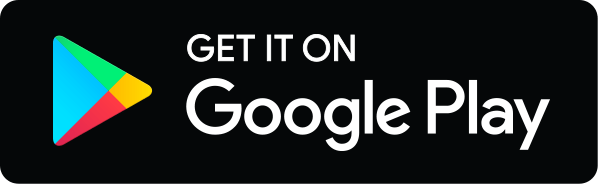