Chapter 5
Dentin, Pulp, and Tooth Pain1
Michel Goldberg
Biomédicale des Saints Pères, Université Paris Descartes, INSERM UMR-S 1124
1 With a contribution by Bradley K. Formaker, Ph.D., University of Connecticut School of Dental Medicine, Farmington, CT.
Dentin forms the bulk of the tooth. It supports the enamel which covers the crown and the cementum that constitutes the superficial layer of the root of the tooth (Fig 5.1). Although dentin is a mineralized tissue, it contains less mineral and is more resilient than enamel, providing resistance to fractures and cracks. Dentin is a vital tissue, containing cytoplasmic processes of the cells that produce it, odontoblasts, and nerve endings (Figs. 5.2 and 5.3). Dentin encloses the pulp, which has an abundant blood and nerve supply, as well as immunologic and regenerative properties. The pulp maintains the vitality of the dentin and the odontoblasts. Dentin, as enamel, is susceptible to dental caries, may develop sensitivity when exposed, and is affected by several hereditary diseases.
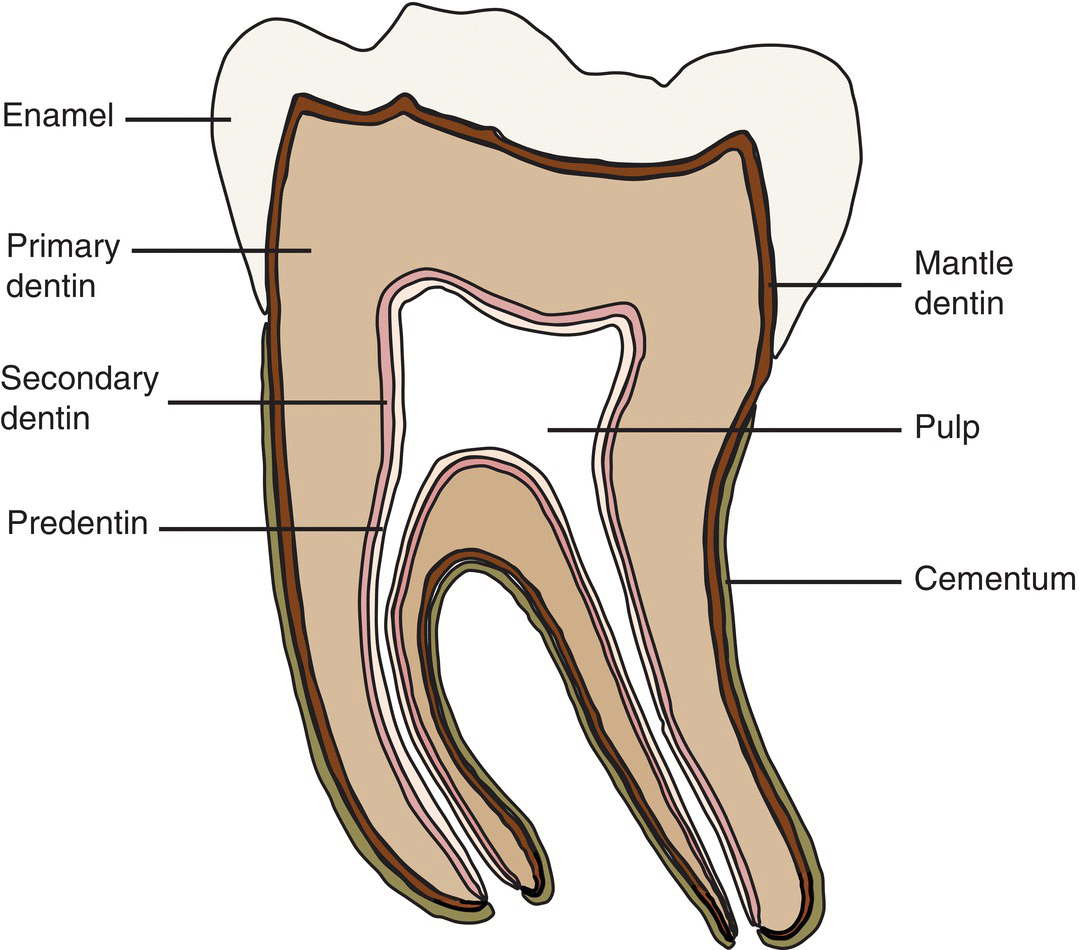
Figure 5.1 General organization of a tooth. Beneath the coronal enamel, the different layers of dentin are found. Mantle dentin is the first dentin deposited; circumpulpal or primary dentin constitutes the bulk of the tooth. Secondary dentin is deposited at a slow rate after primary dentin formation is complete. A layer of predentin separates the dentin from the pulp. The root is covered by cementum.
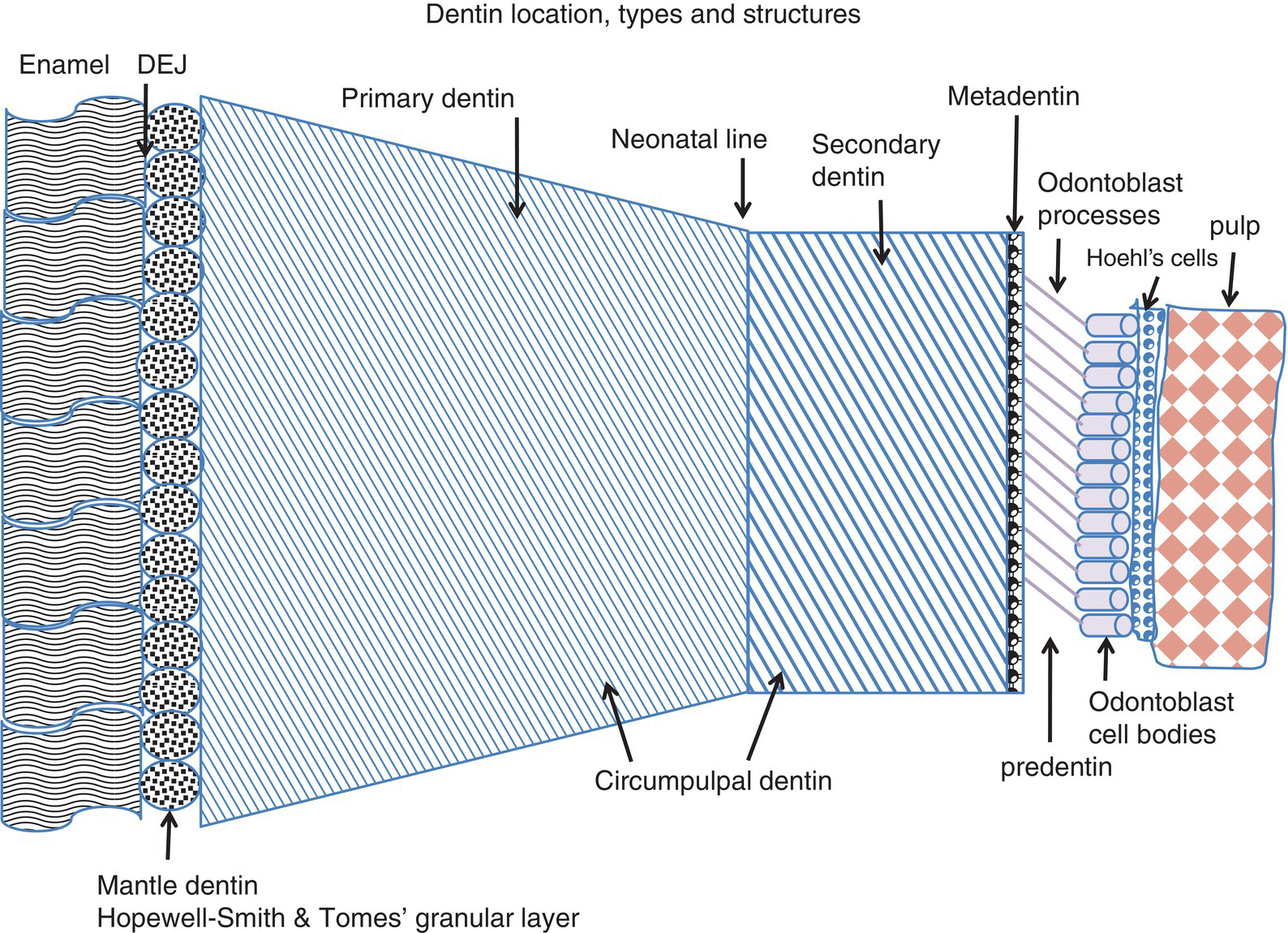
Figure 5.2 Schematic distribution of the different dentin layers located between the dentinoenamel junction (DEJ) and the dental pulp. Two atubular peripheral layers are located beneath the enamel or cementum: the mantle dentin in the crown and the peripheral layers in the root, including the atubular hyaline Hopewell-Smith layer and/or the Tomes’ granular layer. Primary dentin includes the atubular peripheral layers and the tubular circumpulpal dentin of the crown and root. The neonatal line separates dentin formed before birth from dentin formed after birth. Secondary dentin is formed continuously after the completion of primary dentin formation. The mineralization front, or metadentin, is the border where dentin mineralization is initiated. Odontoblast processes cross the predentin (15-20 μm in width) and enter the dentinal tubules. Hoehl’s cells may differentiate into odontoblasts, the dental pulp being located beneath.
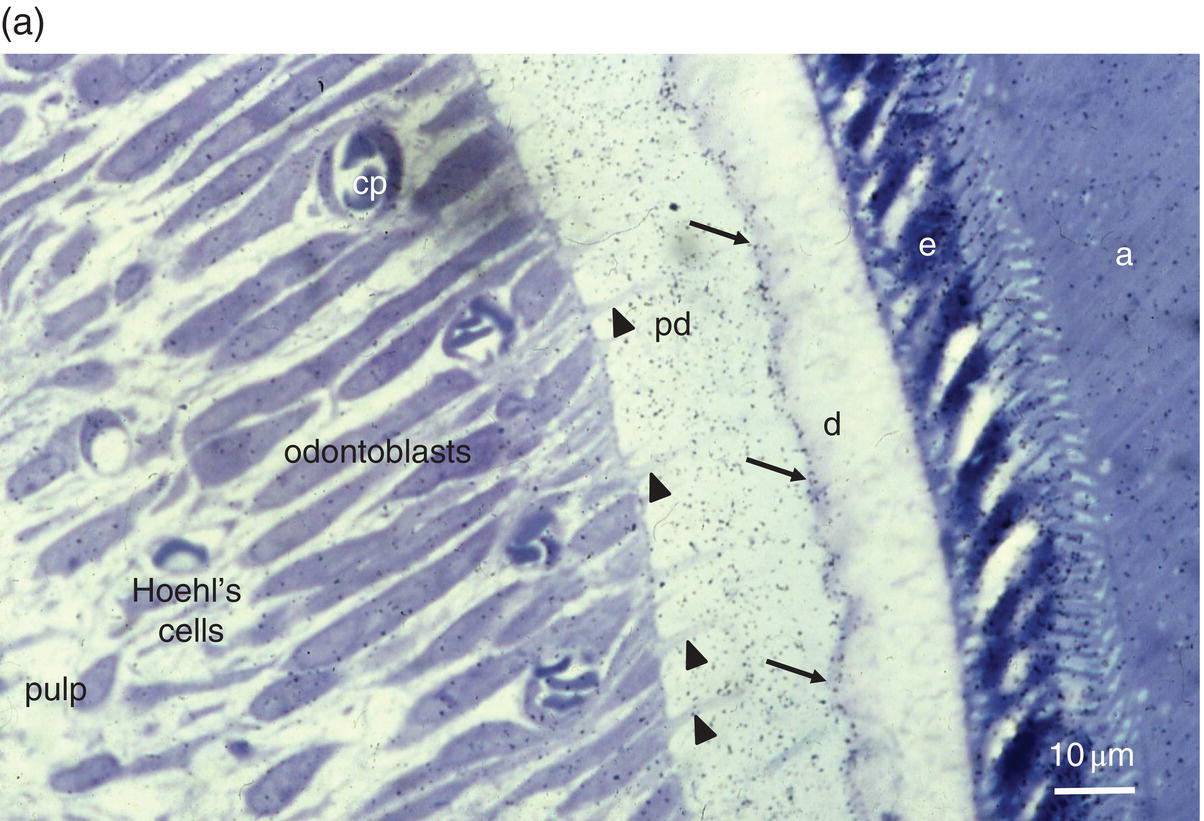
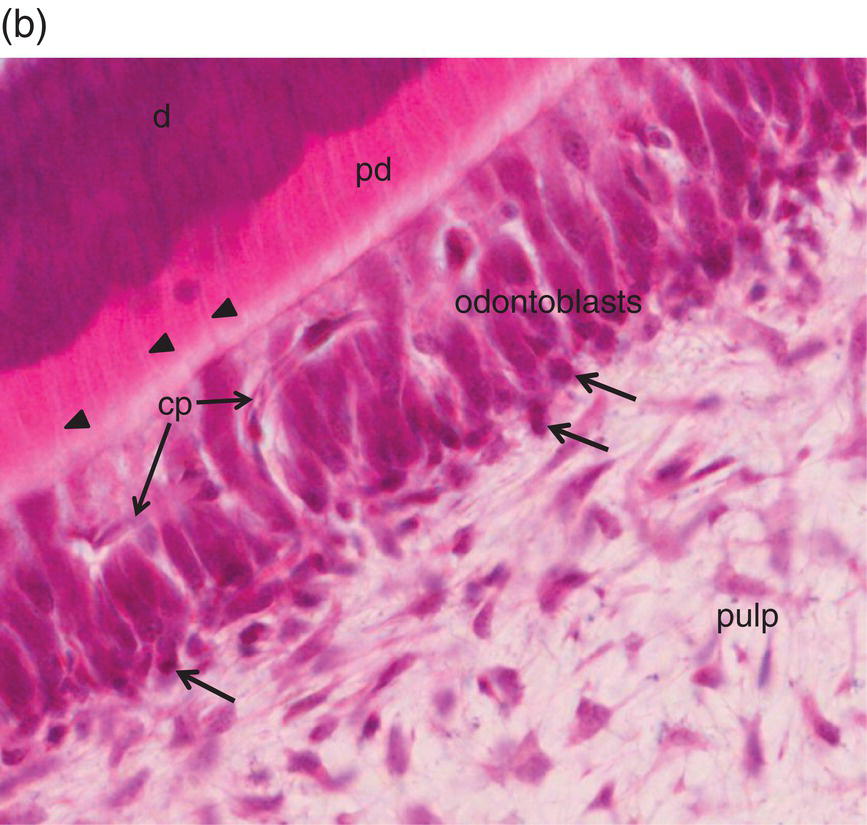
Figure 5.3 Light micrographs of odontoblasts and forming dentin. (a) One-μm-thick section of a rat incisor. On the right, enamel (e) is formed by secretory ameloblasts (a) The mineralization front (arrows) located at the edge of the dentin layer (d) is covered by silver grains (radioautography using (3H) serine as phosphoprotein precursor). Odontoblast processes (arrowheads) extend through the predentin (pd). Dentin extracellular matrix molecules are synthesized in odontoblast cell bodies. Hoehl’s cells may differentiate into odontoblasts. The odontoblast and Hoehl’s cell layers include endothelial cells forming capillaries (cp). The dental pulp is located more centrally. Bar = 10 μm. (b) Hematoxylin- and eosin-stained section of a human molar. Labels as in 5.3(a). Arrowheads indicate odontoblast processes, and arrows indicate Hoehl’s cells.
Evolution of dental structures
From an evolutionary point of view, scales covering the whole body were gradually restricted to the oral cavity. A mineralized bony structure appeared in the so-called odontodes, the primitive teeth of early vertebrates. At later stages of evolution, the formative cells polarized and developed long processes within the mineralized part, which was gradually transformed into osteodentin, a bone-like structure that implies that cells similar to osteocytes are located within lacunae. The cell bodies moved out from the mineralized structure, polarized, and contributed to the formation of a continuous layer at the surface of the pulp. The next step of evolution was the formation of a true orthodentin, with the cell bodies outside the mineralized dentin and long processes extending within dentinal tubules.
Dentin structure and composition
Different types of dentin are present in normal and pathologic teeth (Fig. 5.2 and Tables 5.1 and 5.2).
Table 5.1 Schematic distribution of the successive layers and types of sound dentin in longitudinal section between the dentinoenamel (DEJ) and the pulp
Location | Dentin Type | Structure |
Peripheral dentin | Crown:
|
Atubular dentin, 30–150 µm thick. Initial dentin formed in the crown. Non-phosphorylated proteins. Resilient border. |
Root:
|
Initial atubular dentin. Calcospherites or globular structures and interglobular spaces. A few bent minute tubules. |
|
Circumpulpal dentin | Primary dentin | The dentin formed between the creation of initial peripheral dentin and early tooth functioning. The tubules display a longitudinal S-shaped curvature. The formation of primary dentin is limited by the outer DEJ and the initiation of secondary dentin deposition. |
Secondary dentin | Formed normally and continuously after completion of the crown and root. Results from the daily 4-µm dentin formation. The S-shape of the tubules is more accentuated, and the tubules are less numerous, due to space restriction. | |
Peripheral dentin | Atubular dentin |
|
Circumpulpal dentin | Tubular dentin | Intertubular dentin: a collagen-rich dentin associated with non-collagenous proteins along and between the collagen fibrils. The hydroxyapatite crystallites are 3–5 nm thick and 60 nm long. |
Peritubular dentin: 25–30 nm crystallites, and an amorphous network without collagen fibrils lining the lumina of dental tubules. |
Table 5.2 Pathologic dentin
Causative Event | Response | Structural Alteration |
Genetic alterations:
|
Gene deletion of collagen I, or phosphorylated proteins (SIBLINGs) (DSPP, DSP, DMP1) | Abnormal dentin properties and tooth structure |
Environmental effects:
|
Odontoblast gene expression changes | Hyper/hypomineralization, structural alterations |
Loss of tooth structure:
|
Reactionary dentin | Ortho- or osteodentin formed beneath a calciotraumatic line |
Pulp exposure | Reparative dentin | Dentinal bridge, partial or total pulp mineralization |
Outer layers
In the coronal part of the teeth, beneath the enamel and under the dentinoenamel junction (DEJ), the mantle dentin, 30 to 150 μm thick, displays resilient properties. This is the first layer of dentin deposited, and it is less mineralized than the circumpulpal dentin. Lower calcium phosphate content is found, and the phosphorylated non-collagenous proteins implicated in mineralization are reduced (Fig. 5.4a).
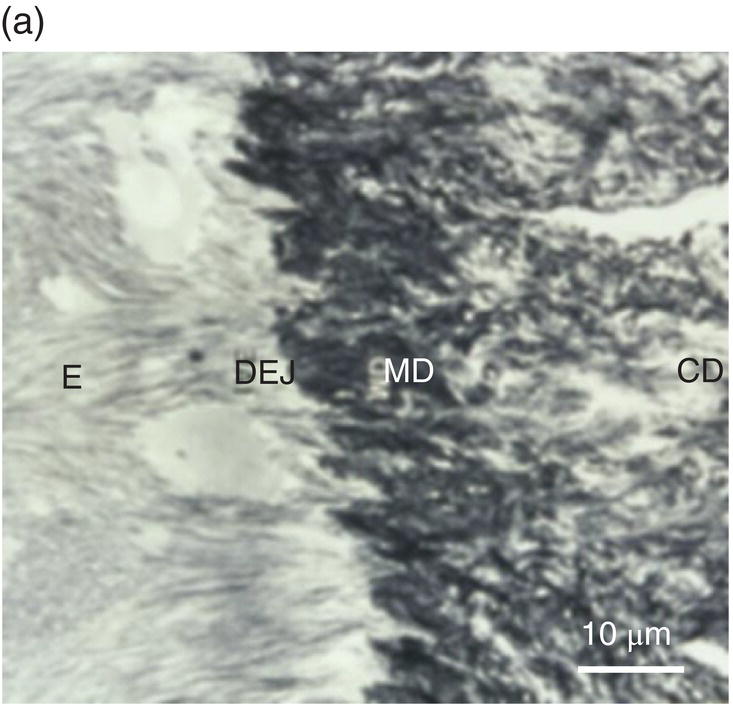
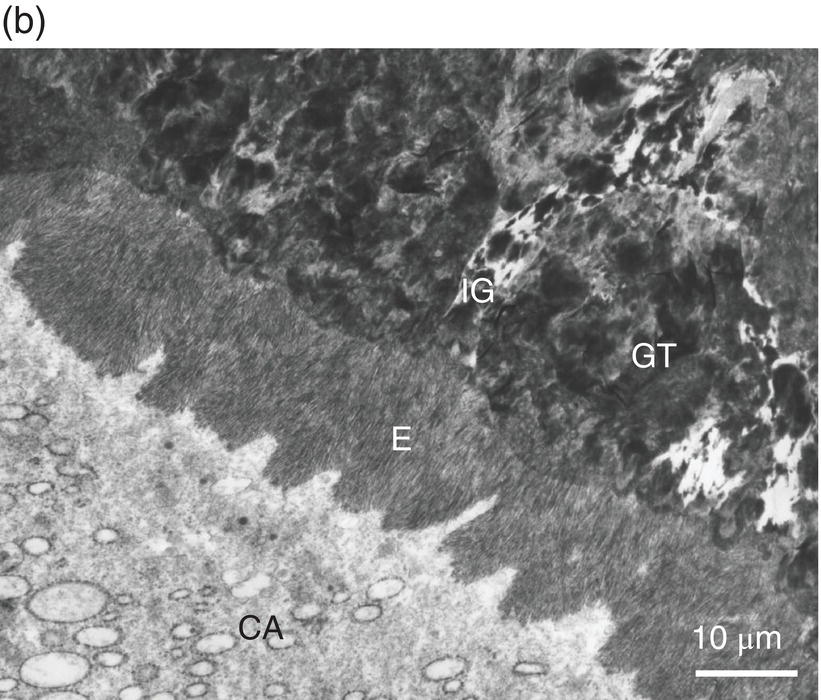
Figure 5.4 Electron micrographs of a rat molar. (a) Atubular mantle dentin (MD) in the coronal part of the forming molar. DEJ: dentinoenamel junction. E: enamel. CD: circumpulpal dentin. (b) Beneath the forming enamel (E) and cervical ameloblasts (CA), calcospherites contribute to the formation of the superficial Tomes’ granular layer (GT). An interglobular space (IG) is located between the granules. Bar = 10 μm.
In the root, beneath the cementum and along the dentin-cementum junction, two different dentinal structures have been identified, with some variations related to species differences: the hyaline Hopewell–Smith zone appearing as a thin border (8–15 μm thick), and Tomes’ granular layer (8–15 μm in width) (Fig. 5.4b). The two peripheral layers contain a few bent, minute dentinal tubules. Globular structures (calcospherites) are grouped in these outer layers, which possibly result from the increased size of matrix vesicles. Calcospherites merge more or less homogeneously, but between the mineralized globules, defective large interglobular spaces are not mineralized. This is reminiscent of what is seen in X-linked hypophosphatemia teeth (hypophosphatemic rickets) where globular structures are seen, isolated by large interglobular spaces containing non-collagenous proteins and proteoglycans (Table 5.2).
Circumpulpal dentin
The bulk of dentin is constituted of circumpulpal dentin exclusively produced by the odontoblasts (Fig. 5.5a). Odontoblasts initially form predentin, which undergoes mineralization and becomes dentin. The daily production of dentin decreases from 10 μm/day at the onset of dentin formation to 4 μm/day. This generates a rhythmic formation, and at each 4 μm a daily incremental (von Ebner’s) line appears. This circadian rhythm is interacting with another longer-period rhythm forming Owen’s lines, appearing each 16–20 μm, due to unknown mechanisms. The continual centripetal formation of primary dentin results in gradual reduction of the pulp volume (Figs. 5.2 and 5.3).
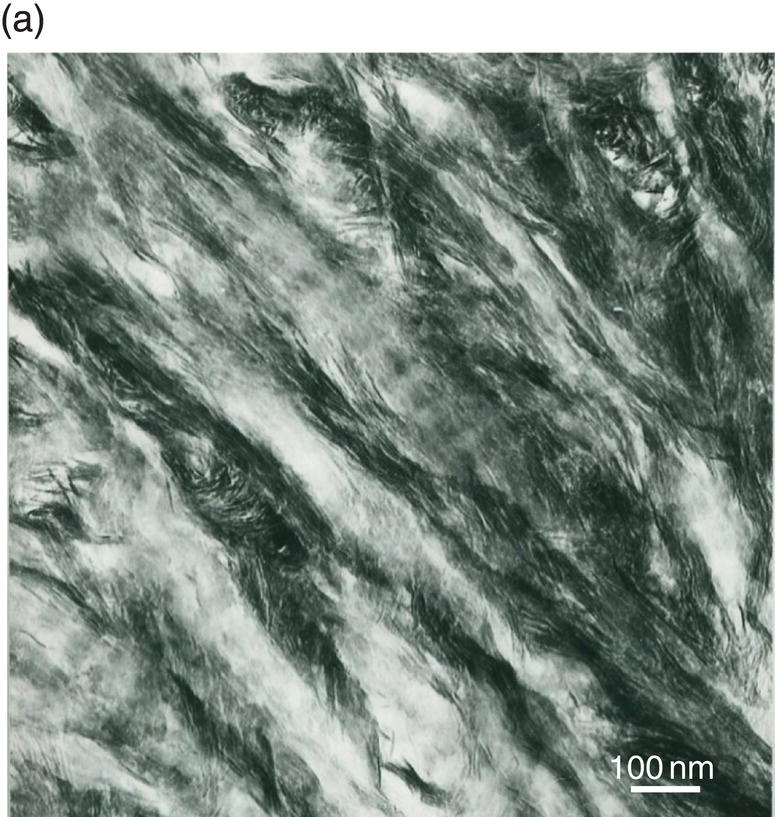
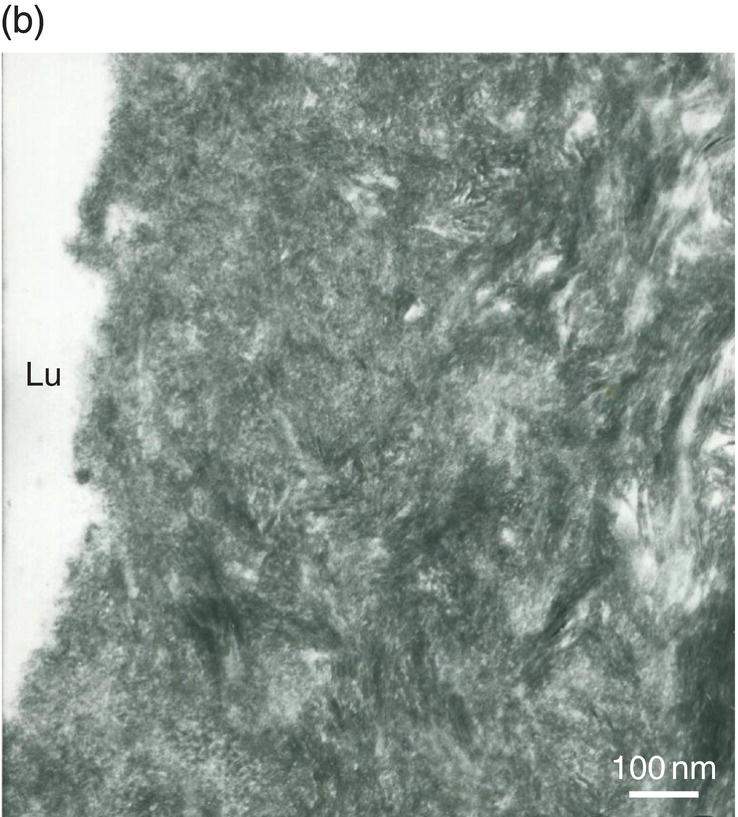
Figure 5.5 Electron micrographs of unstained ultrathin sections of human dentin. (a) Intertubular dentin. Crystallites are located along and between collagen fibrils. The periodic banding of collagen is apparent. Bar = 100 nm. (b) Peritubular dentin displays isodiametric crystallites about 35 nm in diameter. Lumen of dentinal tubule (Lu). Bar = 100 nm.
As each odontoblast deposits dentin matrix and retreats toward the central pulp, it extends an elongated process from the distal end of the cell body. Continual deposition and mineralization of dentin matrix around the odontoblast process creates a tubule within the dentin, from near the dentinoenamel junction to the pulpal surface of the dentin. Dentin contains about 20,000 dentinal tubules per mm2, with variations between the outer and inner parts of the dentin layer. Due to space restriction, more tubules are present in the inner third than in the outer third (between 18,000 and 21,000/mm2). The tubules are curved, displaying a gradual S shape from the dentinoenamel junction to the pulp. The primary dentin of the crown and most of the root is formed before eruption. The curvature is more accentuated in tubules formed after the eruption of the tooth.
Secondary dentin formation begins after completion of primary dentin formation. The structure and composition of secondary dentin are similar to those of primary dentin. A slight change in the direction of the dentinal tubules occurs when secondary dentin formation begins. The formation of secondary dentin continues at a slow rate throughout life, but is gradually reduced in later years (Fig. 5.2 and Table 5.1).
Between the tubules, intertubular dentin is found (Figs. 5.5a, 5.6 and Table 5.1). This dentin specifically results from the continuous apposition of predentin components at the mineralization front (Fig. 5.3). It is a collagen-rich structure, containing fibrils about 80 to 100 nm in diameter (Fig. 5.6). Non-collagenous proteins are associated either with the surface of collagen fibrils and holes due to the quarter-stagger transversal bands of collagen fibrils, or they fill the spaces between collagen fibrils (Fig. 5.7). The dentin organic matrix plays a crucial role in promoting crystal nucleation and allowing limited growth to hydroxyapatite crystallites. The needle-like crystallites are 3 nm in thickness and 60 nm in length. Each crystallite is formed by the association of two plates (each 1 nm thick) separated by a thin electron-lucent layer (Fig. 5.8a).
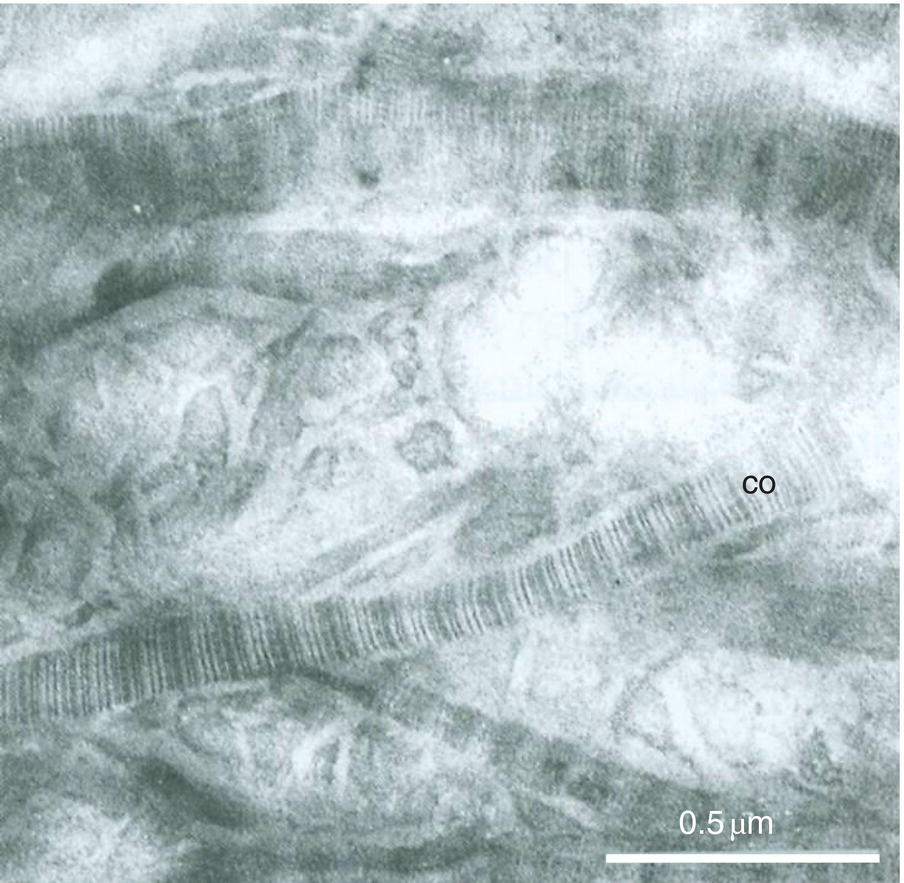
Figure 5.6 Electron micrograph of type I collagen fibrils (co) in intertubular dentin. Demineralized section. Bar = 0.5 μm.
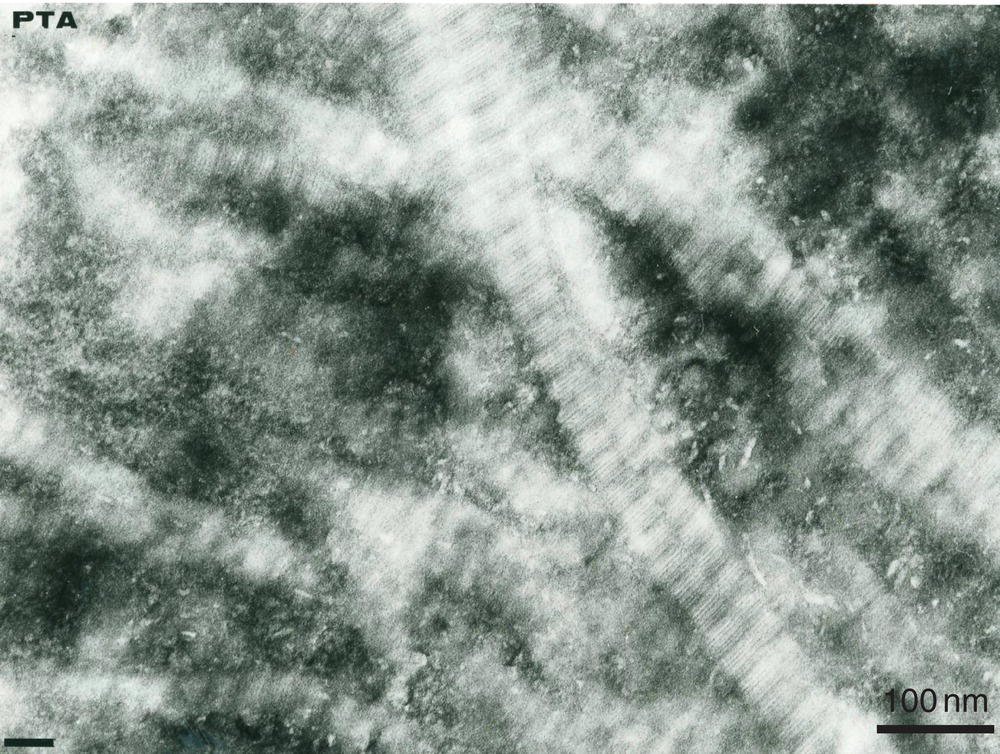
Figure 5.7 Electron micrograph of phosphorylated extracellular matrix proteins stained with phosphotungstic acid–chromic acid (PTA) in intertubular dentin. The electron-dense staining is located along and between the collagen fibrils. Bar = 100 nm.
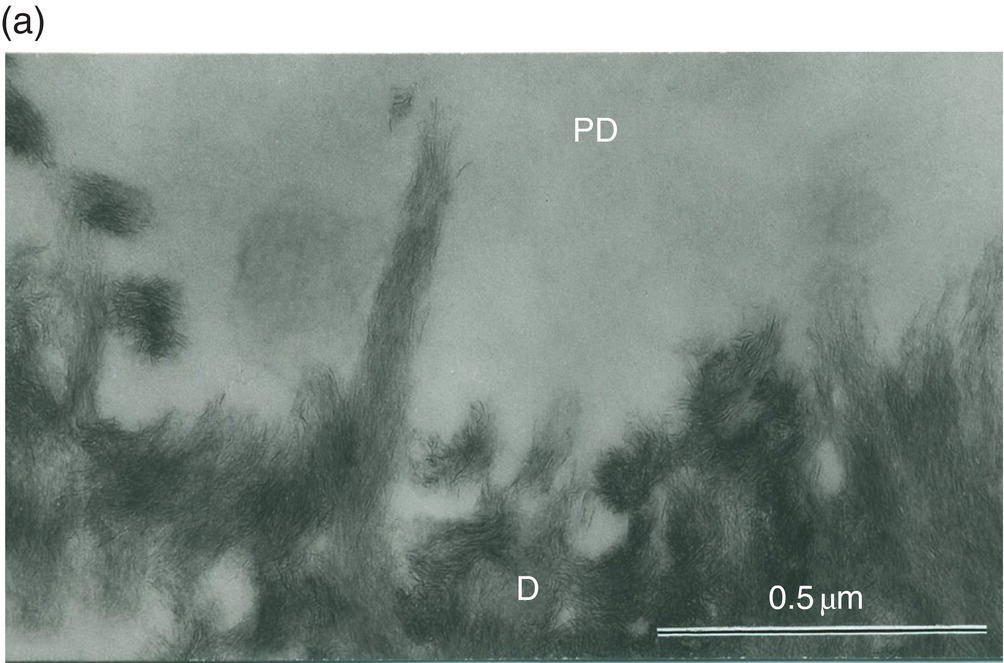
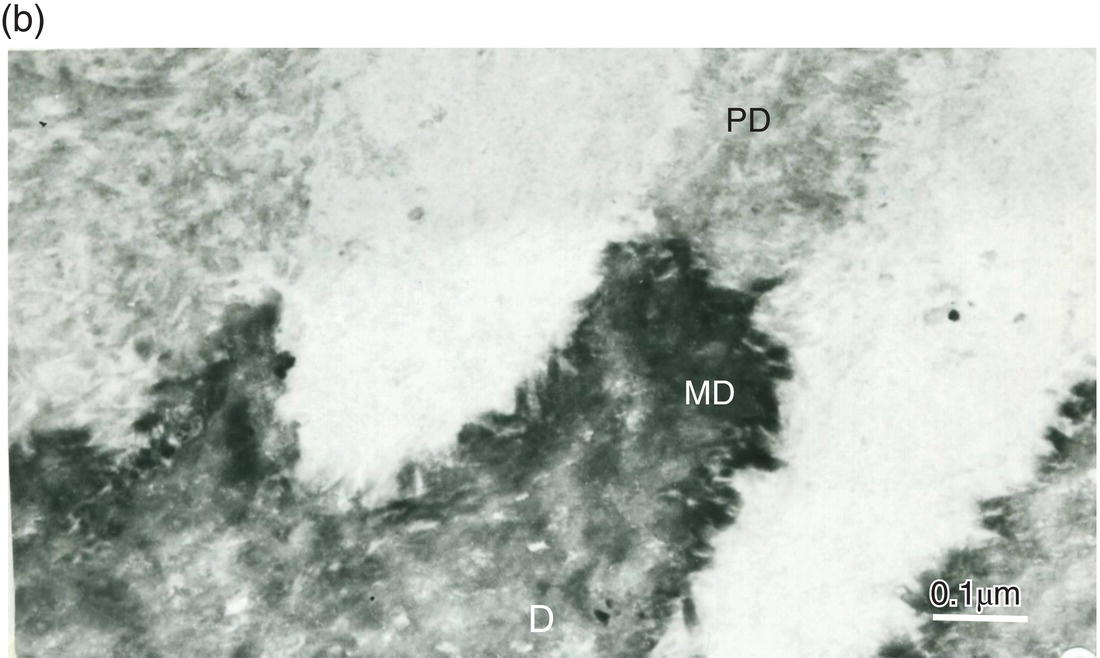
Figure 5.8 Electron micrographs of rat molar dentin. (a) Unstained section. Needle-like crystallites located at the mineralization front between the predentin (PD) and dentin (D) are seen along the collagen fibrils. Bar = 0.5 μm. (b) The distal border (metadentin: MD) located between the predentin (PD) and intertubular dentin (D) is densely stained by the phosphotungstic acid–chromic acid mixture. Bar = 0.1 μm.
The lumen of the dentinal tubules contains non-mineralized collagen fibrils and some amorphous proteins forming a periodontoblastic structure around the odontoblast process. Peritubular dentin forms a highly mineralized ring around the tubules but never completely fills the lumen. Isodiametric crystals about 25 nm in width and 9 to 10 nm in length resist abrasive forces and occlusal pressures (Fig. 5.5b and Table 5.1). These crystallites have high magnesium and carbonate content. They display high solubility in acid and chelating solutions. Peritubular dentin formation begins within the tubules, a few micrometers from the predentin-dentin junction. Present mostly in the inner two-thirds of the dentin, it is reduced in the outer one-third and almost lacking in the mantle dentin. Peritubular dentin does not contain collagen fibrils, but is comprised of non-collagenous proteins, namely phospholipids or lipoproteins and proteoglycans (Fig. 5.5b).
In the sclerotic zone of carious decay, or after pathologic abrasion, or as a consequence of the aging process, the tubules may be filled by intratubular mineralization. Such heterogeneous mineralization results from the occurrence of pathologic processes that favor the precipitation of calcium and phosphate ions into non-apatitic forms within the lumen of the tubules.
The reparative process occurs in arrested carious lesions, or in response to the preparation of cavities. It also may be due to the effects of the release of resin monomers by restorative dental materials. These events lead to the formation of tertiary dentin, classified as either reactionary dentin or reparative dentin. Hoehl’s cells (Fig. 5.3) and surviving secretory odontoblasts form reactionary dentin, which appears either as orthodentin or osteodentin (Table 5.2). If both the odontoblastic and Hoehl’s cell layers are irreversibly altered, pulp progenitor cells contribute to the formation of reparative dentin.
Dentin composition
Dentin consists of an organic matrix composed largely of Type I collagen, within which hydroxyapatite mineral crystals are deposited. Mature dentin contains more organic substance than enamel, but less mineral (Table 5.3). In addition to Type I collagen, small amounts of Type III and Type V collagen are present. Non-collagenous components, including phosphorylated and non-phosphorylated proteins, proteoglycans, lipids, growth factors, and enzymes, account for about 10% of the organic matrix (Table 5.4). While many of the non-collagenous components also are present in bone and other tissue, products of the dentin sialophosphoprotein gene are specific to odontoblasts and dentin. These non-collagenous components have important functions in mineral nucleation and crystal growth.
Table 5.3 Summary of the global composition of dentin.
Component | By Weight | By Volume |
Mineral content | 68–70% | 50% |
Organic content | 21% | 30% |
Water (free and bound) | 11% | 20% |
Note: Substantial differences occur between the different types of dentin described in the text.
Table 5.4 Components of the extracellular organic matrix
Component | Protein Family | Specific Proteins |
Collagen (90%) | Collagens | Type I (~90%) and Type I trimer (~11%) Type III and V (1–3%): During dentin formation and detected in some pathology |
Non-collagenous proteins (10%) | Phosphorylated SIBLINGs (Implicated in mineral nucleation and crystal growth, but also inhibitors in some cases) | Dentin sialophosphoprotein (DSPP): cleaved immediately after secretion into *Dentin SialoProtein (DSP), *Dentin Glycoprotein (DGP) and *Dentin Phosphoprotein (DPP) Dentin matrix protein1 (DMP1) Bone sialoprotein (BSP) Osteopontin (OPN) Matrix Extracellular Phosphorylated Glycoprotein (MEPE) |
Transient phosphorylated protein expressed by young odontoblasts | Amelogenin | |
Other ECM proteins (non-phosphorylated) | Osteonectin Osteocalcin Serum-derived proteins (α2HS glycoprotein, albumin) |
|
Proteoglycans | Decorin, biglycan, fibromodulin, osteoadherin | |
Growth factors | Transforming growth factor β (TGFβ), fibroblast growth factors (FGFs), insulin-like growth factors 1 & 2 (IGF-1 & -2), vascular endothelial growth factor (VEGF), bone morphogenetic proteins (BMPs) | |
Enzymes | Acid & alkaline phosphatases, matrix metalloproteinases (MMPs), tissue inhibitors of metalloproteinases (TIMPS), A disintegrin and metalloproteinase (ADAMs), A distintegrin and metalloproteinase with thrombospondin motifs (ADAMTS) | |
Proteolipids/phospholipids | Intracellular (membrane) and extracellular |
Dentin formation
Pre-odontoblast differentiation and tooth morphogenesis
As described in Chapter 3, at early stages of tooth formation, neural crest-derived mesenchymal cells migrate toward the first branchial arch. Interaction between these cells (condensing mesenchyme) and epithelial cells located specifically at placode sites leads to the proliferation of the epithelial cells and formation of a dental lamina. The two tissues form the rudiment of the embryonic tooth, epithelial cells forming the enamel organ and mesenchymal cells forming the dental papilla, or embryonic pulp (Fig. 5.9).
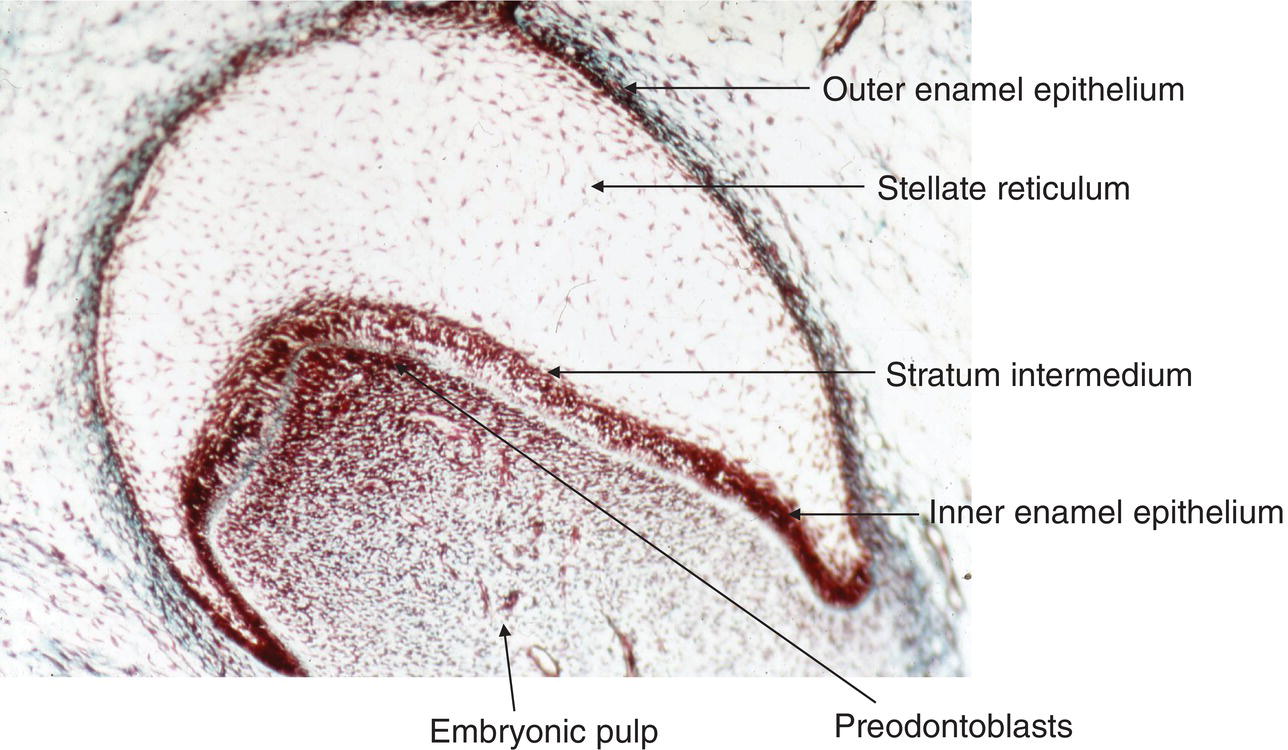
Figure 5.9 Light micrograph of a tooth germ at the bell stage. The enamel organ comprises four layers: the outer enamel epithelium, the stellate reticulum, the stratum intermedium, and the inner enamel epithelium. A basement membrane separates the inner enamel epithelium from the pre-odontoblasts at the tip of the pulp horn. These cells line the periphery of the embryonic pulp.
Crown morphogenesis starts with the formation of dental buds, followed by the cap stage, and later by early and late bell stages. The lateral proliferation of epithelial cells occurring at the periphery contributes to increase the diameter of the enamel organ. Four epithelial layers form the enamel organ: the outer enamel epithelium, the stellate reticulum, the stratum intermedium, and the inner enamel epithelium, the latter eventually differentiating to ameloblasts (Figs. 5.9–5.12). The cells undergo a series of divisions, leading to the formation of folds, the onset of shaping grooves and cusps. In the mouse molar, cell division in the embryonic mesenchymal pulp stops before it does in the enamel organ, leading to a larger surface of inner enamel epithelium compared with pre-odontoblasts. Folds begin to form, leading to cusp formation. In the central area of the enamel organ, the enamel knot regulates the morphogenetic process, influencing the process through cell proliferation and apoptosis.
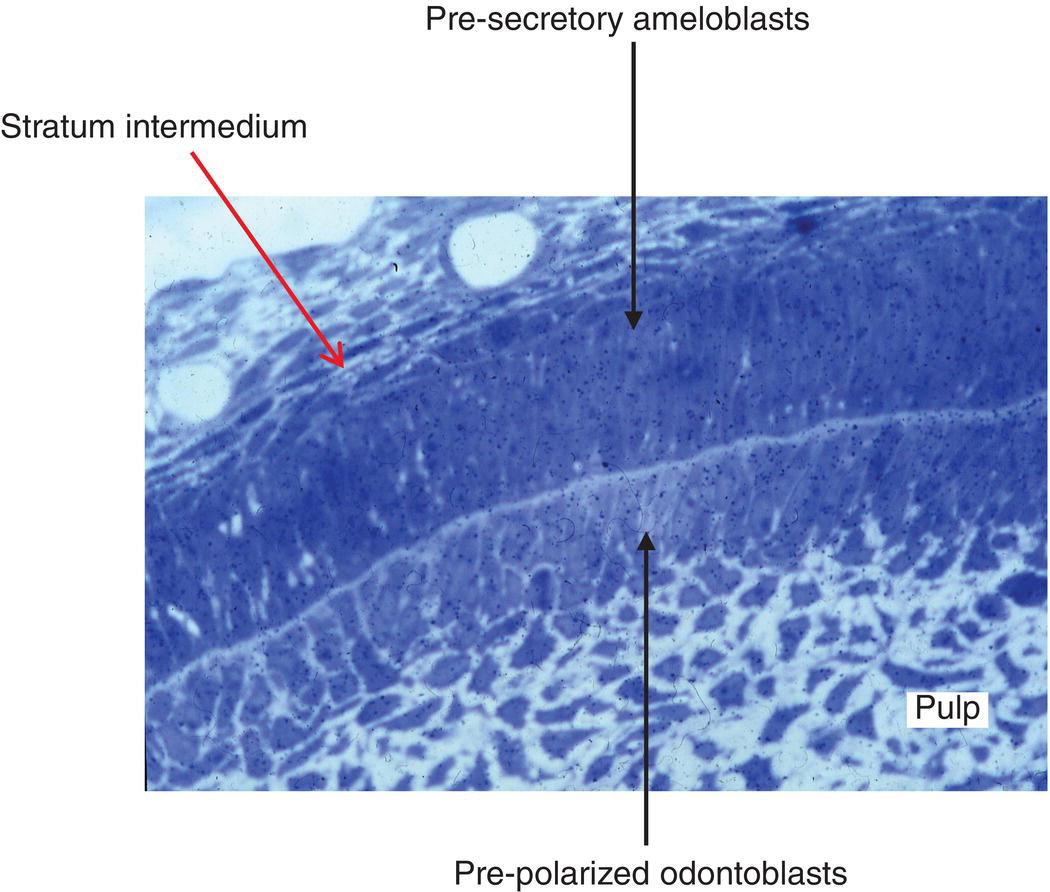
Figure 5.10 Light micrograph of developing rat incisor. The stratum intermedium and presecretory ameloblasts are facing pre-polarized odontoblasts. Between the two layers of cells, the formation of predentin has already been initiated. Numerous pulpoblasts (fibroblasts) are present within the dental pulp.
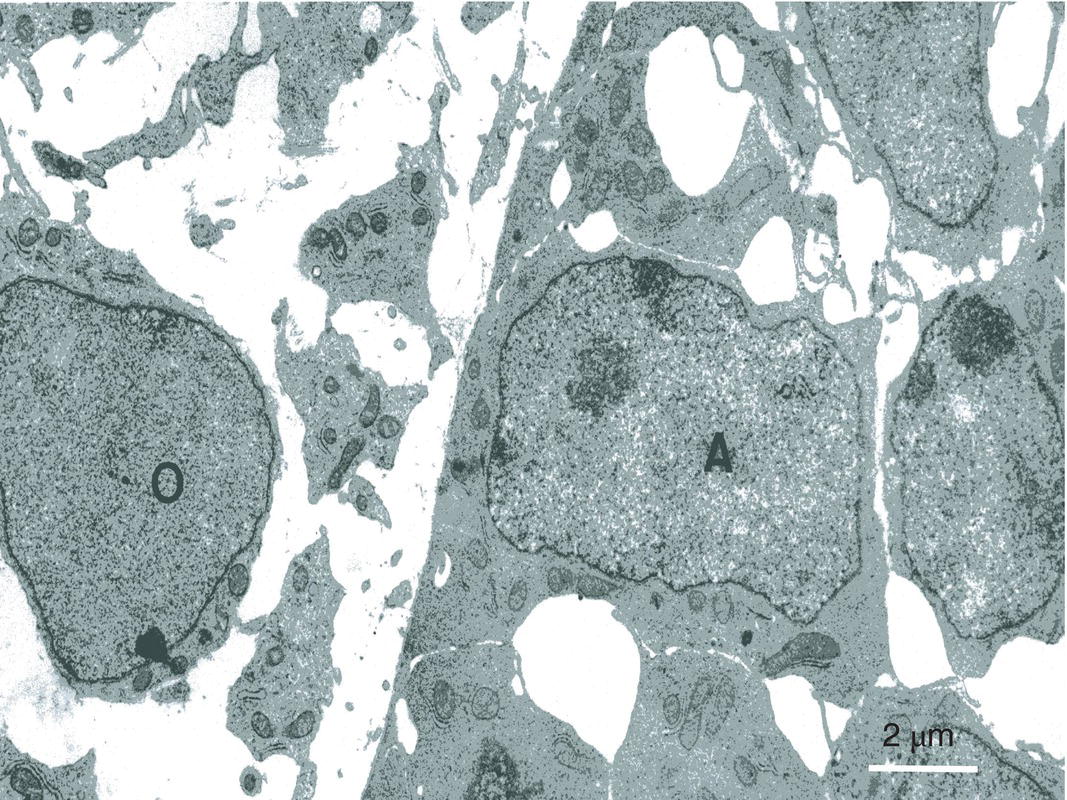
Figure 5.11 In the inner enamel epithelium, presecretory ameloblasts (A) establish a series of intercellular junctions. At the surface of the embryonic pulp polarizing presecretory odontoblasts (O) have not yet begun to produce dentin. Bar = 2 μm.
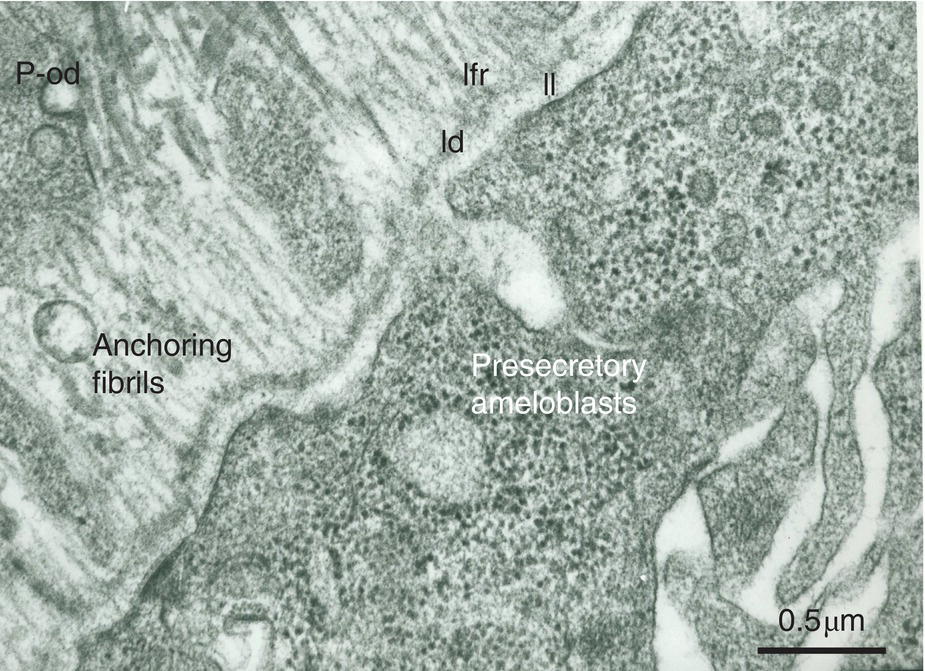
Figure 5.12 Ribosome-rich presecretory ameloblasts form a continuous cell layer. They produce the three parts of the basement membrane (lamina lucida, ll; lamina densa, ld; lamina fibroreticularis, lfr). Anchoring fibrils are the earliest components synthesized by the pre-odontoblasts (p-od). They are at right angles with the basement membrane. Bar = 0.5 μm.
Epithelial-mesenchymal interactions are instrumental in the transition from mesenchymal embryonic pulp cells to a pre-odontoblastic stage. The cells at the periphery of the embryonic pulp undergo asymmetric division. After the last mitosis, the larger cells in contact with the basement membrane (BM) (basal lamina) become early pre-polarized odontoblasts (Figs. 5.10 and 5.11). These cells ultimately differentiate into polarized secretory odontoblasts (Figs. 5.13a and b). The cells some distance away from the BM are smaller and retain their potential as stem cells. They are grouped in the sub-odontoblastic cell layer of Hoehl (Figs. 5.2 and 5.3), which may differentiate ultimately toward the odontoblastic lineage. It is crucial to highlight that odontoblasts are post-mitotic cells. In case of carious decay or odontoblast injury, differentiation of Hoehl’s cells is reactivated and polarized secretory odontoblasts are implicated in reactionary dentin formation (Figs. 5.14–5.16b and 5.16c). If both the odontoblastic and Hoehl’s cell layers are irreversibly altered, pulp progenitors contribute to the formation of reparative dentin (Fig 5.16a). As odontoblasts become older they gradually are reduced in number. Hoehl’s cells then may become activated and differentiate into new odontoblast-like cells.
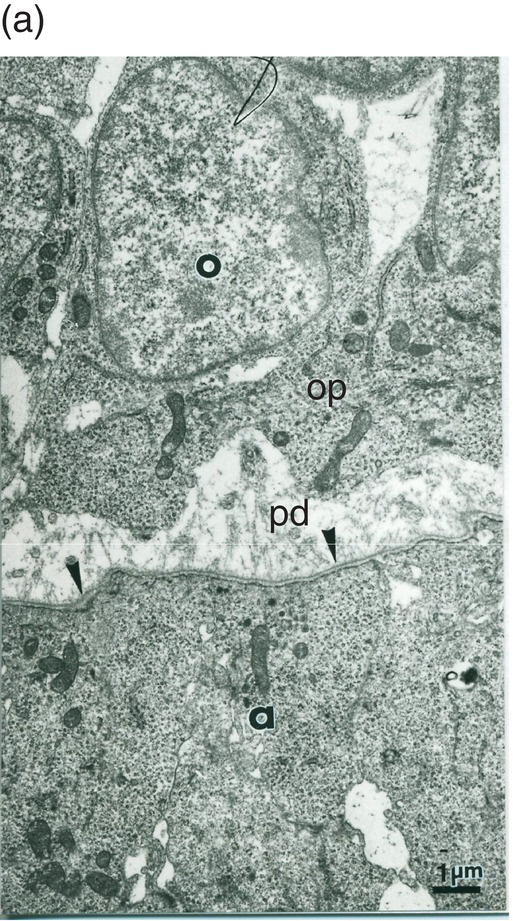
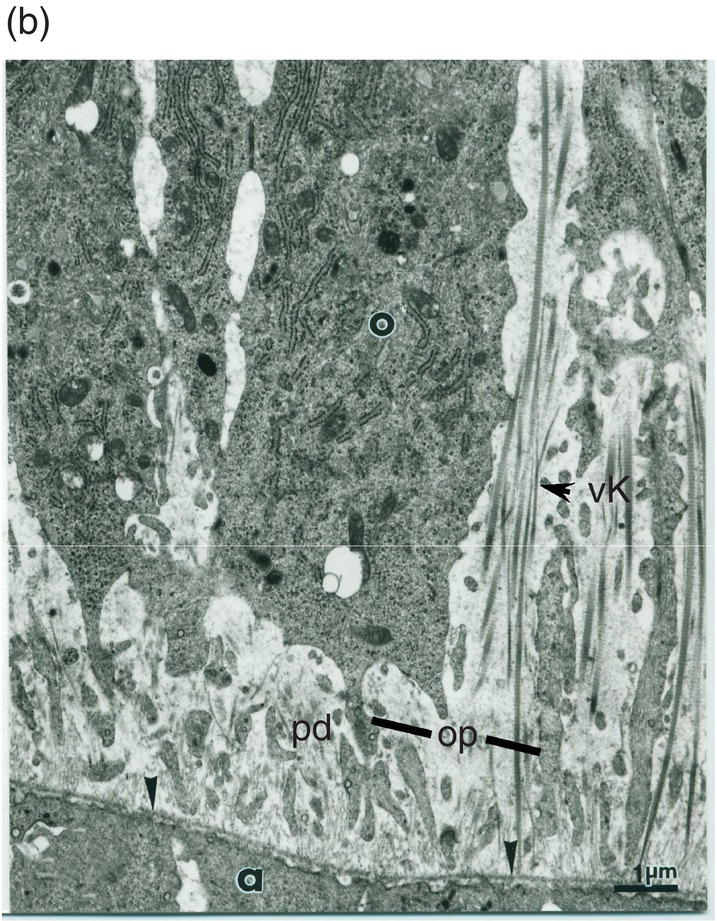
Figure 5.13 (a) Early dentinogenesis. A basement membrane (arrowheads) is present at the distal surface of the presecretory ameloblasts (a). Polarizing odontoblasts (o) display developing processes (op). A few fibrils are located in the initial predentin (pd). Bar = 1 μm. (b) At a later stage, polarizing odontoblasts (o) display an increase in rER cisternae. Between cell bodies, bundles of thick collagen fibrils are probably representative of the von Korff fibrils (vK). Bar = 1 μm.
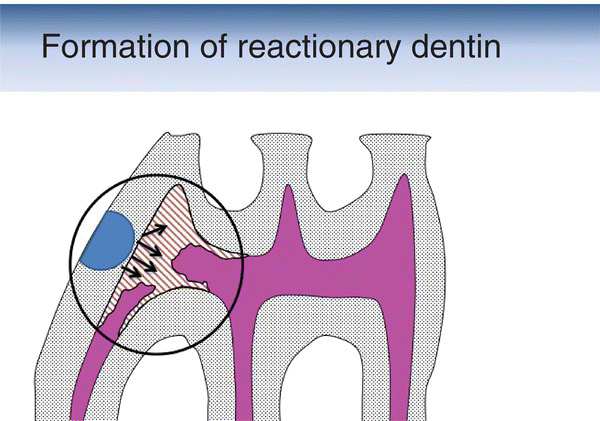
Figure 5.14 Schematic drawing showing the formation of reactionary dentin (brown-hatched area) after the preparation of a cavity (blue semicircle) in the mesial aspect of the first maxillary molar of a rat. Pulp (magenta).
(Based on: Decup, F., Six, N., et al. (2000) Bone sialoprotein-induced reparative dentinogenesis in the pulp of rat’s molar. Clinical Oral Investigations, 4(2), 110–119.)
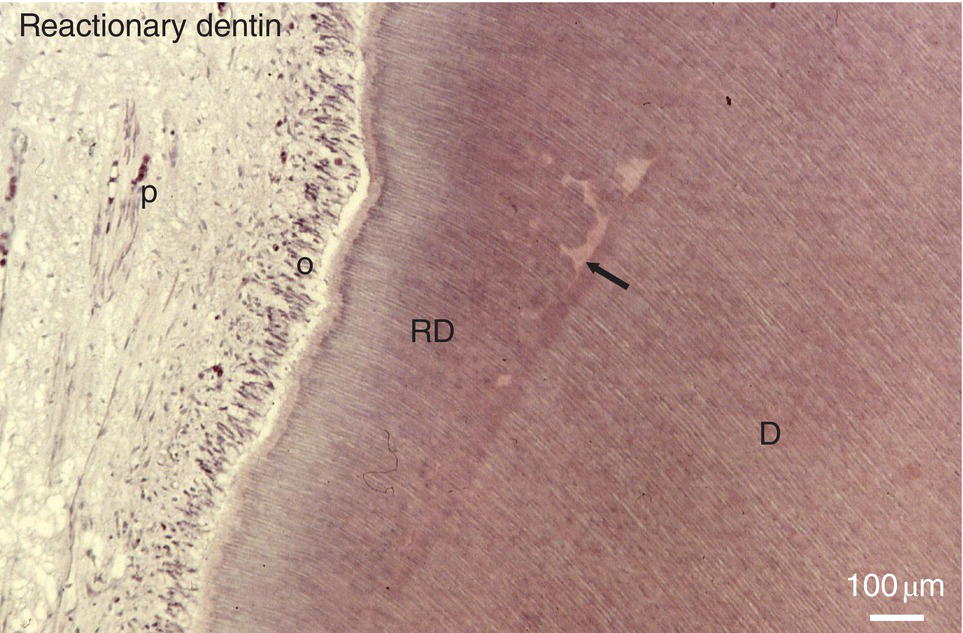
Figure 5.15 Formation of reactionary dentin (RD) in the dental pulp of a human premolar 90 days after the filling of a cavity. Dentin (D); pulp (P); odontoblasts (o); interglobular spaces (arrow). Bar = 100 μm.
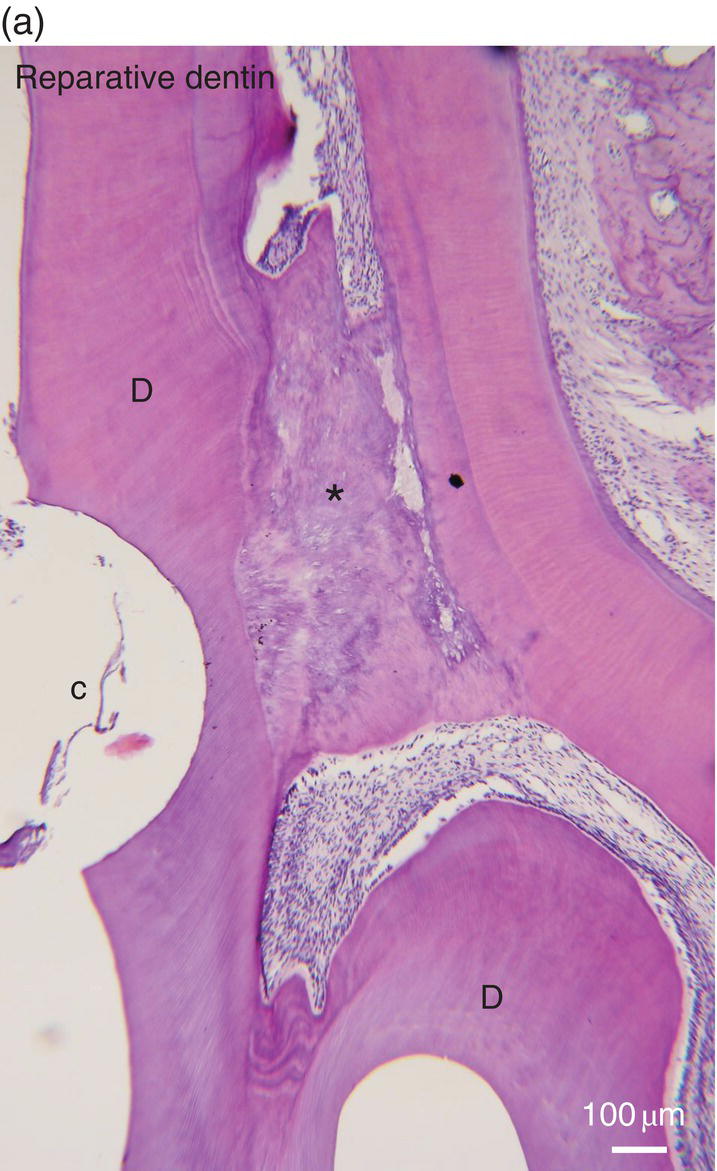
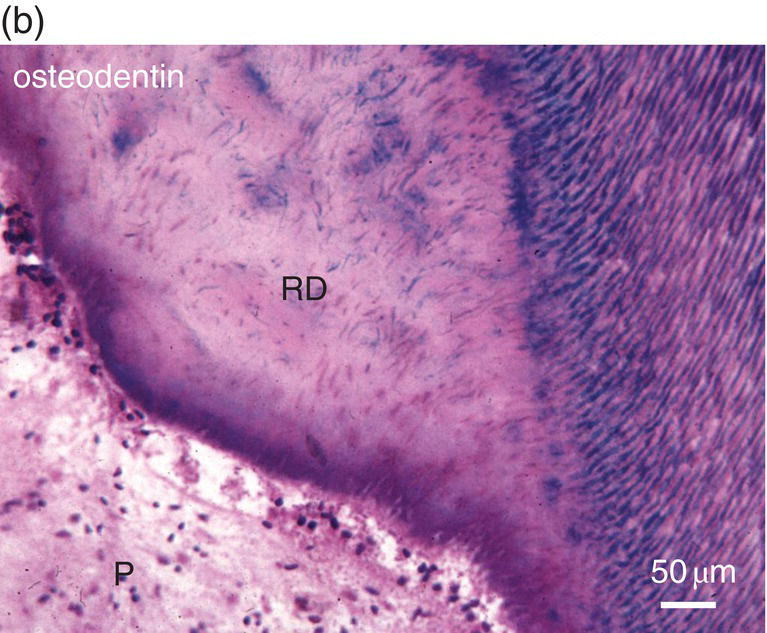
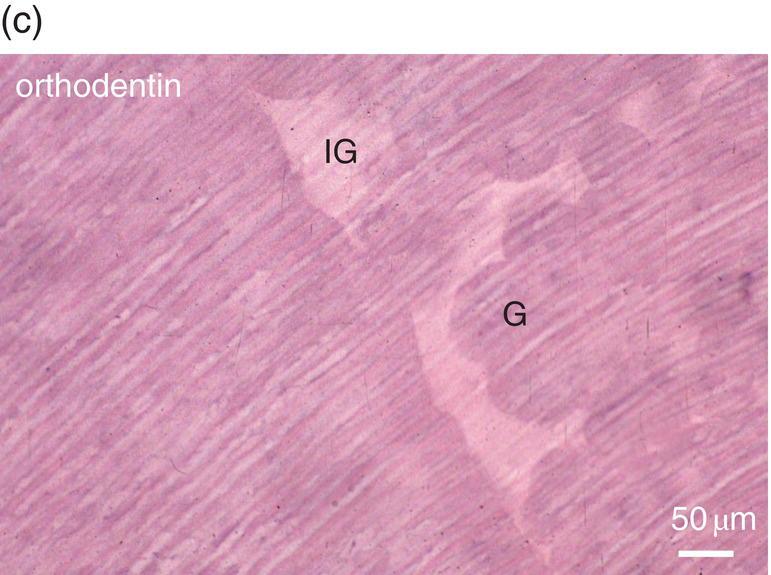
Figure 5.16 (a) Ninety days after a pulp exposure and filling of the cavity (c) with a dental cement, reparative dentin (*) partially occludes the exposure of the mesial root pulp. Dentin (D). Bar = 100 μm. (b) Human tooth: Reactionary osteodentin (RD), stains as a pink/violet structure, whereas orthodentin (right part of the figure) contains purple tubular dentin. Pulp, (P). “Stains all” method. (c) After the preparation of a cavity in a human premolar and glass ionomer filling, reactionary orthodentin is formed, characterized by globular structures (G) and interglobular spaces (IG). Bars in b and c = 50 μm.
Polarizing post-mitotic odontoblasts
Odontoblasts are grouped in a restricted layer at the periphery of the pulp. The odontoblast layer includes three to four rows of cell bodies. In the distal cell body, polarizing post-mitotic odontoblasts establish intercellular junctional complexes, including a series of gap and desmosome-like junctions. Initially, odontoblast processes are short, coated with carbohydrates/oligosaccharides, and adherent to the BM. As newly formed dentin is deposited, odontoblast cell bodies move backward toward the central part of the pulp, and the cell processes elongate. Secretory molecules are initially released all around the cell bodies. Thick interodontoblastic collagen fibers (von Korff fibers), consisting of Type III collagen, contribute to the anchorage of the cellular compartment to the initial forming dentin (Fig. 5.13b). During the terminal cell polarization, the secretion of collagen and non-collagenous proteins is gradually restricted to the distal part of the cell bodies. Secretion then occurs in the predentin and along the mineralization front.
Polarized secretory odontoblasts
Odontoblasts form dentin, a mineralized structure resulting exclusively from the biological activities of these cells. Schematically, intertubular dentin formation occurs within a three-layer system, consisting of the cell bodies, the predentin, and the dentin. Most extracellular matrix (ECM) molecules are synthesized and secreted by the odontoblasts (Figs. 5.2, 5.17–5.19). Very few molecules are synthesized elsewhere and take an interodontoblastic pathway, cross the terminal intercellular barrier, and diffuse in the predentin/dentin areas. Although this is the case for albumin and α2HS glycoprotein (fetuin), serum-derived molecules are a very minor component of dentin ECM.
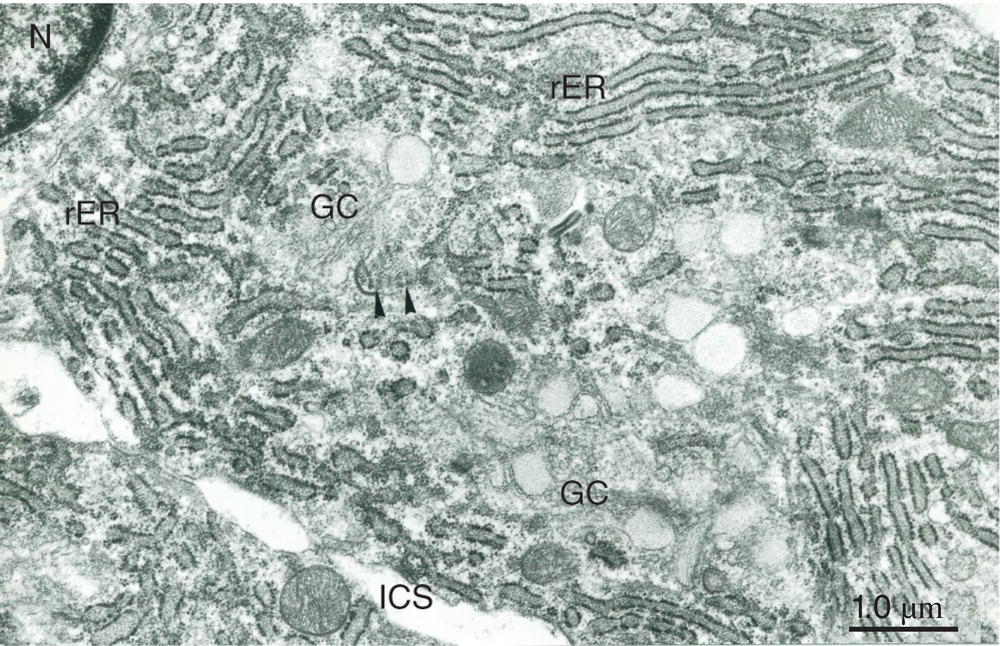
Figure 5.17 Cell body of a secretory odontoblast. Abundant rough endoplasmic reticulum (rER) fills the cytoplasm, and a prominent Golgi complex (GC) is present. Arrowheads indicate the collagen-like content of an abacus-like vesicle. Nucleus (N); intercellular space (ICS). Bar = 1 μm.
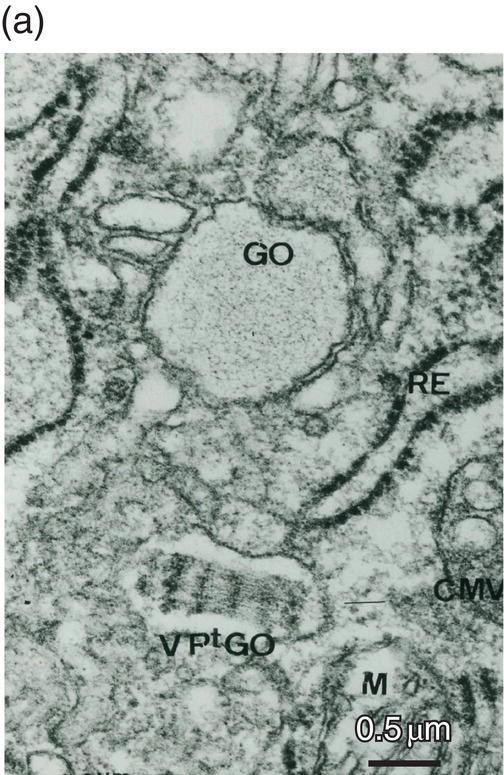
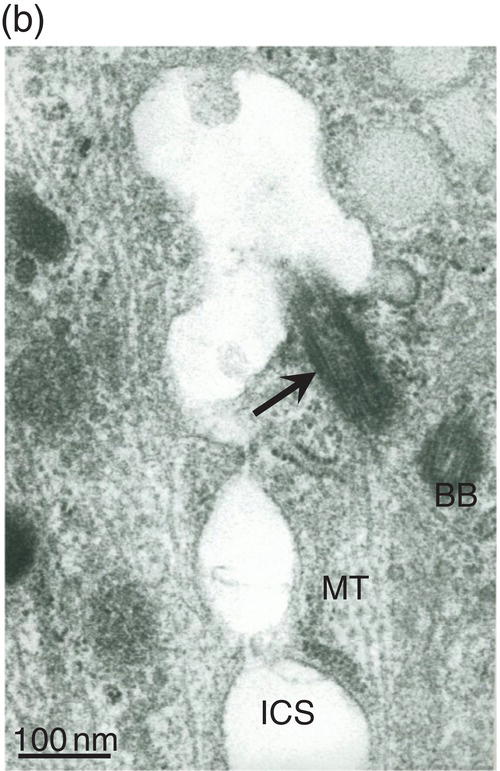
Figure 5.18 (a) Golgi region of an odontoblast cell body. Accumulation of procollagen abacus-like bundles in post-Golgi vesicles (VPtGO) is characteristic of collagen secreting cells. Rough endoplasmic reticulum (RE); Golgi vesicle (GO); mitochondrion (M). Bar = 0.5 μm. (b) Cytoskeletal proteins in the cell body. A cilium (arrow) and basal body (BB) are located near the intercellular space (ICS) between odontoblast cell bodies. Microtubules (MT). Bar = 100 nm.
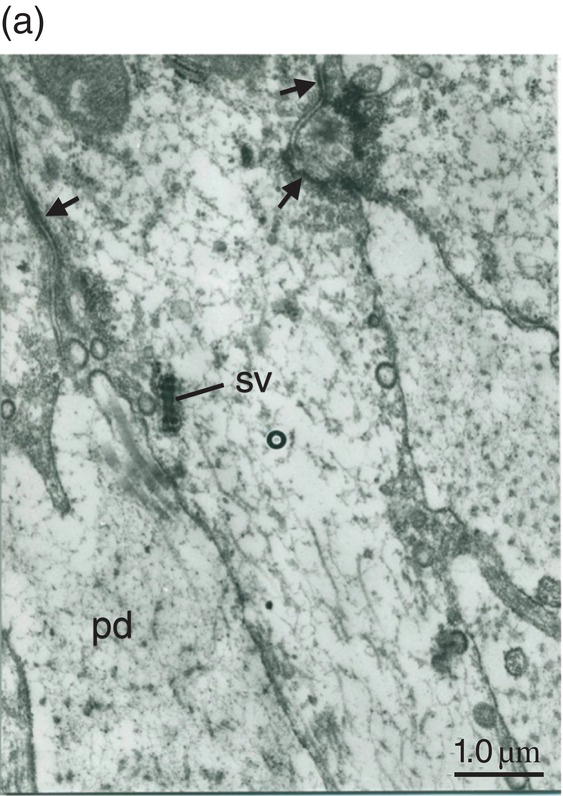
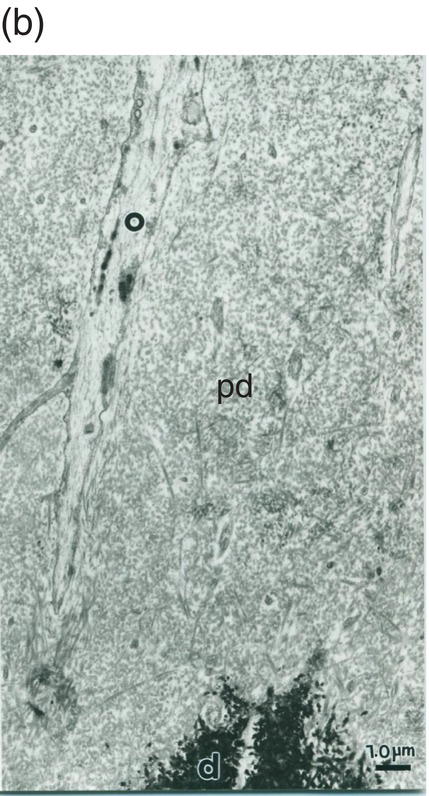
Figure 5.19 (a) Odontoblast process (o) in predentin (pd). Intercellular junctions (arrows) hold adjacent cells together. Secretory vesicle (sv). Bar = 1 μm. (b) Odontoblast process (o) extends through the predentin (pd) and reaches dentin (d). Bar = 1 μm.
The cell body layer
At the periphery of the dental pulp, odontoblast cell bodies form a layer infiltrated by capillaries (Fig. 5.3). Odontoblast cell bodies 3 μm wide and 20 to 40 μm in length display three anatomically distinct parts, as follows.
- The basal part, where mitochondria accumulate around the nucleus, whereas rough endoplasmic reticulum (rER) occupies the lateral borders.
- The central part, with lateral stacks of rER cisternae, a few supranuclear Golgi complexes randomly oriented, immature secretory vesicles, and lysosomal multivesicular bodies. Some vesicles contain abacus-like structures showing periodicity. The repeated 1000-nm banding suggests accumulation of procollagen, larger than the periodic 640-nm banding pattern of collagen fibrils. Immunolabeling reveals a close link between the intracellular collagen and the abacus-like vesicles, also identified as acid-phosphatase-containing structures. Hence, it was first believed that these abacus-like vesicles were implicated in the collagen secretory pathway, but now it is clear that the nonsecreted collagen is stored and destroyed within these vesicles, actually recognized as lysosomes (Figs. 5.17 and 5.18). Cytoskeletal proteins are implicated in the cell body in the formation of cilia, but also shape the cells. These include tubulin in the form of microtubules and molecules implicated in secretion/re-internalization: actin microfilaments and vimentin intermediate filaments, all of them contributing to space information, exocytosis, and endocytotic events (Fig. 5.19).
- The distal part contains clusters of mitochondria. They accumulate together with large lysosomes and small secretory vesicles containing procollagen microfibrils. Near the predentin, gap and desmosome-like intercellular junctions seal the lateral membranes (Fig. 5.19). In a few non-human species, some tight junctions are also identified.
A sub-plasmalemmal undercoat is formed by microfilaments. Inside the odontoblast processes, microtubules and intermediate filaments, such as nestin, constitute a developed network. Mitochondria are smaller compared to those present in the cell body. Internalization of clathrin-coated vesicles contributes to the uptake of degraded molecules or cleaved peptides, which are transferred to the large lysosomes located in the distal cell bodies. Secretion of extracellular matrix proteins occurs by exocytosis of secretory vesicles. Because exocytosis occurs within a few milliseconds, it is very difficult to detect under a microscope. Empty membrane ghosts or electron-lucent areas may characterize the secretory vesicles at the electron microscope level.
Extracellular matrix precursor molecules are delivered by blood vessels, diffuse through the fenestrations of endothelial cells, and cross the semipermeable BM limiting the capillaries. Amino acids are incorporated into the forming protein in the rER of the odontoblasts. The addition of initial sugars such as mannose occurs within the rER, whereas the terminal sugars, e.g., fucose, are added in the Golgi complex. Sulfate and phosphate are incorporated into the proteins directly in the Golgi saccules. The newly synthesized ECM components are conveyed from the presecretory to secretory vesicles where they mature and are secreted after enzymatic processing. The C-terminal non-helical extensions of the procollagen are cleaved in the distal cell bodies by a procollagen peptidase, identical to a bone morphogenetic protein (BMP). After secretion, the N-terminal extensions are cleaved by a peptidase in the proximal predentin. The non-helical extensions are recaptured by the cells and transferred to the distal lysosomes. This recapture provides feedback information to the odontoblasts.
Odontoblast cell processes expand some distance away within the tubules. The actual length of the process is still a matter of discussion. Processes may extend from the pulp to the dentinoenamel junction, but most are probably limited to the inner one-third or one-half of the dentin.
The predentin compartment
Between the cell bodies and dentin, the predentin forms a layer with a constant thickness (15–20 μm in width). The collagen molecules in this non-mineralized compartment are secreted from the odontoblast processes. After secretion into the predentin, the native collagen fibrils increase in thickness (lateral aggregation) and elongate (end-to-end association due to telopeptide interactions, consequently contributing to the lengthening of the collagen fibrils). Initially, just after their assembly, collagen fibrils in the inner predentin display a 20-nm average diameter. At the end of the fibrillation process, in the distal predentin, collagen fibers show a 70- to 80-nm mean diameter. Proteoglycans such as decor/>
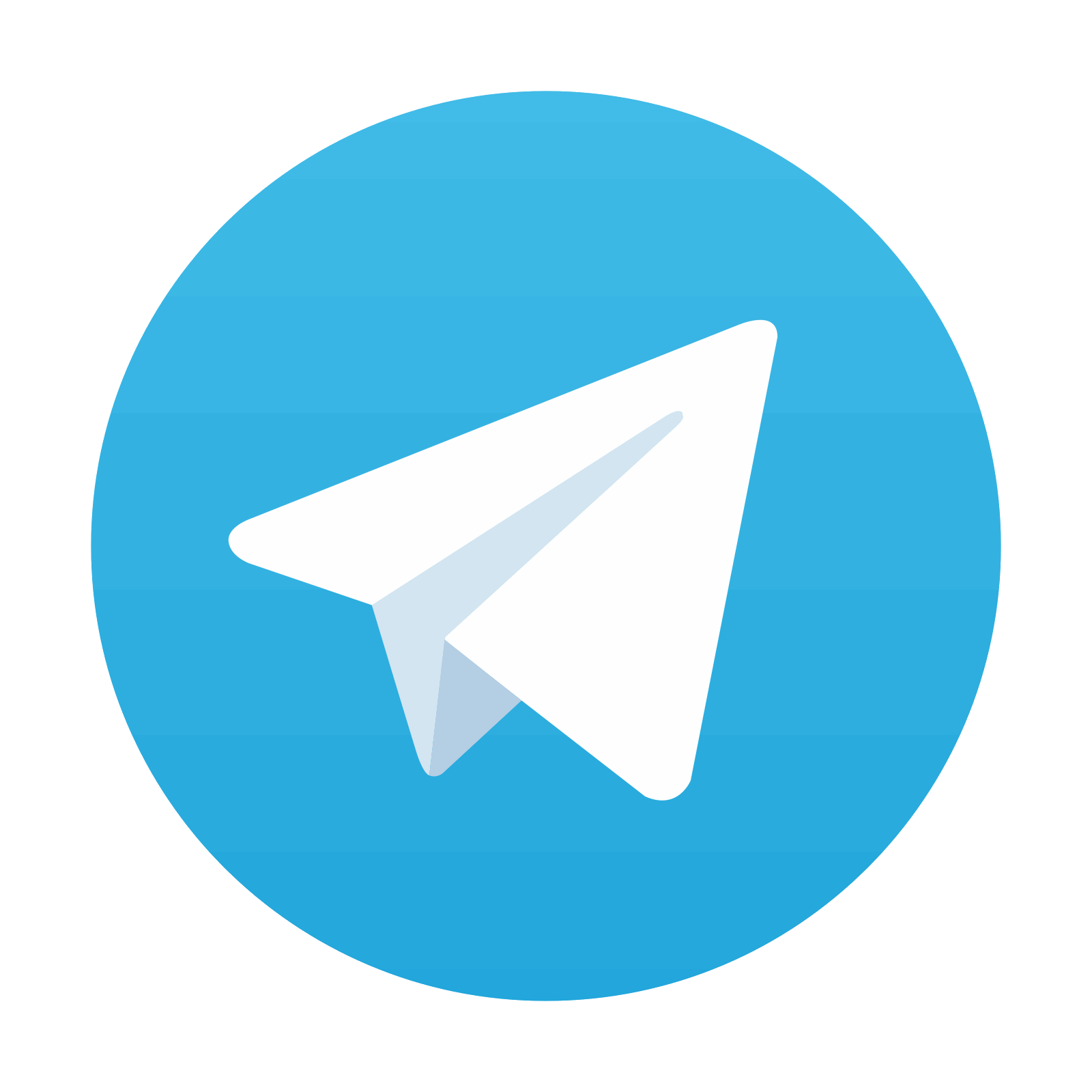
Stay updated, free dental videos. Join our Telegram channel

VIDEdental - Online dental courses
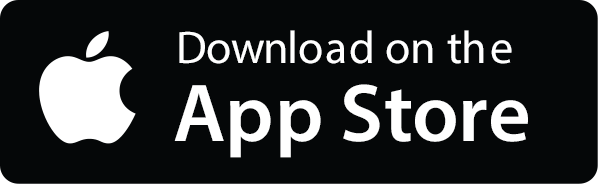
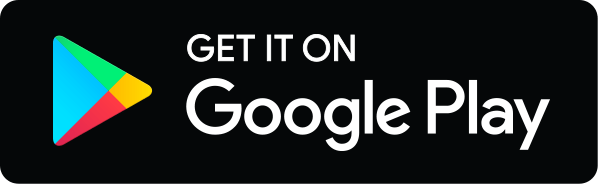