Abstract
This study verified the resistance to displacement of six miniplate fixation methods after sagittal split osteotomy (SSO). SSO was performed in 30 polyurethane synthetic mandible replicas. The distal segments were advanced (4 mm) and specimens were grouped according to the fixation method: four-hole standard miniplate; four-hole locking miniplate; six-hole standard miniplate; six-hole locking miniplate; six-hole standard sagittal miniplate; six-hole locking sagittal miniplate. Biomechanical evaluation was performed by applying compression loads to three points on the second molar region, using an Instron universal testing machine until a 3 mm displacement of the segments occurred. Compression loads able to produce 3 mm displacement were recorded in kN and subjected to analysis of variance ( P < 0.01) and Tukey’s tests for comparison between groups ( P < 0.05). The locking sagittal miniplate showed higher resistance to displacement than the regular four- and six-hole locking and standard miniplates. No significant differences were observed between the locking sagittal miniplate and the regular sagittal or the four-hole locking miniplates. Two of the three groups with the best results had locking plate fixation methods. Fixation of SSO with a single miniplate is better accomplished using six-hole locking sagittal miniplates, six-hole standard sagittal miniplates, or four-hole locking miniplates; these methods are more resistant to displacement.
Mandibular sagittal split osteotomy is a widely employed technique for correction of dentofacial deformities directly or indirectly affecting the mandible. While most post-surgical complications of this procedure are related to failure in fragment fixation, no fully efficacious stabilization technique is currently known. Among possible complications are relapses, lack of stability, and displacement of the condyle after fixation. The best results are generally observed when plates and/or screws are used.
The greatest difficulty in assessing bone segment stability by biomechanical tests is achieving an adequate simulation of the masticatory musculature function during mandibular movements. The early biomechanical testing model involved two compression points, with one end of the specimen suspended as a cantilever. Recently, a three-point model was developed and employed in studies focusing on the evaluation of different methods of mandibular sagittal split osteotomy fixation.
Despite all the research and advancements in different methods of osteosynthesis, oral surgeons are still unsure which fixation technique to select to achieve the best results with the least amount of trouble, especially when dealing with large mandibular advancements or facial asymmetry. With new designs of osteosynthesis plates being constantly launched by the industry, studies focusing on investigation of their performances are encouraged.
Generally, fixation plates should conform perfectly to the cortical bone in order to prevent disruption in the alignment of fragments, which might induce undesirable changes in dental occlusion or in the condyle-fossa relationship. The locking miniplates/screw systems routinely used prevent displacement of the segments even when the fixation plate is not in full contact with the cortical bone. In this system the bone segments are not compressed against the plate and the screws. Haug et al. compared locking and standard (non-locking) plates in mandibular angle fractures and observed that the degree of adaptation of the locking plate to the receptor site does not interfere with stability of the fixation, this differs from what occurs when standard plates are used.
Gutwald et al. verified in vitro that 2.0 mm locking plates show greater resistance than conventional plates and screws. Similar results have been reported by other authors.
The use of locking systems has been extensively proposed for use during treatment of facial fractures, but their applications in orthognathic surgery deserve further attention.
Aiming to achieve less rigid osteosynthesis while maintaining enough stability to ensure good adaptation of the condyle to the mandibular fossa, the use of a single miniplate has been suggested. Given the different options available for fixation of mandibular osteotomies, it is important to investigate which plate designs promote the best stability in these clinical situations.
The aim of this study was to compare the biomechanical stability of three different designs of standard (non-locking) and locking single fixation plates for osteosynthesis after mandibular sagittal split osteotomy.
Material and method
This study involved the use of 30 polyurethane synthetic mandible replicas (Synbone, Lauddquart, Switzerland). An acrylic resin matrix was positioned on the buccal and lingual surfaces of the specimen to indicate the area to be sectioned. After sectioning, the distal segment of the mandible was advanced 4 mm and fixed with a Neoface system miniplate (Neoortho, JJGC Indústria e Comércio de Materiais Dentários, Curitiba-PR, Brazil).
Specimens were divided into 6 groups, with 5 mandibles each, according to the fixation method used. Group 1 used one four-hole standard (non-locking) miniplate, with two holes placed on the proximal segment and two holes placed on the distal, fixed by standard screws measuring 2.0 mm × 6 mm ( Fig. 1 ). Group 2 used one four-hole locking miniplate, with two holes placed on the proximal segment and two holes placed on the distal, fixed by locking screws measuring 2.0 mm × 6 mm ( Fig. 1 ). Group 3 used one six-hole standard (non-locking) miniplate, with three holes placed on the proximal segment and three holes placed on the distal, fixed by standard screws measuring 2.0 mm × 6 mm ( Fig. 1 ). Group 4 used one six-hole locking miniplate, with three holes placed on the proximal segment and three holes placed on the distal, fixed by standard screws measuring 2.0 mm × 6 mm ( Fig. 1 ). Group 5 used one six-hole standard (non-locking) sagittal (horizontal double Y design) miniplate, with three holes placed on the proximal segment and three holes placed on the distal, fixed by locking screws measuring 2.0 mm × 6 mm ( Fig. 1 ). Group 6 used one six-hole locking sagittal (horizontal double Y design) miniplate, with three holes placed on the proximal segment and three holes placed on the distal, fixed by locking screws measuring 2.0 mm × 6 mm ( Fig. 1 ).

The specimens were subjected to compression loads, as described by Armstrong et al. The mandible replicas were positioned on the base of the testing apparatus, to simulate the resistance at three areas of the specimen: point A determined the resistance at the condyle and posterior regions of the mandible; point B simulated the resistance of the food bolus; and point C, on the anterior portion of the mandibular base, simulated the resultant forces that act during mastication (points A, B, and C; Fig. 2 ).

One indentation was produced with a bur on the proximal segment and another on the distal segment, on the base of the mandible. The distance between the two indentations was measured with a surgical calliper, and 3 mm were added to this measurement ( Fig. 2 ). A compressive load was applied to the specimen by an Instron universal testing machine (Model 4202, Norwood, MA, USA) until a 3 mm displacement of the fragments was observed. At this moment, the compressive load was quantified and recorded.
The values associated with the compression loads applied to the specimens in the six groups were subjected to analysis of variance after square root transformation was performed to verify normality of the sample. Multiple comparisons between the groups were carried out by Tukey’s test ( P < 0.05).
Results
As shown in Table 1 , the load values show greater resistance for Group 6 (sagittal locking plate), followed by Group 5 (non-locking sagittal plate), Group 2 (four-hole locking plate), Group 1 (four-hole non-locking plate), Group 4 (six-hole locking plate), and Group 3 (six-hole non-locking plate). Increasing the number of holes from four to six on straight plates did not increase the resistance to displacement: Groups 3 and 4 had worse results than Groups 1 and 2.
Mandible 1 | Mandible 2 | Mandible 3 | Mandible 4 | Mandible 5 | Mean | |
---|---|---|---|---|---|---|
Group 1 | .049 | .047 | .048 | .047 | .055 | .049 |
Group 2 | .077 | .077 | .046 | .044 | .059 | .055 |
Group 3 | .035 | .059 | .041 | .044 | .055 | .046 |
Group 4 | .052 | .056 | .040 | .050 | .035 | .046 |
Group 5 | .071 | .071 | .044 | .072 | .062 | .064 |
Group 6 | .148 | .077 | .059 | .070 | .072 | .085 |
The horizontal double Y design of the six-hole sagittal plate increased the resistance to displacement, since these plates had better results than the straight six-hole plates: Groups 5 and 6 showed higher compression values than Groups 3 and 4.
The results from the analysis of variance are given in Table 2 . The results from Tukey’s statistical test ( Fig. 3 and Table 3 ) indicate that fixation using locking sagittal miniplates (Group 6) was significantly different from fixation with four-hole standard miniplates (Group 1), six-hole standard miniplates(Group 3), or six-hole locking miniplates (Group 4). Group 6 did not show statistically significant differences from Group 5 (standard sagittal plate) or from Group 2 (four-hole locking plate).
Source of variation | Degrees of freedom | Mean square | Sum of squares | F | P |
---|---|---|---|---|---|
Group | 5 | 0.01988965 | 0.00397793 | 5.23 | 0.0031 * |
Mandible | 4 | 0.00680557 | 0.00170139 | 2.24 | 0.1013 |
Residue | 20 | 0.01520903 | 0.00076045 | ||
Total | 29 | 0.04190424 |
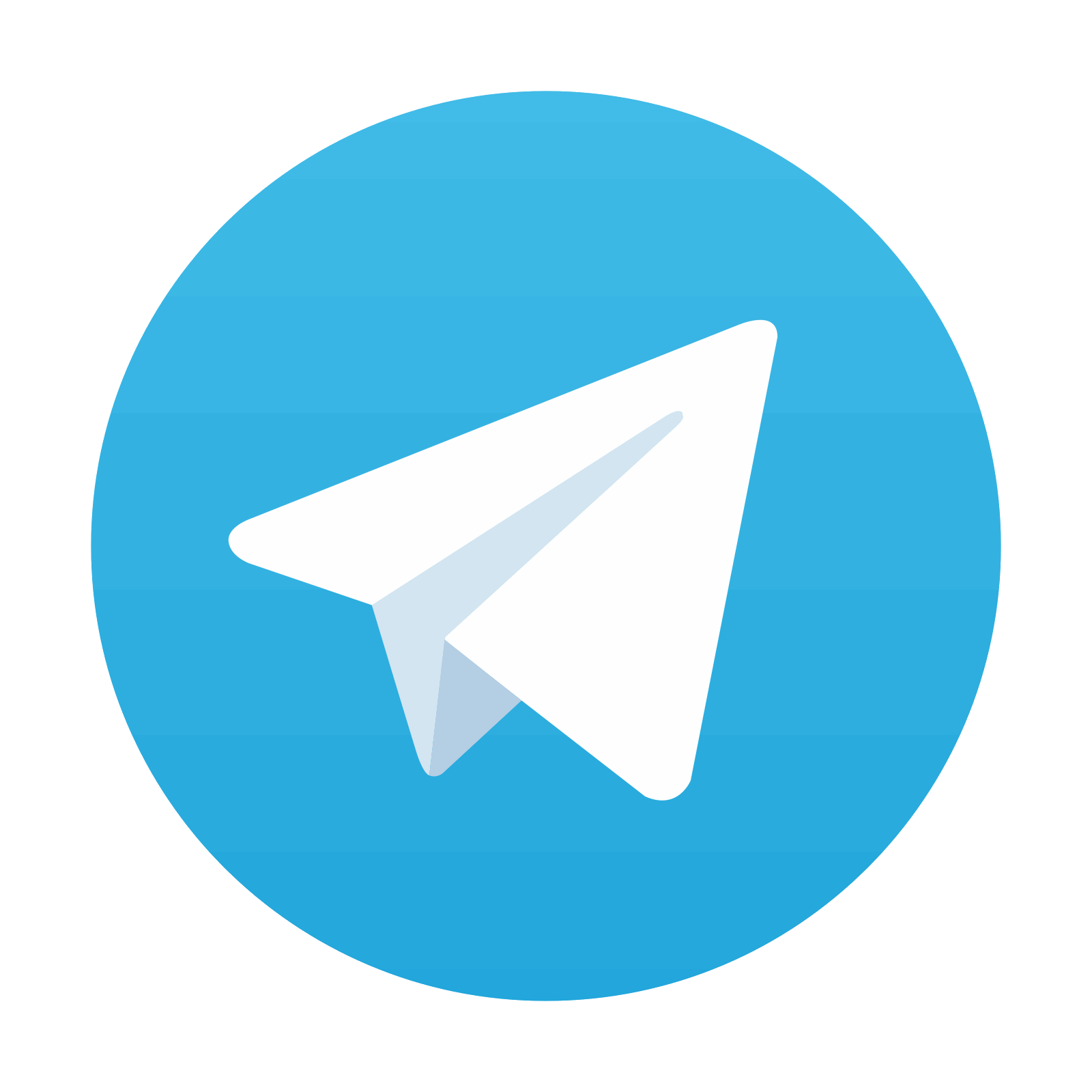
Stay updated, free dental videos. Join our Telegram channel

VIDEdental - Online dental courses
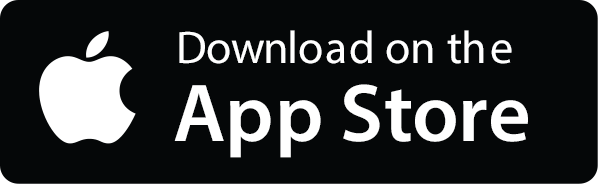
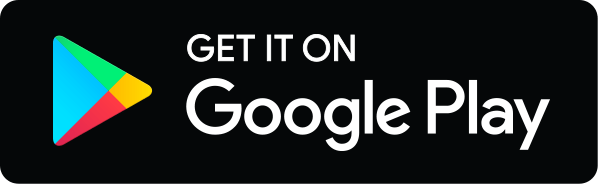
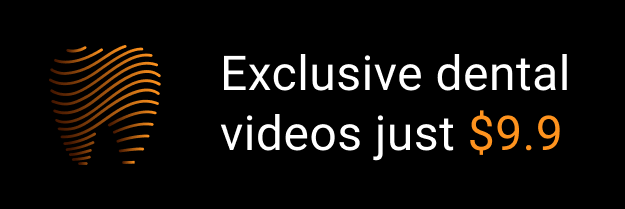