Abstract
The purpose of this study was to evaluate the properties of a novel inorganic xenogenic bone substitute, calcinated antler cancellous bone (CACB). Physicochemical properties of CACB including surface morphology, phase composition, chemical bond structure, Ca/P ratio and porosity were characterized by scanning electron microscopy, X-ray diffraction spectroscopy, Fourier-transform infrared spectroscopy, inductively coupled plasma-atomic emission spectroscopy and nitrogen adsorption analysis, and were found to closely resemble calcinated human cancellous bone. The bone defect repair efficacy of CACB was evaluated in comparison with commercially available bone substitutes (Bio-Oss ® ) within rabbit mandible defects. The gross observation, micro-CT and histology analysis data demonstrated that CACB was efficacious for bone regeneration, and was comparable with Bio-Oss ® bone substitute in inducing neovascularization and osteogenesis within the mandible defects. CACB can therefore serve as a safe, renewable, and sustainable source of bone graft material, but without the ethical issues pertaining to animal welfare.
Bone graft implantation is a major treatment modality for bone deficiencies arising from various diseases, traumas and congenital defects, with effective clinical outcomes being reported. Although autologous bone graft is considered the gold standard in clinical practice, extensive research effort is focused on synthetic materials to avoid the problems of limited availability, additional surgery, and potential donor site morbidity associated with autologous bone grafts. The clinical application of artificial bone substitutes is uncommon and utilized for only a narrow range of indications because of their uncertain clinical efficacy and lack of osteoinductive properties. Xenogenic grafts are among the most common materials utilized clinically owing to their ready availability and good osteoconductivity as a result of their origin from natural bone tissues of animals, examples are Bio-Oss ® or Endobon ® derived from bovine femur, and OsteoBiol ® derived from porcine bone.
Although the clinical performance of these inorganic xenografts bone substitutes has been confirmed and widely accepted by physicians and patients, the disadvantage of these products are their high price, the time-consuming manufacturing process, and controversial ethical issues pertaining to animal slaughter. Therefore, a safe, effective, sustainable and ethically acceptable bone graft substitute material would be of substantial clinical interest.
Antlers are the only body parts that can achieve complete regeneration in mammals, and are one of the fastest growing tissues, with a peak growth rate of 2–4 cm every day. This extraordinary growth rate is the result of the rich blood supply arising from the highly vascularized velvet tissue on the antler surface, and from the pedicle at the antler base. Every year, deer antlers begin to grow in spring (March–April), mature after 3–4 months of growth, and are naturally shed, to conserve energy, at the end of the autumn mating season. This cycle is repeated annually. The remarkable growth rate and material properties of antler make it an interesting model for bone growth and regeneration. Much interest is focused on the structural and mechanical properties of antler arising from their main function as the shield and weapon during mating combat. Little is known about the clinical efficacy of utilizing antler as a bone xenograft substitute for bone defect repair.
Antlerogenesis involves rapid deposition of calcium and phosphate in a short period of time, resulting in the formation of a cancellous bone-like structure in the central portions of antlers. Antlers have a similar composition to human cancellous bones and other mammalian long bones, but differ substantially from keratin-based mammalian horns. The authors hypothesize that calcinated antler cancellous bone (CACB) may serve as an ethically acceptable xenograft substitute for bone defect repair. In this study, the cancellous portions of naturally shed Sika deer antlers were calcinated at 800 °C for 6 h. The composition and physiochemical properties of CACB were rigorously characterized. The efficacy of CACB in promoting bone defect repair in situ in rabbit mandibles was evaluated compared with inorganic bone substitutes (Bio-Oss ® ) that are used clinically.
Materials and methods
Naturally-shed antlers from adult male Sika deer ( Cervus nippon ) were purchased from Jilin Wilderness Trading Company (Changchun, China). The commercially available Bio-Oss ® bone substitute was supplied by Switzerland Geistlich-Pharma, Co., Ltd. Human cancellous bone (gamma-irradiated and freeze-dried vertebral bone; Batch No. 3460285/2009) was kindly provided by Ao Rui Biological Material Co., Ltd. (Shanxi, China). Sodium hydroxide, chloroform methanol and hydrogen peroxide were obtained from Sigma-Aldrich Inc. (St. Louis, MO, USA).
Preparation and characterization of CACB
The cancellous portion of naturally-shed and fresh antlers was obtained by removing the cortex ( Fig. 1 A and B ). To eliminate organic components of the bone blocks, chemical treatment and high temperature calcination were carried out as previously described. Briefly, the cancellous bone blocks were immersed in 2% (w/v) sodium hydroxide for 12 h, and then in 30% hydrogen peroxide for 24 h; followed by washing in flowing tap water. The pieces were immersed in a 3:1 mixture of chloroform and methanol for 1 h and dried at 70 °C for 24 h. The pieces were sintered at 800 °C over a period of 6 h and maintained at that temperature for 3 h in a tube-type furnace to obtain CACB. The bone blocks were milled and sieved to obtain similar sized granules as the control material (Bio-Oss ® ) with diameter ranging between 1.0 and 2.0 mm ( Fig. 1 D).

The surface morphology and structure of the bone blocks were characterized using scanning electron microscopy (SEM; ZEISS, Supra 55, Germany). The phase composition and chemical bond structure of bone blocks were evaluated by X-ray diffraction spectroscopy (XRD, Rigaku D/max 2500 VB2+/PC, Japan) and Fourier-transform infrared spectroscopy (FT-IR, Nicolet 8700, USA). Inductively coupled plasma-atomic emission spectroscopy (ICP-AES, SPS8000, Thermo Elemental, USA) was used to analyze the Ca/P ratio of the CACB. The porosity was determined with nitrogen adsorption (brunauer–emmet–teller; BET isotherm) using a Sorpty 1750 (Carlo Erba) apparatus. For these analyses, the calcinated human cancellous bone was calcinated by the same method as that of CACB, and was used as a control.
Experimental animals and surgical procedures
All animal experiments were performed in accordance with a protocol approved by the Laboratory Animal Care and Use Committee of Peking University. 18 healthy New Zealand white rabbits weighting approximately 3.0 kg each were evenly divided into three groups: CACB group; Bio-Oss ® group; and untreated group. The surgical procedure for creating experimental mandible defects was performed according to a previously published protocol. The rabbits were anesthetized with pentobarbital sodium (1 mg/kg). After shaving the skin and disinfecting the surgical site in each animal, a parallel skin incision was made along the inferior border of the mandible on both sides. After exposing the masseter muscle, subperiosteal elevation of the muscle’s detachment was performed buccally and lingually, exposing the bone of the jaw angle. Under continuous saline irrigation, a slowly rotating trephine burr was used to create circular defects 8 mm in diameter in the region anterior to the jaw angles on every side of the mandible of each rabbit ( Fig. 2 ). A diameter of 5 mm in this experimental model has been reported to correspond to the critical size that prevents spontaneous healing during an animal’s lifetime. Each defect was flushed with saline to remove bone debris and then implanted with 0.25 g of either CACB or Bio-Oss ® granules. The experimental defect was left completely untreated (untreated group) as a control. Each treatment was randomly applied to each defect in a rabbit. The mucoperiosteal flaps were carefully sutured over the defect area using 3-0 absorbable sutures. After surgery, each rabbit received an intramuscular injection of sodium ampicillin at a dose of 20,000 U/kg every day for 3 successive days. Animals were killed by lethal intravenous administration of sodium pentobarbital at specific time points (4 and 12 weeks after the transplantation) for tissue processing.

Micro-CT analysis
12 weeks post-surgery, the animals were killed and their mandibles were explanted and fixed in 10% neutral buffered formalin. The mandible defect site was assessed by gross observation, and the morphology of the reconstructed mandibles was assessed using a micro-CT system (Skyscan 1076; Skyscan, Aartselaar, Belgium). The CT settings were: X-ray energy levels at 70 kV, current at 139 μA, and integration time of 600 ms with 300 projections per 180°. After scanning, the reconstructed data were segmented using a threshold algorithm as reported previously, and a three-dimensional (3D) histomorphometric analysis was performed automatically (three independent regions in each group). The parameters of bone mineral densities (BMD) and bone volume fraction (bone volume/total volume, BV/TV) were used for comparison in this study.
Histological evaluation
All the rabbit mandibles from the three groups after 4 and 12 weeks post-surgery were subjected to tissue processing and sectioning as reported previously. Briefly, the tissue samples were fixed in 10% neutral buffered formalin for 7 days, followed by decalcification and dehydration, and finally embedded in paraffin and sectioned at 5 μm thickness. Haematoxylin–eosin (H–E) and Masson’s trichrome staining were performed separately on consecutive tissue sections, and images were captured under microscopy (CX21, Olympus, Japan).
Statistical analysis
Quantitative data were presented as mean ± standard deviation (SD). Statistical differences between groups were evaluated by Student’s t -test using the software SPSS 13.0 (SPSS Science). A p -value < 0.05 was considered statistically significant.
Results
Physicochemical characterization of CACB
SEM images of the surface morphology and microstructure of CACB compared with Bio-Oss ® and calcinated human cancellous bone are shown in Fig. 3 . CACB exhibited a highly porous and well-interconnected pore structure. The pore size range of CACB was typically around 300–600 μm, which was in a similar scale to that of calcinated human cancellous bone (300–800 μm) and Bio-Oss ® (200–500 μm) ( Table 1 ). The volumetric porosity of CACB was 75 ± 2.23%, which was comparable to the corresponding values of 79 ± 3.14% and 71 ± 4.35% obtained for calcinated human cancellous bone and Bio-Oss ® , respectively. The higher magnification SEM imaging revealed that CACB possessed hexagonal apatite crystal morphology, which more closely resembled calcinated human cancellous bone, compared to Bio-Oss ® . The XRD spectrograph demonstrated that the characteristic peaks of CACB corresponded to the characteristic peaks of hydroxyapatite (HA) and that of calcinated human cancellous bone ( Fig. 4 A ). Additionally, CACB more closely resembled the crystal structure of calcinated human cancellous bone apatite minerals compared to Bio-Oss ® . The FT-IR spectrograph confirmed that the composition of CACB was mainly carbonated hydroxyapatite ( Fig. 2 B) with the Ca/P ratio of CACB being 1.81 ( Table 1 ). All these features were similar to those of calcinated human cancellous bone, suggesting that CACB would be a suitable matrix for bone regeneration.
Specimen | CACB | Bio-Oss ® | Calcinated human cancellous bone |
---|---|---|---|
Pore size (μm) | 300–600 | 200–500 | 300–800 |
Porosity (%) | 75 ± 2.23 | 71 ± 4.35 | 79 ± 3.14 |
Ca/P ratio | 1.81 | 1.47 | 1.76 |
Micro-CT measurement
To assess the 3D structure of the repaired mandible, micro-CT images were taken at 12 weeks post-surgery. In the CACB group, it was found that the boundary between the mandible defect site and surrounding host bone was indistinguishable ( Fig. 5 A ), which is similar to the Bio-Oss ® group ( Fig. 5 B). The regenerated bone tissue at the defect site was flushed with the surrounding host bone and the surface morphology of the healed mandible closely resembled that of normal mandible ( Fig. 5 D), suggesting that desirable repair of the defect area has been achieved. In the untreated group, the mandible defect site was left largely unrepaired and remained a conspicuous introcession ( Fig. 5 C).
To quantify calcification within the repaired mandible, local BMD of implants in the CACB and Bio-Oss ® group were assessed by micro-CT analysis at 12 weeks post-surgery. As shown in Fig. 6 A , the CACB group showed relatively high BMD of (541.10 ± 26.76) mg HA/cm 3 , {} which was similar to that of the Bio-Oss ® group (561.17 ± 22.30) mg HA/cm 3 and the normal mandible (594.34 ± 20.42) mg HA/cm 3 . By contrast, in the untreated group, the local BMD at the defect site without any implant was (213.50 ± 25.59) mg HA/cm 3 , which was significantly lower than that of the CACB group ( p < 0.05) and the Bio-Oss ® group ( p < 0.05). The ratio BV/TV, as an indicator of the relative amount of newly formed bone, was significantly higher for the CACB group when compared to the untreated group ( Fig. 6 B), but was similar for both the CACB and Bio-Oss ® groups. These results confirmed that both the CACB and Bio-Oss ® scaffolds displayed similar levels of osteogenesis upon implantation.
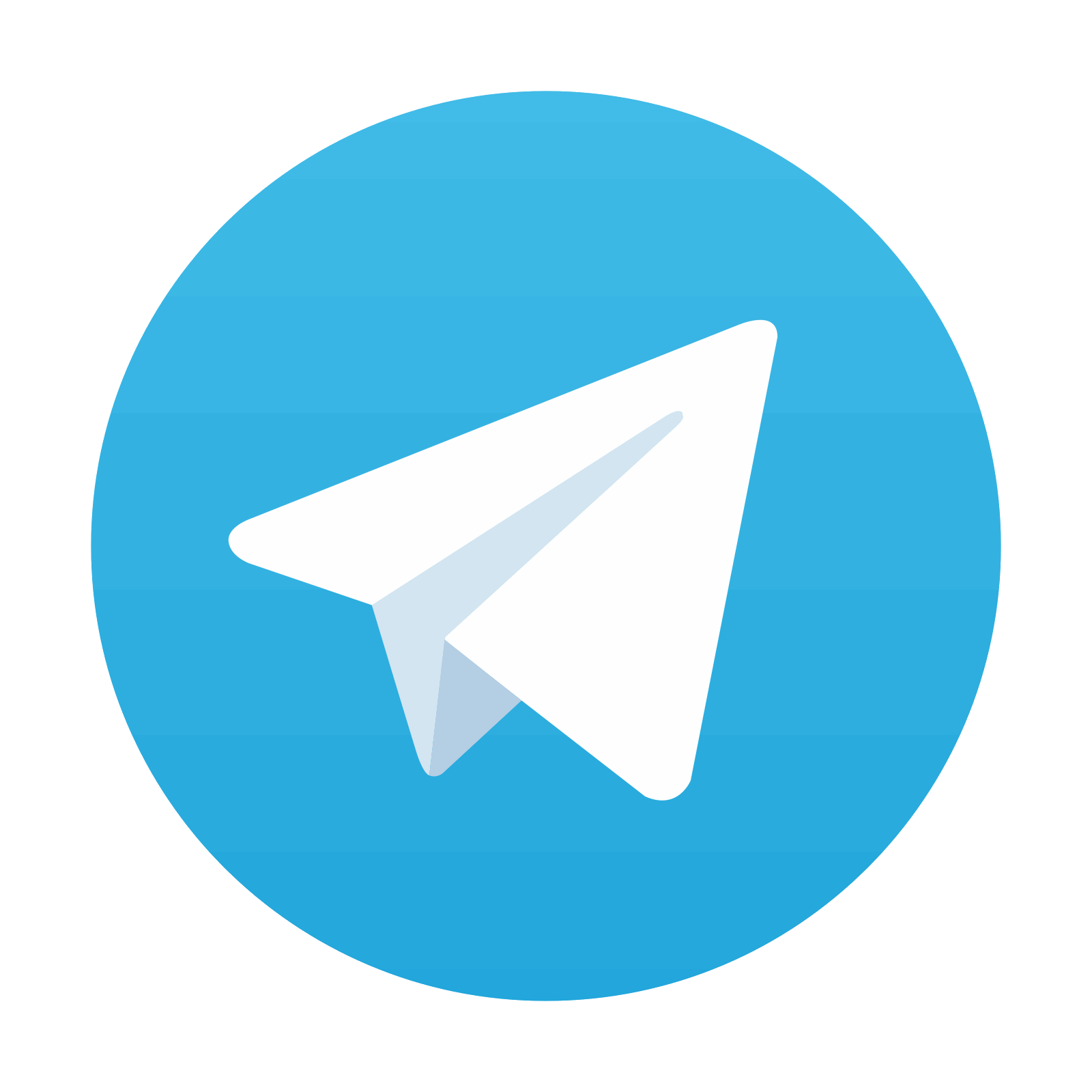
Stay updated, free dental videos. Join our Telegram channel

VIDEdental - Online dental courses
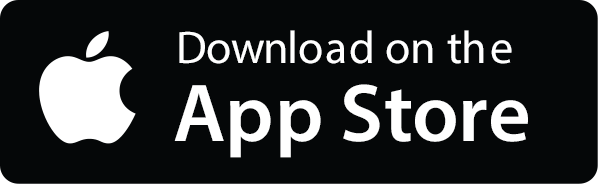
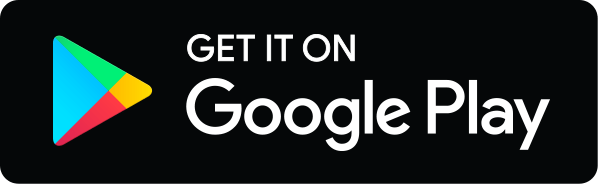
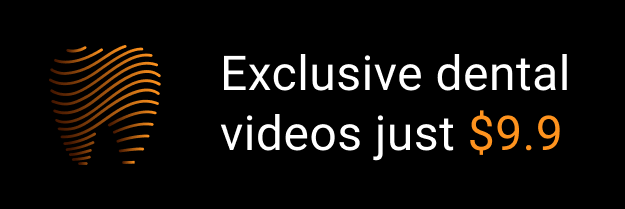