Highlights
- •
Wear tests were conducted on a multilayer 5Y/4Y-PSZ and a monolithic 3Y-TZP.
- •
Wear volume loss and depth were greater in 5Y/4Y-PSZ than in 3Y-TZP.
- •
Worn surface was more rugged in 5Y/4Y-PSZ than in 3Y-TZP.
- •
The rough surface was due to extensive lateral cracking, leading to spalling.
- •
The presence of different layers had no effect on the wear behavior of 5Y/4Y-PSZ.
Abstract
Objective
To characterize the composition, microstructure and wear properties of a multilayer translucent zirconia relative to the conventional 3Y-TZP.
Methods
Two types of ceramics were evaluated: a multilayer zirconia (MULTI, IPS e.max ZirCAD Multi, Ivoclar Vivadent) and a control 3Y-TZP (IPS e.max ZirCAD LT, Ivoclar Vivadent). Pre-sintered CAD-CAM blocks were cut, ground, sintered and polished to 1 μm finish. The phase fraction and grain size were measured using XRD and FE-SEM. For wear testing ( n = 12), square-shaped specimens (16 × 16 × 1 mm) were adhesively bonded to a dentin analog. Sliding wear tests were performed using a spherical zirconia antagonist ( r = 3.15 mm), with 30 N load at 1.5 Hz for 500,000 cycles in water. Optical and scanning electron microscopes and 3D laser scanner were used for quantitative wear analyses. Data were analyzed using Student’s t -test ( α = 0.05).
Results
For MULTI, the enamel layer had the highest cubic content and the largest grain size, followed by the two transition layers, and the dentin layer. 3Y-TZP showed the smallest grain size and cubic content. A significant amount of wear was observed in both materials up to 50,000 cycles until it reached a plateau. MULTI showed higher volume loss and greater wear depth than 3Y-TZP (p < 0.01). The higher volume loss was associated with extensive lateral fracture, leading to material spalling from the surface of cubic-containing zirconias.
Significance
The wear pattern in multi-layered zirconia was more severe than 3Y-TZP. Additionally, the different layers of the multi-layered zirconia had similar wear behavior.
1
Introduction
Dental materials must combine good physical and mechanical properties with tooth-like esthetics in order to provide restorative treatments with high survival rates and to guarantee the patient´s satisfaction. The composition and microstructure of the first-generation zirconia, specifically 3 mol.% yttria stabilized tetragonal zirconia polycrystals (3Y-TZP), were tailored to optimize its strength and toughness rather than translucency. Thus, they are suitable for the frameworks of crowns and bridges, as well as implants and abutments [ ]. The improved translucency in the second-generation zirconia was achieved by reducing the content of alumina sintering additives while increasing its bulk density [ , ]. Such an effort ultimately expanded the clinical indication of zirconia to monolithic posterior crowns and bridges [ ]. Further improvement of translucency of the third-generation zirconia was realized by increasing the quantity of yttria stabilizer, leading to higher amounts of optically isotropic cubic phase content [ , ]. The tradeoff is that these highly translucent, cubic-containing zirconias also exhibited lower strength and toughness relative to the first and second generations of 3Y-TZP [ ]. Therefore, they are clinically indicated for veneers, inlays, onlays, crowns, and anterior bridges up to 3 units [ , ].
Despite much improved translucency, the first 3 generations of zirconia are monochrome. In order to produce a restoration with a more natural appearance, the fourth-generation, multichromatic zirconia with a layered structure have been developed to mimic the shade and translucency gradients found in natural teeth [ , ]. In that first multilayer zirconia system introduced to restorative dentistry (Katana, Kuraray Noritake), the same yttrium content and cubic fraction were observed in the different layers of the materials. The only difference among those layers was the pigment composition, which led to differences in shade, but not in translucency [ ]. More recently, a different strategy has been adopted, in which different compositions and microstructures can be found in the same material (IPS e.max ZirCAD Multi, Ivoclar Vivadent). According to the manufacturer, this material has four distinct layers: the incisal one-third (enamel) contains a 5 mol.% yttria partially stabilized zirconia (5Y-PSZ); the body layer (dentin), which is the gingival counterpart, has a 4 mol.% partially stabilized zirconia (4Y-PSZ); and the composition of the two intermediate layers that integrate the enamel and body layers is unknown. As a result, the unique property of a gradual progression of shade and translucency permits the use of this material for a broad spectrum of clinical indications, including anterior veneers with high esthetic demands.
Nevertheless, the effect of the composition and microstructure gradients on the mechanical behavior of these new multi-layered materials has not been investigated. The literature shows that 4Y-PSZ and 5Y-PSZ have lower fracture strength and toughness than the conventional 3Y-TZP, due mainly to the reduced ability of transformation toughening associated with a decrease in tetragonal zirconia content [ , , , ]. Additionally, as 5Y-PSZ has higher cubic content than 4Y-PSZ, their mechanical properties also differ from each other [ , , ].
Clinically, restorations are exposed to a hostile humid environment with varying pH levels and temperatures. Chewing and parafunctional habits can induce both axial and off-axial forces to the restoration [ , ]. During the mandibular movements, the antagonist slides over the restoration surface across different layers of the multi-layered zirconia, where its composition, microstructure and wear behavior is still unclear. The wear produced by the contact with the antagonist is classified as attrition (two-body wear) and could result in surface damage, with crack initiation and propagation, and loss of material, leading to failures [ , ]. Although zirconia-based materials are more wear resistant than the tooth enamel, extensive prosthetic rehabilitation has become very common in dentistry and other wear-resistant restorative materials may also be found as the antagonist [ ].
Literature on the wear behavior of the third- and fourth-generations, cubic-containing zirconia is limited; furthermore, none of the studies focused on the multi-layered material with compositional and microstructural gradients [ ]. In addition, only a few clinical reports on the multi-layered zirconia restorations are available [ ]. Therefore, it is important to understand the structure and wear behavior of the new multilayer zirconia in order to support its clinical indications. Accordingly, the objectives of this study are to characterize the composition, microstructure and wear properties of a multilayer translucent zirconia dental ceramic (MULTI, IPS e.max ZirCAD Multi, Ivoclar Vivadent) in relation to its multi-transition zones as well as to compare its properties with a conventional monolithic 3Y-TZP ceramic (IPS e.max ZirCAD LT, Ivoclar Vivadent).
2
Materials and methods
A multilayer translucent zirconia ceramic (MULTI, IPS e.max ZirCAD Multi, Ivoclar Vivadent) and a conventional 3Y-TZP (3Y, IPS e.max ZirCAD LT, Ivoclar Vivadent) control were investigated. Material description can be found in Table 1 .
Legend | Material | Mean Grain Size (standard-deviation) b | Cubic Content b | Mean Flexural Strength (standard-deviation) b |
Fracture Toughness a | Mean Translucency Parameter (standard-deviation) b | Clinical Indication a |
---|---|---|---|---|---|---|---|
MULTI | IPS e.max ZirCAD Multi (Ivoclar Vivadent) | Enamel layer: 1.87 (0.35) μm | Enamel layer: 80 wt.% | Enamel layer: 387 (60) MPa | 3.6 MPa m 1/2 | Enamel layer: 36.7 (1.7) | Full-contour crowns, full-contour 3-unit bridges, implant-supported superstructures |
T1: 1.72 (0.32) μm | T1: 64 wt.% | ||||||
T2: 1.40 (0.15) μm | T2: 49 wt.% | ||||||
Dentin Layer: 1.12 (0.21) μm | Dentin Layer: 46 wt.% | Dentin layer: 744 (104) MPa | Dentin layer: 29.0 (0.7) | ||||
3Y | IPS e.max ZirCAD LT (Ivoclar Vivadent) | 1.08 (0.23) μm | 15 wt.% | 851 (97) MPa | 5.0 MPa m 1/2 | 26.3 (0.0) | Full-contour crowns, full-contour 3- and 4-unit to multi-unit bridges, frameworks, implant-supported superstructure |
2.1
Composition and microstructure
2.1.1
Zirconia phase quantification
MULTI blocks were cut into three parts to obtain samples of each layer using a low speed diamond saw (Isomet 1000, Buehler, USA). For enamel and dentin layers, specimens were cut from the top and bottom of the block. For transition layers, the middle part of the block was used; the surface near the enamel layer was designated as transition zone 1 (T1) and the surface near the dentin layer, transition zone 2 (T2). The material was sectioned according to a careful microscopic analysis of the location of each layer (layer thickness). A specimen from 3Y control group was also obtained by cutting the ceramic block. All specimens were ground using silicon carbide paper with 320-grit and 800-grit in order to obtain flat surfaces. The same sintering protocol (1500 °C for 2 h) was performed for both materials, following the manufacturer instructions.
In order to identify the crystalline phase fractions, specimens were analyzed by X-ray diffraction (XRD, X’Pert3 Powder, PANalytical, Netherlands). Scanning conditions were set as follows: the tube voltage 40 kV, tube current 45 mA, with CuKα radiation ( λ =1.5418 Å) using a step-scanning technique with a fixed step size of 0.01° and a rate of 0.3 s/step, over a 2 θ range between 20° and 80°. A full-profile fitting technique was used to precisely calculate the phase fractions of each material by the Rietveld refinement method implemented in the HighScore Plus software [ ]. The diffractograms were used to quantify phases of zirconia by focusing on tetragonal (002 and 200) and cubic (111) peaks around 2 θ = 35°.
2.1.2
Microstructural analysis
To analyze the microstructure and measure the grain size, specimens from each layer of MULTI and 3Y were produced ( n = 2), as described above for the XRD analysis. After sintering, one surface of the zirconia specimen was further polished to 1 μm finish using a polishing machine (Ecomet Polisher, Buehler, USA) to obtain a final dimension of 12.8 × 12.8 × 1 mm. Specimens were then thermally etched at 1150 °C for 20 min with heating and cooling rates of 10 °C per min.
After cooling, the specimens were ultrasonically cleaned in ethanol for 5 min. Prior to imaging in a Field Emission Scanning Electron Microscope (FE-SEM, Merlin, Carl Zeiss), specimens were coated with a nanometric layer of irridium. The grain size was measured on SEM micrographs using the linear intercept technique [ ]. The average grain size ( D ) was obtained using <SPAN role=presentation tabIndex=0 id=MathJax-Element-1-Frame class=MathJax style="POSITION: relative" data-mathml='=1.56CMN’>=1.56???=1.56CMN
= 1.56 C M N
, where C is the total length of test line used, N the number of intercepts and M the magnification of the photomicrograph. The proportional constant, 1.56, is essential for random slices through a model system consisting of space-filling tetrakaidekahedral shaped grains with a lognormal size distribution.
2.2
Wear characteristics
2.2.1
Specimen preparation
MULTI and 3Y ceramics were evaluated in the oral wear simulator ( n = 12). Specimens of MULTI and 3Y were produced and polished to 1 μm finish, as described for the microstructure analysis.
Zirconia specimens were cemented to a dentin analog substrate (G10, glass-fiber reinforced epoxy resin rods, Acculam, USA) with a dual-cure resin cement. G10 cementation surface was acid-etched with 5% hydrofluoric acid (Ceramic Etching Gel, Ivoclar Vivadent) for 2 min, while the ceramic surface was air-abraded with 50 μm alumina particles at 3 bars pressure for 20 s. After cleaning the G10 and ceramic surfaces, a bonding agent (Monobond Plus, Ivoclar Vivadent) was applied for 60 s and air dried. Ceramic specimens were then cemented to the dentin analog using Multilink Automix dual-cure resin cement (Ivoclar Vivadent). A 9.8 N load was kept over the ceramic specimen for 5 min to ensure a uniform cement layer and specimens were light cured for 30 s on each side using a dental curing light (Bluephase Style, Ivoclar Vivadent). The bonded specimens were kept in distilled water at 37 °C for 3 days in an incubator (Isotemp Incubator, Fisher Scientific, USA) prior to testing in the oral wear simulator.
2.2.2
Wear tests
A 4-chamber Oregon Health Science University (OHSU) oral wear simulator (Proto-tech, USA) was used in this study. The test was performed as follows: (1) a 30 N vertical load was applied through a zirconia spherical antagonist ( r = 3.15 mm) to the ceramic surface, (2) the antagonist advances across the specimen surface in a 5-mm horizontal path, (3) the load level decreases at the end of the path as the antagonist lifts out of contact while returning to its original position, to begin a new cycle.
Careful examinations of sintered MULTI specimens by optical microscopy revealed that the thicknesses of the enamel, T1, T2, and dentin layers are 2.56, 1.15, 1.40, 7.68 mm, respectively ( Fig. 1 ). To ensure the antagonist has traversed all four layers, the 5-mm cyclic sliding action began with positioning the antagonist 2 mm away from the surface of the enamel layer (5Y), sliding across the 2 transition layers (T1 and T2), and ending in the dentin layer (4Y) approximately 1.89 mm away from the T2/dentin interface ( Fig. 1 ).
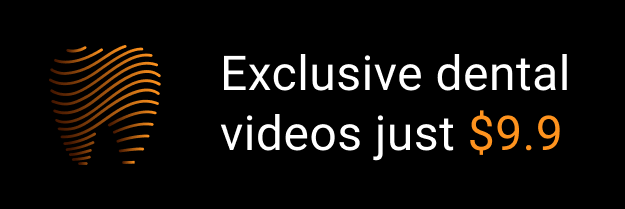