Highlights
- •
Monolithic bridges from three zirconia grades were subjected to aging/loading.
- •
Aging and loading did not affect the fracture load of translucent zirconia bridges.
- •
Transformability correlated with fracture load.
- •
5Y-PSZ might not be suitable for restorations thinning towards the margin.
Abstract
Objective
To investigate the fracture resistance and phase composition of tooth supported four-unit fixed dental prostheses (FDPs) made from three different zirconia grades after loading and aging.
Methods
Seventy-two FDPs were fabricated from 3Y-TZP, 4Y-PSZ and 5Y-PSZ. This resulted in 24 FDPs per grade, subdivided into three groups (n = 8): a control group (C), a hydrothermally aged (H 2 O, 85 °C, 90 days) group (A) and a group subjected to loading (2.5M cycles, 98N) with simultaneous thermal cycling (H 2 O, 5–55 °C) subsequent to treatment A (AL). Subsequently, FDPs were statically loaded to fracture. Phase composition was quantified by X-ray diffraction (XRD) and μ-Raman spectroscopy. Focused ion beam (FIB) – Scanning electron microscopy (SEM) was used for visualization in-depth.
Results
Compared to 3Y-C FDPs (1233 ± 165N), reduced fracture load was found for 5Y-C FDPs (889 ± 80 N; p < .001). This did not apply for 4Y-C samples (1065 ± 111N). Treatments (A, AL) did not negatively affect the fracture load for the three grades of zirconia (p > .645). Both A and AL increased monoclinic phase content for 3Y and 4Y FDPs, whereas FIB-SEM suggests no transformability of 5Y-PSZ. 5Y-AL FDPs showed cracks and fractures at the abutment walls and restoration margins after dynamic loading. Reduced fracture load of 5Y samples as compared to 3Y and 4Y was associated with deficient transformability in the fracture zone.
Significance
Aging and loading did not negatively affect the fracture resistance of monolithic four-unit FDPs made from three grades of zirconia. Due to cracks after dynamic loading, 5Y-PSZ cannot be recommended for the clinical application of four-unit FDPs.
1
Introduction
High strength 3 mol% yttria stabilized tetragonal zirconia polycrystal (3Y-TZP) dental zirconia was developed for a computer aided design/computer aided manufacturing (CAD/CAM) fabrication of longer span posterior restorations or even complete dental dentures [ ]. 3Y-TZP being almost opaque, the translucency of actual 4- and 5Y- partially stabilized zirconia (PSZ) zirconia was improved, increasing the indication of zirconia to the aesthetic anterior region [ ]. Nevertheless, with developed translucency, strength is dramatically reduced. This is a reason why different types of zirconia provide strength values between approx. 600–1200 MPa and different indication opportunities [ ].
Zirconia is a polymorphic material, which is present in three different phases: monoclinic (m), tetragonal (t) and cubic (c) [ ]. Transformations between the phases take place in certain temperature ranges (athermal). The martensitic phase transformations are diffusion-free, take place without rearrangement of atoms and are reversible. They are characterized by shifts in the positions of the crystal lattices. The increase in volume during cooling (t->m) is the reason why pure zirconia can only be produced with great effort (e.g. with highly reactive monoclinic powders). During cooling, the expansion leads to stresses and cracks in the structure. However, the t->m transformation can be prevented by doping with oxides. The corresponding structure is thus (meta-)stabilized at room temperature in the tetragonal and/or cubic state [ , ]. The ions used for stabilization must have a suitable atomic radius and a corresponding solubility in the solid solution lattice to stabilize the tetragonal or cubic phase [ ]. The doping of dental systems is usually done with Y 2 O 3 . The quantity of added Y 2 O 3 can be used to determine the phase content (tetragonal, cubic), but also the size of the grains and thus some properties of the material (e.g. strength, translucency, coefficient of thermal expansion, etc) [ ]. The tetragonal phase is metastable, that means that spontaneous conversion of t->m takes place. Various parameters such as temperature or particle size also influence this t->m conversion. Principally the main zirconia phase is decisive for the properties of individual zirconia systems [ , ]. Phase structure and grain boundaries can be examined using X-ray diffraction with Rietveld analysis and scanning electron microscopy, scanning transmission electron microscopy and energy-dispersive spectroscopy (STEM-EDS) [ ]. A typical 3Y-TZP consists of approx. 85% tetragonal and 15% cubic phase [ ]. In contrast, 5Y stabilized zirconia consists of about 50% cubic phase [ ]. This type of zirconia may also be referred to as 5Y-PSZ (partly stabilized zirconia, occasionally/ not correctly also as cubic zirconia).
The t->m transformation can be triggered by stress [ ]. Causes can be application-related, e.g. cracks or abrasion during mastication or defects caused by manufacturing (blasting, grinding). If a crack occurs, the material at the crack tip can change from tetragonal to monoclinic (domain orientation/ferro-elastic domain change)-a volume expansion (pseudo-elastic) takes place and the crack is compressed/stopped [ ]. Therefore, Y-TZP with tetragonal phase have a higher fracture toughness. This strengthening mechanism is reduced in partly stabilized zirconia PSZ with higher cubic content.
Tetragonal zirconia is thermally relatively unstable between 200 °C and 400 °C. However, the chemical resistance is also reduced below 100 °C, especially in acidic or alkaline surrounding. This hydrothermal degradation (low temperature degradation) is associated with a spontaneous phase transformation, the removal of grains, microcracks and other corrosion phenomena, which may particularly intensified by mechanical stress (e.g. chewing forces). One released grain (triggered by non-uniform and too large grains, too low a proportion of Y 2 O 3 , the presence of purely cubic grains or also by internal stresses) may trigger a cascade and affect other grains. These ageing effects are supported, for example, by impurities, an irregular distribution of the doping oxides or by applying incorrect sintering temperatures. Current data show that PSZ is unlikely to undergo such aging. However, increasing the aging resistance of zirconia by increasing the yttria content normally reduces the crack resistance [ ].
Based on these considerations, the question arises whether 4/5 Y-PSZ zirconia with lower mechanical properties than 3Y-TZP might be sufficient for longer span restorations even after aging. In-vitro tests conducted with simple-shaped and standardized objects (discs, bars) lacking the complex geometrical design and topography of a dental reconstruction are currently available in the literature and supposed to improve our knowledge of isolated aspects, whereas superimposed effects may be investigated in simulation tests on anatomically shaped dental restorations considering the complex geometrical design and topography of teeth to be restored or replaced. To the present, such laboratory data, subjecting ready to be delivered dental reconstructions to aging and loading conditions closely approximating clinical reality, is not available for multi-unit fixed dental prostheses made from different and market-available zirconia grades. Available studies are addressing the connector design and embrasure shaping method [ ], evaluating the stress distribution using finite element analysis [ ], marginal and internal fit [ ], optical characteristics [ ], the effect of speed sintering [ ], or mechanical properties of implant supported anterior FDPs [ ]. Of these studies, only a single one investigated the fracture resistance of anatomically shaped tooth-supported FDPs, however not dynamically loading or hydrothermally aging the samples [ ]. In-vitro storage (e.g. water storage at evaluated temperatures) and/or clinically approximated thermal and mechanical simulation (TCML) may allow for comparative evaluation of the different types of zirconia restorations [ ]. Therefore, the hypothesis of this in-vitro study was that restorations made of different type of zirconia (3Y-TZP and 4/5Y-PSZ) will show (1) different phase composition after manufacturing, (2) different stress and environmentally induced transformability due to the applied treatments, (3) comparable wear behavior, and (4) that fracture load will be affected (strength reduction) by mechanical loading subsequent to aging.
2
Materials and methods
2.1
Sequence of experimental procedures
Twenty-four maxillary four-unit FDPs were fabricated from three zirconia grades with varying yttria content ranging from 3 to 5 mol% (3Y-TZP, 4Y-PSZ, 5Y-PSZ). This resulted in a total of 72 FDPs ( Fig. 1 ). Each zirconia grade (3Y, 4Y, 5Y) was subdivided into three groups of 8 samples to be exposed to different treatments. In the control group (C), samples were stored in H 2 O set at 37 °C for 24 h, whereas samples of the aging group (A) were exposed to H 2 O set at 80 °C for 90 days. Samples of the last group (aging/loading, AL) were subjected to dynamic loading in the artificial mouth subsequent to the aging procedure.

2.2
Sample preparation
2.2.1
Design and manufacturing
A maxillary four-unit FDP revealing terminal pillars (first premolar and second molar) and two adjacent pontics (second premolar and first molar), the corresponding abutment teeth and the embedding sockets were computer-aided designed (exocad; exocad, Darmstadt, Germany) and manufactured ( Fig. 2 ) in order to mimic intraoral conditions to the extent possible. The connector size measured 22.6 (in between the molars), 16.1 (in between the second premolar and the first molar) and 19.2 mm 2 (in between the premolars), respectively. Seventy-two FDPs were fabricated from 3Y-TZP (n = 24; priti multidisc ZrO 2 “translucent”; Pritidenta, Leinfelden-Echterdingen, Germany), 4Y-PSZ (n = 24; “extra translucent”; Pritidenta) and 5Y-PSZ (n = 24; “high translucent”; Pritidenta), respectively. Manufacturing (CORiTEC 350i; imes-icore, Eiterfeld, Germany) and sintering (HT Speed, 2 h holding time at 1450 °C; MIHM-VOGT, Stutensee-Blankenloch, Germany) followed the manufacturer’s instructions. After final sintering, samples were polished to a clinically satisfying standard. Standardized abutment teeth (first premolar, n = 72; second molar, n = 72) were grinded from Polymethyl methacrylate (CORiTEC Disc PMMA; imes-icore), whereas the embedding socket was printed (n = 72; Form 2; Formlabs, Somerville, USA), likewise from PMMA (Standard resin; Formlabs).

2.2.2
Embedding
Subsequent to the aging procedure (see below), FDPs were prepared according to the manufacturer’s instructions (air particle abraded, aluminum oxide, 50 μm, 1 bar) and adhesively luted to the artificial abutment teeth (Panavia F2.0; Kuraray Noritake, Tokyo, Japan). Thereafter, FDPs with attached teeth were embedded to the printed sockets with a polyether material (Permadyne Garant; 3M Espe, Neuss, Germany) in order to simulate the periodontal ligament. Finally, the inner occlusal surface of the loaded unit (first molar) was polished according to the manufacturer’s instructions prior to dynamic and static loading in the dental laboratory. No glazing or additional firing was applied.
2.3
Treatments
2.3.1
Control group (C)
Control group samples (n = 24) were stored in H 2 O set at 37 °C for 24 h prior to static loading to fracture.
2.3.2
Aging group (A)
FDPs to be aged (n = 48) were stored in H 2 O set at 80 °C for 90 days prior to cementation in order to simulate a long-term intraoral exposition. Assuming an actication energy of 107 kJ·mol −1 for the aging reaction of 3Y-TZP [ ], this might roughly correspond to three to four decades. After cementation and embedding, half of these samples (n = 24) was statically loaded to fracture.
2.3.3
Aging/loading group (AL)
Half of the aged samples (n = 24) were dynamically loaded in the artificial mouth (CS-4.8, SD Mechatronik; Feldkirchen-Westerham, Germany). The 2.5 million loading cycles consisted of the loading force (10 kg, 98N) being vertically applied (60 mm/s) to the mesio-palatal cusp of the first premolar and a subsequent horizontal movement (1 mm, 30 mm/s) under load reaching both buccal cusps. This setup resulted in a chewing frequency of 2 Hz. During the entire loading period of 15 days, samples were stored in a thermocycling environment (H 2 O, 5/55 °C, 36.000 cycles consisting of 30 s exposure and 6 s drain time).
2.4
Measurements and analyses
2.4.1
Static loading
All FDPs of the C/A groups and FDPs of the AL group that survived the dynamic loading procedure were subjected to quasi-static loading to fracture using a universal testing machine (Zwick, Z010, v = 1 mm/min; Ulm, Germany). Analogously to chewing simulation, the load was occlusally applied with a steel sphere (d = 12 mm) in the center of the first molar. A tin foil (0.25 mm; Dentaurum, Germany) between occlusal surface and sphere was inserted to prevent force peaks.
2.4.2
Fractography
Fracture pattern was investigated in detail using scanning electron microscopy (SEM Quanta; Low vaccum, Sweden; working distance [Wd] ∼52 mm, Philips, The Netherlands) at magnifications between 20×–4000×.
2.4.3
Wear analyses
FDPs and steatite antagonists were digitized using a model scanner (D2000; 3shape, Copenhagen, Denmark) and exported as standard tessellation language (STL) format to an inspection software (Geomagic Control X; 3D-Systems, Rock Hill, South Carolina). For the local-Best fit superimposition, only regions beside areas of contact were included. Afterwards, wear was determined by means of maximum intrusion depth (mm), surface area (mm 2 ) and worn volume (mm 3 ).
2.4.4
X-ray diffraction (XRD)
Phase transformations on the outer surface of all FDPs were examined by X-ray diffraction (XRD; 3003-TT, Seifert, Ahrensburg, Germany). The XRD patterns were collected using Cu-Kα radiation at 40 kV and 40 mA from 20 to 90° (2θ) with a step size of 0.02 for 2 s. The monoclinic zirconia phase ( m -ZrO 2 ) content was calculated according to the formula of Garvie et al. [ ] that was modified by Toraya et al. [ ].
2.4.5
μ-Raman spectroscopy
μ-Raman (Senterra, BrukerOptik; Ettlingen, Germany) was complementarily used to characterize the phase composition of different positions at loaded area of mesio-palatal cusp, non-loaded area of mesio-palatal cusp, mesial restoration margin of the first premolar and fracture zone ( Fig. 3 ). μ-Raman spectra were collected using a 532 nm wavelength Ar-ion laser source of 20 mW through a 50× objective with a pinhole aperture of 25 μm in three successive measurements of 20 s integration time per measurement. Due to the geometry of the FDPs especially for reaching occlusal surface and the limited working distance with higher magnification, 50× was the largest magnification can be used, which gave rise to lateral resolution of about 2 μm [ , ]. At least 25 spots were assessed for each investigated position of the FDPs. The μ-Raman monoclinic zirconia fraction ( V m ) was calculated according to the formula of Tabares et al. [ ].

2.4.6
Focused ion beam (FIB)-scanning electron microscopy (SEM) FIB-SEM
The subsurface microstructure at the thin walls surrounding the abutment teeth of 5Y-AL was examined by FIB-SEM (Nova NanoLab 600 DualBeam, FEI, Eindhoven, The Netherlands). Cracked thin wall was glued with silver paint onto an aluminium stub and were coated with a platinum layer of about 20 nm. An in-situ ion beam-induced Pt deposition was performed over the areas of interest to minimize the curtain effect and to protect the sample surface from implantation. Coarse milling of FIB sections in the size of about 30 μm width and 30 μm depth was performed at 30 kV ion accelerating voltage and 6.5 nA beam currents. Fine milling and cleaning were performed at 30 kV ion accelerating voltage and decreased beam currents from 2.7 nA, 0.9 nA, 0.44 nA till 0.26 nA in sequence to reach a clean cross-section for SEM imaging and to avoid any ion-beam induced transformation. SEM imaging was performed at 3 kV simultaneously with Secondary Electron (SE) and Energy filtered Backscattered Electron (BSE) imaging.
2.5
Statistical analyses
Shapiro–Wilk-test and Levene–test were performed to verify normal distribution and variance homogeneity. Consequently, static loading was analyzed with univariate ANOVA for both variables, zirconia grade (3Y, 4Y, 5Y) and treatment (C, A, AL). In case of significant results, post-hoc pairwise Tukey–HSD tests were applied. Monoclinic content was evaluated with non-parametric Kruskal–Wallis-H-tests followed by post-hoc Dunn’s tests comparing same locations per zirconia grade and per treatment. All data sets were included twice for analysis. Therefore, significance level was set at p < .05/2 = .025 according to Bonferroni correction. Wear was solely described descriptively, due to limited sample size. The statistical analysis was performed with SPSS 22.0 (SPSS; Chicago, IL, USA).
3
Results
3.1
Loading procedures
3.1.1
Dynamic loading
AL samples of all grades (n = 24) survived the dynamic loading procedure (i.e., no fracture in the connector area occurred). However, all 5Y-AL samples (n = 8) showed cracks and fractures at the walls surrounding the abutment teeth ( Fig. 4 ) on the mesial aspect of the anterior abutment (first premolar) and on the buccal aspect of the posterior abutment (second molar).

3.1.2
Static loading
3Y samples fractured at loads of 1233 ± 165 N (C), 1174 ± 69 N (A) and 1240 ± 78 N (AL), respectively ( Table 1 , Fig. 5 ). Treatments (A, AL) did not affect the fracture load (p = .645). Referring to the treatment modalities (C, A, AL), 4Y samples did not show reduced fracture loads compared to 3Y. However, within 4Y samples, significantly increase fracture load was measured for the group 4Y-A (p = .007). 5Y-C samples fractured at a load of 889 ± 80 N. Treatments (A, AL) did not affect the fracture load (p = .735). In comparison to 3Y and 4Y, fracture load of 5Y samples was statistically significant lower (p < 0.001).
Grade / Treatment | Control (C) | Aged (A) | Aged/Loaded (AL) | Significance per treatment |
---|---|---|---|---|
3Y | 1233 ± 165 A | 1174 ± 69 C | 1240 ± 78 D | .645 |
4Y | 1065 ± 111 A, B, 1 | 1306 ± 173 C | 1115 ± 208 D, 1 | .007 |
5Y | 889 ± 80 B | 909 ± 257 | 849 ± 139 | .735 |
Significance per grade | .000 | .000 | .000 |
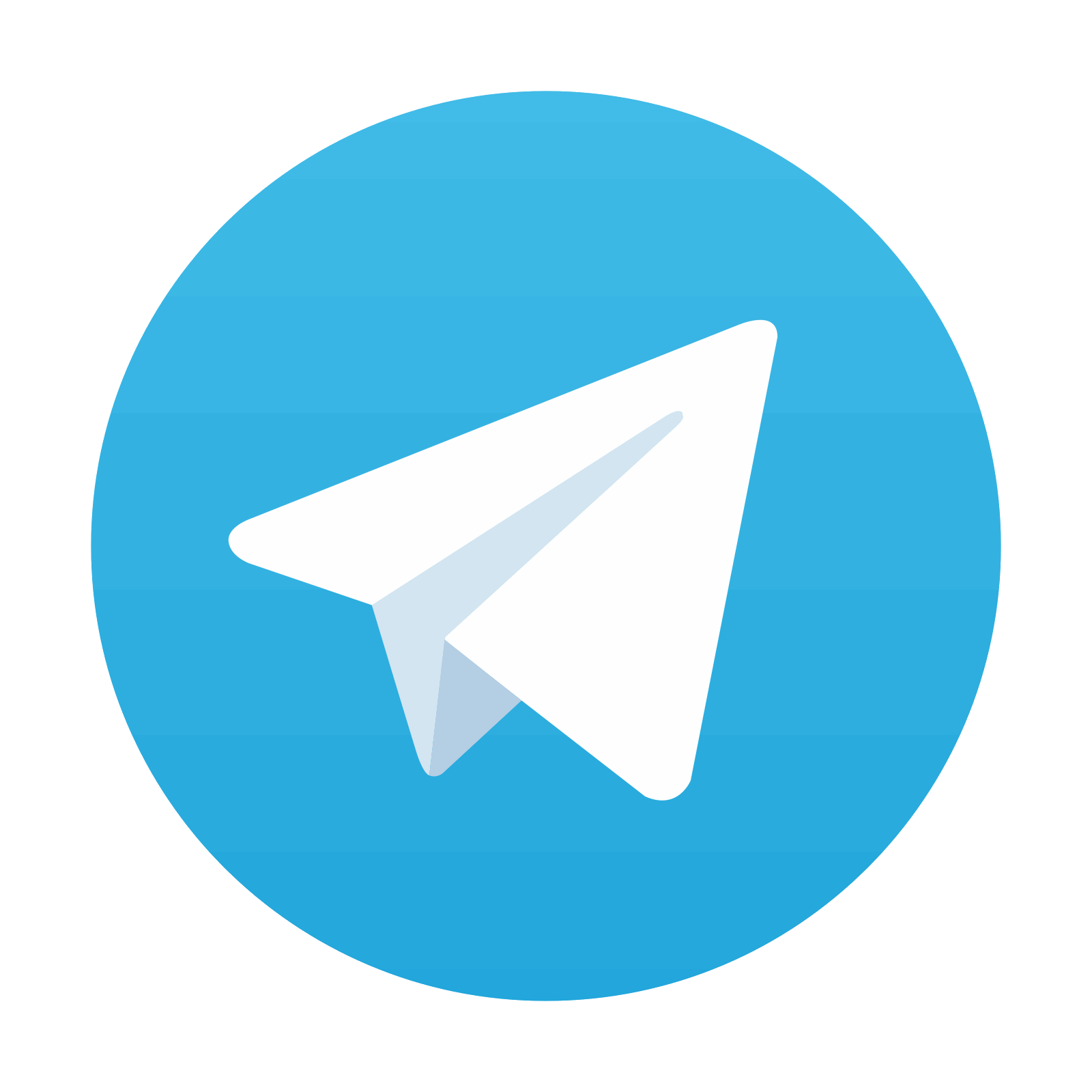
Stay updated, free dental videos. Join our Telegram channel

VIDEdental - Online dental courses
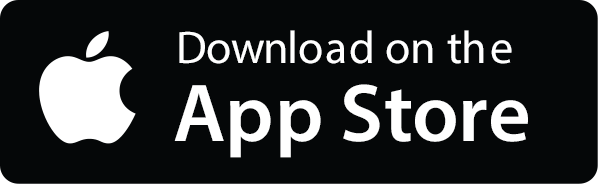
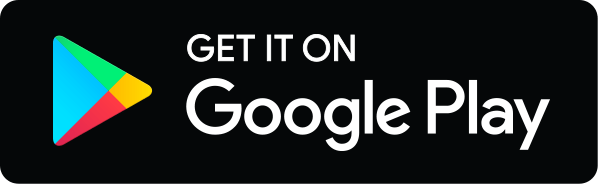
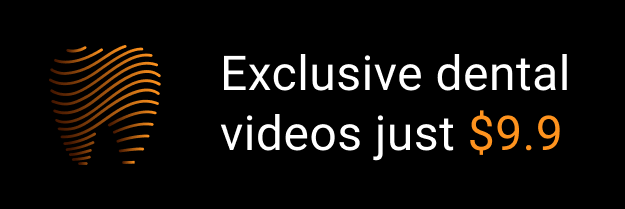