Abstract
Objective
Polymerization shrinkage developed in vertical and horizontal directions after light activation of light-curing composite restorative materials. The purpose of this study was to examine the effects of vertical and horizontal polymerization shrinkage on: (a) dimensional changes of resin composites in tooth cavities; (b) shear bond strengths to enamel and dentin; and (c) marginal gap width in a non-reacting Teflon mold.
Methods
Vertical and horizontal polymerization shrinkage in tooth cavities were measured immediately (3 min) after light activation. With the same time lapse, shear bond strengths to enamel and dentin and marginal gap widths in Teflon mold were also measured.
Results
There was a significant correlation between vertical and horizontal polymerization shrinkage ( r = 0.647, p = 0.043) in the tooth cavity. Composite materials which produced small vertical shrinkage also produced smaller horizontal shrinkage. Composite materials which produced small vertical shrinkage in the tooth cavity exhibited greater shear bond strengths to both enamel ( r = −0.697, p = 0.025) and dentin ( r = −0.752, p = 0.012). Composite materials which produced smaller horizontal shrinkage produced smaller marginal gap widths in the Teflon mold ( r = 0.829, p = 0.003). No relationships were observed between horizontal shrinkage in the tooth cavity and shear bond strengths to both enamel and dentin ( p > 0.05).
Significance
During the early stage of setting (<3 min) in tooth cavities, the vertical shrinkage of light-activated composite restorative materials was correlated with horizontal shrinkage.
1
Introduction
Polymerization of light-activated resin composites in cavity preparations is accompanied by volumetric shrinkage. Most resin composites shrink by about 1–4%, leading to a competition between polymerization contraction forces and the strength of bonds to tooth structure. As adhesive bonds were put under tension, tensile and/or shear stresses develop at the tooth-restoration interface. When polymerization shrinkage stress exceeds the bond strength to the cavity walls, it causes contraction gaps to be formed at the tooth-restoration interface . The onset of marginal gap formation then leads to microleakage , marginal discoloration, postoperative sensitivity, secondary caries development , cuspal strain and displacement in composite-restored teeth , cohesive failure within the tooth structure or resin composite, or immediate adhesive failure at tooth-composite interface . Eventually, this slew of problems associated with polymerization shrinkage will culminate in the clinical failure of the composite restoration .
Many factors influence the magnitude of stress generated during the polymerization of resin composites. These factors include the elastic modulus and flow capability of the resin composite, curing method, filling technique of resin composite restoration, bond strength of resin composite to the cavity walls, and the cavity configuration factor (C-factor) of the bonded restoration. The C-factor describes the ratio of the bonded surface area to the unbonded surface area of a composite restoration . Three-dimensional cavity preparations have the highest C-factor, and an increase in C-factor induces higher polymerization shrinkage stress . When a polymer reaches its gel point, flow of the resin composite ceases as it transforms from a viscous paste into an elastic solid. As post-gel polymerization shrinkage increases during the early stage of polymerization, contraction stress increases at a high rate as the resin composite rapidly gains rigidity, shrinks, and pulls away from the cavity walls . Therefore, the higher the number of bonded surfaces, the higher the degree of polymerization shrinkage stress in a bonded composite restoration because of the constraints imposed by the geometry of the cavity preparation.
In butt-joint cavity preparations, the interplay between polymerization shrinkage and marginal gap formation is suggested to be as follows. During the initial setting, the resin composite exerts a force on the cavity walls that is resisted by the adhesion mediated by the bonding system. As polymerization proceeds, shrinkage stress increases. When shrinkage stress exceeds the bond strength, a gap is formed. If shrinkage stress exceeds bond strength late in the polymerization process, gap formation would be limited; if this occurs early in the polymerization process, gap formation might be extensive. For a resin composite with high volumetric polymerization shrinkage, high shrinkage stress may rapidly develop which then induces large gap formation. However, shrinkage stress can be reduced by the viscous flow of the resin composite supplied from the free, unbonded surfaces .
Tooth cavities or Teflon molds simulating tooth cavities have been used in many studies to examine the effect of resin polymerization shrinkage on marginal gap formation in composite restorations, as well as the effects of other contributing factors such as the adhesive bonding of restorative filling materials to the cavity walls . To the best of the authors’ knowledge, no studies have been carried out to investigate a probable direct relationship between horizontal and vertical polymerization shrinkage in composite restorations in tooth cavities. Horizontal polymerization shrinkage, derived from measuring marginal gaps formed in a tooth cavity, is an indicator of the marginal adaptation of resin composite restorations. Vertical polymerization shrinkage involves the interaction of several factors: adhesive forces between composite restorative material and cavity walls, magnitude of volumetric shrinkage of composite restorative material, and viscosity/flow capability of composite material.
In today’s restorative dentistry, composite restorative materials are used in conjunction with a plethora of self-etch adhesives and all-in-one adhesives to save chairside time and maximize patient comfort. They vary in terms of composition and concentration of polymerizable acids and acidic resin monomers . For a comprehensive and clinically relevant investigation, a wide selection of contemporary light-activated composite restoratives and bonding systems were employed in this study to investigate the following correlations which might occur immediately after light activation: (1) between vertical ( S V , %) and horizontal ( S H , %) polymerization shrinkage in tooth cavities; (2) between S V and shear bond strengths to enamel ( B E ) and dentin ( B D ); and (3) between S H and marginal gap width in a Teflon mold.
Vertical and horizontal polymerization shrinkage were reportedly dependent on the C-factor, adhesive bonding at tooth-restoration interface, and viscous flow of composites . The hypotheses to be tested in this study were: (1) S V is correlated with S H ; and (2) B E and B D are inversely correlated with S V or S H .
2
Materials and methods
2.1
Light-activated composite restorative materials
Ten light-activated composite restorative materials were selected for this study. Details of the composite restorative materials are listed in Table 1 , and those of their accompanying bonding systems are listed in Table 2 . This range of composite restorative materials and bonding systems was thus selected to represent the major restorative products used by dentists and also to provide a comprehensive, clinically relevant range of values for the parameters under investigation.
Product | Composition | Manufacturer | Code | Lot No. |
---|---|---|---|---|
Esthet-X HD | Barium boron fluoroaluminosilicate glass, silicon dioxide | Dentsply/Caulk | EST | 0903000635 |
Titanium dioxide, sodium alumino silica (75 wt%, 66 vol%) | Milford, DE, USA | |||
Urethane modified Bis-GMA, photo initiators, stabilizers | ||||
Filtek Supreme DL | Zirconia/silica cluster filler (76 wt%, 60 vol%) | 3M ESPE, | FIL | 3TC |
Bis-GMA, UDMA, Bis-EMA, photo initiators | St. Paul, MN, USA | |||
Stabilizers | ||||
Premise | Barium aluminumborosilica (76 wt%, 56 vol%) | Kerr, Orange, CA, USA | PRE | 112330 |
Bis-GMA, TEGDMA, EBPADMA | ||||
Tetric EvoCeram | Bariumglass filler, ytterbium trifluoride, mixed oxide | Ivoclar vivadent AG, | TET | KO4764 |
Prepolymer (75–76 wt%, 53–55 vol%) | Schaan,Liechtenstein | |||
Dimethacrylates, additives, catalysts, stabilizers, pigments | ||||
Venus Diamond | Barium aluminum, fluoride glass, highly discrete nanoparticles | Heraeus Kulzer, Hanau | VEN | 010030 |
(80–82 wt%, 63.5–65.1 vol%) | Germany | |||
TCD-DI-HEA, UDMA | ||||
Kalore | Fluoro-aluminosilicate-glass, strontium/barium glass | GC, Tokyo, Japan | KAL | 0807112 |
Composite filler, silicon dioxide (82 wt%, 69 vol%) | ||||
UDMA, (Technology from Dupont), Dimethacrylate, | ||||
Pigment, photo initiators | ||||
Beautifil II | S-PRG filler, multi-functional glass filler | Shofu, Kyoto, Japan | BEA | 110615 |
Ultra-fine filler (83.3 wt%, 68.6 vol%) | ||||
Bis-GMA, TEGDMA, UDA, photo initiators | ||||
Fantasista | Barium glass filler, TMPT reactive filler silica, Sr-glass filler | Sun Medical | FAN | TE6 |
(76 wt%, 57 vol%) | Moriyama, Japan | |||
Dimethacrylates, photoinitiator, stabilizer | ||||
Estetite Sigma Quick | Silica/zirconia filler, composite filler (84 wt%, 71 vol%) | Tokuyama Dental | EST | J213 |
Bis-GMA, TEGDMA, photo initiators | Tokyo, Japan | |||
Clearfil Majesty | Silanater glass ceramics, surface treated alumina micro filler | Kuraray Medical | CLE | JP6M |
Posterior | (92 wt%, 82 vol%) | Kurashiki, Japan | ||
Bis-DGMA, TEGDMA, hydrophobic aromatic dimetnacrulate, | ||||
dl -Camphorquinone |
Adhesive | Composition and surface treatment | Manufacturer | Batch No. |
---|---|---|---|
Xeno IV | Polymerizable organophosphate monomer | Dentsply/Caulk | 081229 |
Polymerizable organocarboxlic acid monomer | Milford, DE, USA | ||
Polymerizable tri/dimethacrylate resin | |||
Light cure initiator, stabilizer, acetone | |||
Xeno IV (20 s) – air – light (10 s) | |||
Adper Prompt L -Pop | Methacrylated phosphoric acid ester, water, phosphine oxide | 3M ESPE AG, Seefeld | 177396 |
Stabilizer, Fluoride complex | Germany | ||
Adper Prompt L-Pop (15 s) – air – light (10 s) | |||
OptiBond SoLo ALL-IN-ONE | HFGA-GDM, GPDM, ethanol, water phototoinitiator | Kerr, Orange, CA | 208113 |
Self-Etch primer (15 s) – air – OptiBond SoLo ALL-IN-ONE (15 s) – air – OptiBond SoLo Plus (15 s) – air – light (20 s) | USA | ||
AdheSE One F | Bis-acrylamide derivative, water | Ivoclar vivadent AG, | R 320611 |
Bis-methacrylamide dihydrogenphosphate, | Schaan, Liechtenstein | ||
Amino acid acrylamide, hydroxyalkyl methacrylamide, | |||
Silicon dioxide, fluoride | |||
AdheSE One F (10 s) – air – light (10 s) | |||
iBond | UDMA, 4-META, acetone, water, fillers, photoinitiator | Heraeus Kulzer, Hanau | 010082 |
iBond (20 s) – air – light (20 s) | Germany | ||
G-Bond Plus | 4-MET, phosphoric ester monomer, dimethacrylate monomer, | GC, Tokyo, Japan | 0404021 |
Water, acetone, phototoinitiator | |||
G-Bond Plus (10 s)– strong air – light (10 s) | |||
FL-BOND II | Primer: carboxylic acid monomer, phosphonic acid monomer, | Shofu, Kyoto, Japan | 787T2060712 |
Water, solvent, initiator | |||
Bond: S-PRG filler, UDMA, TEGDMA, HEMA, photo-initiator, | |||
Primer (10 s) – air – Bond – light (10 s) | |||
AQ Bond SP | Bond: 4-META, poly-function(math)acrylate, monomethacrylates, | Sun Medical | TE1 |
Water-acetone, photo initiator, stabilizer | Moriyama, Japan | ||
Cata-sponge: sopdium p-toluenesulfinate, aromatic amine | |||
AQ bond SP (20 s) – gentle air (15 s) – strong air (5 s) – light (10 s) | |||
Tokuyama Bond Force | Phosphoric acid monomer | Tokuyama Dental | 001 |
Bis-GMA, TEGDMA, HEMA, ethanol, photoinitiatar | Tokyo, Japan | ||
Tokuyama Bond Force (20 s) – air – light (10 s) | |||
Clearfil SE Bond | Primer: MDP, HEMA, hydrophilic dimethacrylate | Kuraray Medical | 00404A |
DL -Camphorquinone, N,N-diethanol-p-toluidine, water | Kurashiki, Japan | ||
Bond: MDP, Bis-GMA, HEMA, hydrophobic dimethacrylate | |||
DL -Camphorquinone, N,N-Diethanol-p-toluidine | |||
Silanated colloidal silica | |||
Primer (20 s) – air – Bond – light (10 s) |
Tooth preparation procedures, mixing, and handling were carried out according to manufacturers’ recommendations ( Table 2 ). A visible light-curing unit (New Light VL-II, GC, Tokyo, Japan; fiber optic tip diameter: 8 mm) was used to irradiate the light-activated materials for 40 s. Using a radiometer (Demetron/Kerr, Danbury, CT, USA), light intensity was checked immediately before the application of each adhesive resin and composite restorative material. During light curing, light intensity was maintained at 450 mW/cm 2 .
2.2
Human teeth
Human premolars, extracted for orthodontic reasons, were used in this study. This study was approved by the Ethics Committee of Okayama University Graduate School of Medicine, Dentistry and Pharmaceutical Sciences, and Okayama University Hospital. After extraction and cleaning, teeth were immediately stored in cold distilled water at 4 °C for 2–3 months before use.
2.3
Measurement of polymerization shrinkage in tooth cavity
Each tooth was embedded in a slow self-curing epoxy resin (Epofix, Struers, Copenhagen, Denmark). A flat enamel surface was obtained by grinding the tooth with wet 220-grit silicon carbide paper until a plane area of at least 4 mm diameter was exposed. With the tooth firmly held in a custom-made drill press, a cylindrical cavity of approximately 1.5 mm depth and 3.5 mm diameter was prepared with the sequential use of a tungsten carbide bur (200,000 rpm) and a customized bur (4000 rpm) under wet conditions. One cavity preparation was made in each tooth in the coronal region and on the mesial surface. A total of 200 cavities were prepared in 200 teeth for this study.
2.3.1
Measurement of vertical shrinkage, S V (%)
Ten tooth cavity specimens were prepared for each composite restorative material as follows. After tooth preparation, the center of each cavity was inspected and measured using a measuring microscope at ×400 magnification (Measurescope MM-11, Nikon, Tokyo, Japan). With reading accuracy at 1 μm in X – and Y -directions and 0.5 μm in Z -direction (Digimicro ME 50 HA, Nikon, Tokyo, Japan), baseline depth of unfilled tooth cavity between top and bottom surfaces was recorded as L 0 .
After baseline depth measurement, cavity walls and surrounding enamel margins were treated according to manufacturers’ instructions as described in Table 2 . Each cavity was filled with a restorative material using a syringe tip (Centrix C-R Syringe System, Centrix, CT, USA). Using the same reference point at which L 0 was measured, depth was measured again using the same procedure at a time lapse of 3 min from the start of light activation. Polymerization shrinkage on the bottom interface, if any, would result in a lower depth measurement value after light activation. Depth difference before and after light activation was then as recorded ( L 1 ) ( Fig. 1 ), which took into account polymerization shrinkage at both the top and bottom interfaces. The L 0 and L 1 values of each specimen were used in the following equation to calculate vertical polymerization shrinkage, S V , expressed as a percent (%):
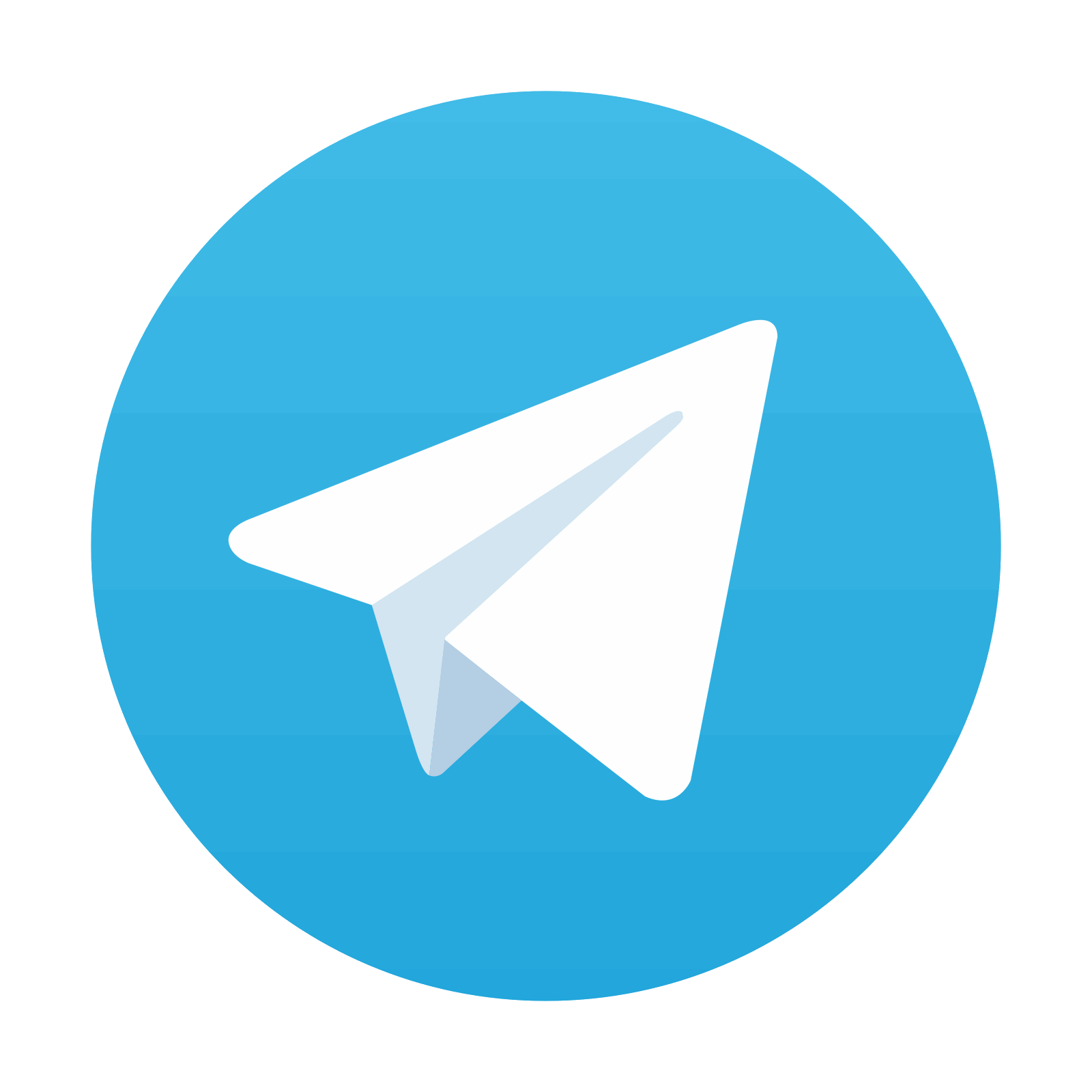
Stay updated, free dental videos. Join our Telegram channel

VIDEdental - Online dental courses
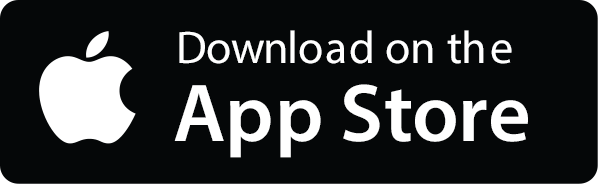
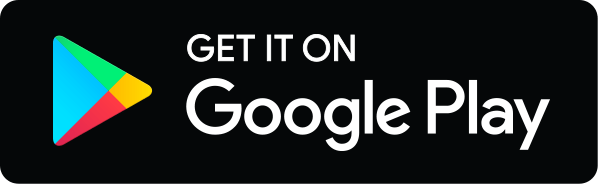