Abstract
Objectives
The bonding interface of glass ceramics and resin luting cements plays an important role in the long-term durability of ceramic restorations. The purpose of this systematic review is to discuss the various factors involved with the bond between glass ceramics and resin luting cements.
Methods
An electronic Pubmed, Medline and Embase search was conducted to obtain laboratory studies on resin–ceramic bonding published in English and Chinese between 1972 and 2012.
Results and discussion
Eighty-three articles were included in this review. Various factors that have a possible impact on the bond between glass ceramics and resin cements were discussed, including ceramic type, ceramic crystal structure, resin luting cements, light curing, surface treatments, and laboratory test methodology.
Conclusions
Resin–ceramic bonding has been improved substantially in the past few years. Hydrofluoric acid (HF) etching followed by silanizaiton has become the most widely accepted surface treatment for glass ceramics. However, further studies need to be undertaken to improve surface preparations without HF because of its toxicity. Laboratory test methods are also required to better simulate the actual oral environment for more clinically compatible testing.
1
Introduction
All-ceramic materials are well known for the esthetic ability of imitating tooth color. Recent developments in ceramic restoration fabrication techniques came about with ceramics possessing higher strength and toughness , making it possible for wider applications in dentistry such as veneers, inlays/onlays or dental implants .
Nowadays, there are increasing cases where the retention of restorations is reliant on bonding, e.g. resin-bonded bridges. Hence, the quality of bonding is of increasing importance, and is a dominant factor required for the long-term success of glass ceramic restorations. Compared with traditional cements such as polycarboxylate or glass ionomer cement, resin luting cements were introduced to aid all-ceramic restoration retention . Resin cements not only provide stronger and more durable bonding between ceramics and teeth, but can also achieve better esthetic outcomes and maintain higher ceramic strength .
Ceramics in dentistry can be generally classified into “oxide ceramics” and “glass ceramics” based on the chemical composition. Oxide ceramics are defined as a group of ceramics containing not more than 15% silica with little or no glass phase . They can be subclassified into aluminium-oxide/alumina ceramics, including glass-infiltrated alumina ceramics, densely sintered alumina ceramic systems, and zirconium-oxide/zirconia ceramics, including glass-infiltrated and densely sintered zirconia ceramic systems. Because of their stable chemical structure, oxide ceramics have significantly improved mechanical properties and can be called “high strength core ceramics”. However, the surface cannot be etched by hydrofluoric acid (HF), which is the usual chemical means to condition the surface to obtain micro-mechanical adhesion. These oxide ceramics are known as “acid-resistant ceramics” . Nevertheless, caution should be drawn in using such terminology as recent studies have only tested this type of ceramic with HF but not other acids.
It is accepted that adhesion between ceramics and resin cements is provided by two major mechanisms: micromechanical attachment and chemical bonding. Micromechanical attachment can be created by acid etching and/or sandblasting , whilst a silane coupling agent provides a chemical bond . Various glass ceramics differ in chemical conformation and microstructure, so it might be necessary to establish bonding procedures according to the glass ceramic type.
The ceramic and resin cement bond is subjected to a complex environment in the oral cavity being influenced by several extrinsic factors such as temperature change, saliva, daily food and drink intake, biting force and other habits. Consequently, laboratory testing should simulate these variables to enable the development of superior materials and surface preparation methods that provide long-term durable bond strengths in the oral environment.
The aim of this systematic review was to evaluate laboratory studies investigating the resin luting cement (resin cement) bond to dental ceramics.
2
Materials and methods
2.1
Search strategy
A search of the Pubmed library, Medline, Embase and the Cochrane library was performed on the literature available from 1972 to April 18, 2012. The keywords used were: ‘bond’, ‘bonding’, ‘adhesive’, ‘adhesion’, ‘luting’, ‘resin cement’, ‘resin composite’, luting composite’, ‘luting agent’, ‘luting resin’, ‘porcelain’, ‘ceramic’, ‘ceramics’. English and Chinese papers were included in this search.
2.2
Articles reviewed and data extraction
The included articles were independently reviewed by two reviewers. Inclusion criteria were studies that evaluated bonding aspects between the resin cement and glass ceramic interface in laboratory tests;
Exclusion criteria were studies on:
- (1)
alumina-based or zirconia-based ceramic;
- (2)
brackets, posts or implants;
- (3)
evaluating ceramic/porcelain repair systems;
- (4)
tooth preparation, tooth conditioning, and resin-enamel/dentin bonding;
2
Materials and methods
2.1
Search strategy
A search of the Pubmed library, Medline, Embase and the Cochrane library was performed on the literature available from 1972 to April 18, 2012. The keywords used were: ‘bond’, ‘bonding’, ‘adhesive’, ‘adhesion’, ‘luting’, ‘resin cement’, ‘resin composite’, luting composite’, ‘luting agent’, ‘luting resin’, ‘porcelain’, ‘ceramic’, ‘ceramics’. English and Chinese papers were included in this search.
2.2
Articles reviewed and data extraction
The included articles were independently reviewed by two reviewers. Inclusion criteria were studies that evaluated bonding aspects between the resin cement and glass ceramic interface in laboratory tests;
Exclusion criteria were studies on:
- (1)
alumina-based or zirconia-based ceramic;
- (2)
brackets, posts or implants;
- (3)
evaluating ceramic/porcelain repair systems;
- (4)
tooth preparation, tooth conditioning, and resin-enamel/dentin bonding;
3
Results
After searching and screening the database, a total of 83 articles were included. Owing to the high heterogeneity of studies regarding preparation of materials, methods of testing and outcome variables, it was not possible to analyze the data quantitatively. Articles are summarized in Section 4 concerning factors related to the ceramic-resin bond.
4
Discussion
4.1
Ceramics
Glass ceramics, also termed silica-based ceramics , are a group of materials that have been widely used for all-ceramic restorations since the 1970s. These can be further classified as feldspathic, leucite-reinforced, fluormica glass or lithium disilicate ceramic. HF etching has a distinctive effect on the surface of these ceramics creating a uniformly porous surface . This distinctive effect has made this group of glass ceramics dominate resin-bonded ceramic restoration construction and therefore glass ceramics are the focus of the present review.
Despite glass ceramics exhibiting lower mechanical strength than oxide ceramics, the fracture resistance has been shown to increase with resin cementation . One study suggested that using a relatively thin layer (approximately 100 μm) of resin cement bonded to leucite-reinforced and lithium disilicate ceramics on HF treated surfaces and silanization could significantly increase the biaxial flexural strength compared with ceramics with the same surface preparations but without resin cement application .
Several studies have suggested that various glass ceramic types may produce different microstructures after different surface treatments, which can consequently affect the bond strength between ceramic and resin cement . It was demonstrated that a feldspathic ceramic exhibited a significantly higher shear bond strength than a leucite-reinforced ceramic when luted with the same resin cement after 20,000 thermocycles . When comparing leucite-reinforced and lithium disilicate ceramic, one study showed a resin cement which luted to a leucite-reinforced ceramic had a higher Vickers hardness. The authors attributed the difference to the variation of microstructure in the two ceramic types. The SEM image of HF-etched lithium disilicate glass ceramics showed a surface with a scaffold-like structure, comprising many small interlocking needle-like crystals without orientation. Further, a second crystalline phase, consisting of a lithium orthophosphate (Li 3 PO 4 ) of a much lower volume was also reported. On the other hand, the microstructure of HF-etched leucite-reinforced ceramic was less dense in crystal structure and characterized by single leucite crystals, without interlocking between crystals. However, a further study showed that the microtensile bond strength was significantly higher for lithium disilicate ceramics than leucite-reinforced ceramics with the same surface preparation . It seems there is little correlation between the hardness of resin cement and the resin–ceramic bond strength. It is also worth noting that a single application of silane coupling agent produced the highest bond strength in the leucite-reinforced ceramic, while HF acid etching combined with silanization created the highest bond strength in the lithium disilicate ceramic. Thus, ceramic type may need different surface preparation methods for optimal adhesion, however, no further explanation was presented . Another study compared the microshear bond strength between two leucite-reinforced ceramics, GN-I ® (GC Corporation, Tokyo, Japan) and ProCAD™ (Ivoclar Vivadent, Schaan, Liechtenstein). The results showed that when using the same silane application, GN-I ® presented a higher bond strength than ProCAD™. Nevertheless, no comparison of chemical composition or microstructure of these two materials was performed. Thus, it is unclear regarding the exact mechanism of how the surface morphology of these glass ceramics affected the resin–ceramic bond.
The different surface morphology of ceramics may also affect adhesion. It was shown with the same unconditioned glass ceramic surface, the microtensile bond strength of RelyX™ Unicem (3M Espe AG, Seefeld, Germany) was significantly higher than that of Multilink (Ivoclar Vivadent, Schaan, Liechtenstein) and Panavia F (Kuraray Medical Inc., Tokyo, Japan). However, after HF etching and silanization of the ceramic, statistically higher microtensile bond strength values for RelyX™ Unicem and Multilink were obtained compared with Panavia F. This suggests different resin cements may have an individual bonding capability by varying surface morphology with various surface treatments .
Ceramic translucency has been reported to have an effect on the cured resin hardness . Numerous factors affect ceramic translucency, including thickness , crystalline structure , number of firings, repeated staining cycles , grain size, pigment, number, size and distribution of defects, and shade . Results showed that increasing thickness and opacity of glass ceramics produced a statistically significant decrease in hardness of tested resin cements . Although the translucency of the ceramic may affect resin cement polymerization, it seems to have no or little effect on the bond strength between resin cement and ceramic. It was illustrated that ceramic thickness, polymerization mode, storage time, or combinations of these parameters did not influence shear bond strength between lithium disilicate ceramics and resin cements .
4.2
Resin cements
The cementation procedure also plays a significant role in the clinical success of indirect fixed restorations. The resin cement links the restoration and tooth. Resin luting cements can be classified as light-cured, self/auto-cured or dual-cured based on polymerization mode, without considering the dentin pretreatment method.
Since the 1970s, resin cements have been used to lute glass ceramic restorations . Resin cements exhibit some clinical advantages over other conventional luting cements, e.g. zinc phosphate, polycarboxylate and glass ionomer cements. Resin cements can provide a clinically acceptable margin after fatigue testing that has been shown to minimize leakage and thus reduce the risk of caries and restoration failure . Resin cements have various shades that can offer a more natural esthetic appearance compared with opaque conventional luting cements . The requirement for a retentive tooth preparation is reduced for resin cements because of their adhesion properties, thus preserving healthy tooth structure .
4.2.1
The effect of resin cement on the ceramic-resin bond
Resin cements exhibit a diverse bonding potential with glass ceramics. Higher shear bond strengths were obtained when using Variolink II (Ivoclar Vivadent, Schaan, Liechtenstein) or RelyX Unicem bonded to HF etched and silanized lithium disilicate ceramics after thermocycling, compared to Panavia F . This result was supported by another study using the same ceramics and surface treatments. That study showed groups bonded with Multilink or RelyX™ Unicem had higher microtensile bond strengths than the group bonded with Panavia F in thermocycled conditions. Similar results were also found in HF-etched and a silanized feldspathic ceramic which were cemented with Noribond DC (Noritake Dental Supply, Inc., Aichi, Japan) or Variolink II exhibited significantly higher shear bond strengths than Panavia 21 for 3 and 150 days water storage . Another study using thermocycling showed Variolink II had a statistically higher shear bond strength to lithium disilicate ceramics than Panavia 21, Panavia F, RelyX Unicem and RelyX ARC groups . However, in that study, the surface treatments of the ceramic-cement groups were inconsistent: Variolink II and RelyX™ ARC groups used HF acid-treatment then silanization; for Panavia 21 and Panavia F groups, phosphoric acid treatment and silanization were used, and the RelyX™ Unicem group were directly cemented without any surface treatment. Such variations in surface treatment methods may lead to bias of the results and invalidate comparisons. It seems that although Panavia is regarded as a gold standard for resin cements, it shows relatively low bond strength compared with other resin cements. Some studies explained that the poor bonding performance of Panavia F was due to the inhibition of polymerization by acidic monomers present in the cement .
Resin cement factors that may influence the resin–ceramic bond, including curing mode, elastic modulus, color shade and the thickness have limited information available.
Compared with light-cured and dual-cured resin cements, it was concluded that light activated materials performed better than dual-cured cements . Other studies showed that dual-cured resin cements exhibited significantly higher extrusion shear bond strengths with a feldspathic ceramic than the corresponding bond strengths for an auto-cured cement .
The elastic modulus of the luting agent seems to have little effect on the stresses in a ceramic crown. A finite element analysis study showed that when a resin cement with a higher elastic modulus was replaced with a low elastic modulus cement, the magnitude of tensile stresses in the glass ceramic did not decrease . Another study observed there might be a strong positive linear relationship between the mean biaxial flexural strength of the feldspathic ceramics and the flexural elastic modulus of different resin cements . This indicated that the elastic modulus of resin cements may have an effect on the strengthening of ceramics. However, in that study , the ceramic surfaces were not conditioning, and may not reflect the clinical situation. Thus, further research on the effect and relationship of resin cement elastic modulus on surface treated ceramics is needed.
The shade of resin cement has little effect on the bond between either ceramic or dentin. A microtensile bond test compared two different shades of a dual-cured resin cement, Variolink II, A3 and transparent (Tr), bonded to a feldspathic ceramic. No significant difference was found between these two shades using the same curing light and storage period (no storage and 150 days storage) .
The bond strength between glass ceramics and resin cements was postulated to decrease with an increase of resin cement film thickness . A finite element model experiment demonstrated that an increase of cement thickness from 10 to 180 μm resulted in higher stresses developing within the resin cement. This study further investigated, and showed, a resin cement thickness less than 50 μm might create a better bond to a glass ceramic compared to a film thickness of greater than 50 μm .
4.2.2
The effect of external factors on resin cements
Except for resin cement there can be other influential factors for resin–ceramic bonding, some external factors, such as the ceramic (which was discussed in the ceramic section) and the light curing unit, can also have an impact on the polymerization of resin cements and potentially affect the bond between a resin cement and ceramic.
Three common types of light curing units are commercially available, namely quartz halogen (QTH: quartz tungsten halogen), light emitting diode (LED) and Xenon plasma arc (PAC). QTH lights are the original device commonly used to activate resin-based materials. Nevertheless, several drawbacks of QTH lights were found in the last decade, such as a limited effective working time of around 100 h . The heat generated in the bulb lead to the bulb degradation and ultimately reduced polymerization capacity . The LED system was proposed for light curing to overcome the drawbacks of the QTH. Compared with QTH lights, LED lights has the advantage such as smaller size, lighter, lower heat and noise generation, greater longevity of the output intensity and unit, and less consumption of electricity . Plasma-arc curing lights are designed for high-speed polymerization which is a significant time saving of the curing procedure, but the heat generated can be high .
No difference in microtensile bond strength was found between QTH and LED light-cured resin cements when studied . Similar results were observed in a shear bond strength study of a resin cement to ceramic and dentin when the cement was polymerized using QTH light in standard mode and a high-powered (1100 mW/cm 2 ) LED light in exponential mode. However, the shear bond strength was significantly lower when cured using LED in the fast and pulse modes. This implies the light output energy of an LED unit may influence the resin cement curing . Further, higher hardness, were observed with an LED compared with a QTH light whilst another study showed no difference between these two types of curing unit . The possible reason might be due to the different time duration of light curing. When comparing QTH light and plasma arc light units, 60-s QTH light source produced microtensile bond strength greater than those achieved with a 9-s exposure with the plasma arc light .
It is worth noting that the long-term duration of resin–ceramic bonding does not completely rely on a high degree of polymerization and cement mechanical properties. A longer and higher intensity of light exposure may enhance the polymerization of the resin cement, but it may cause more rapid shrinkage of the material which might be detrimental to the bond due to rapid stress increase. Therefore, it is important to use the curing time following the manufacturers’ instructions of the resin cement.
4.3
Surface treatment
The morphology and chemical properties of the ceramic surface are very important factors for the ceramic-resin bond. These properties can be achieved by application of chemical conditioning agents and/or mechanical treatments by creating a micromechanical and/or chemical bond to the resin cement.
4.3.1
Chemical conditioning methods
4.3.1.1
Hydrofluoric acid (HF) etching
Hydrofluoric acid is an aqueous solution of hydrogen fluoride. It is a weak acid because its H F bonding is relatively more stable than the bonding of other strong acids ( e.g. H Cl etching) , thus the free hydronium ion (H 3 O + ) function is not strong. HF can be used for dissolution of the ceramic glass phase surface by reacting with silicon dioxide. This increases the roughness of the ceramic surface and consequently creates a micromechanical interlock between the ceramic and resin luting cement. The reaction process is shown as:
It is noteworthy that the dissolution process does not rely on the “acidic” property of HF, but the fluoride substitution to oxygen due to electronegativity in glass that forms the Si.F glass. Thus, the term “acid etch” is misleading and therefore “HF etching” is preferred. HF has a long history in the pretreatment of silica-based ceramics before bonding. This was first described by Horn et al. who showed a successful increase in bond strength between a thin layer of porcelain and resin by the application of HF , to obtain a tensile bond strength of 7.5 MPa .
From the early 1990s, further studies were carried out in order to analyze the effect of the concentration and etching time of HF on the bond strength between ceramics and resin cements . It was reported, where combinations of HF concentrations (2.5%, 5.0%, 7.5%, 10%, 15%) and etching times (0.5 min, 1 min, 2.5 min, 5.0 min, 7.5 min, 10 min) were examined on feldspathic ceramics, that no direct and obvious correlation was found between the etching period and etchant concentration. The best etching periods for 2.5%, 5.0%, 7.5%, 10%, 15% HF were 5 min, 7.5 min, 10 min, 1.0 min, 0.5 min respectively. The group in which the ceramic was treated with 10% HF for 1.0 min produced the highest shear bond strength (11.66 ± 1.91 MPa) . In another study that evaluated the effect of 5% HF gel etching time on the surface of a feldspathic ceramic showed a different outcome. This study found that with an increase in etching time, the shear bond strength also increased. The highest bond strength was achieved when the ceramic surface was etched for 2 min, and beyond this etching time, a reduction of the shear bond strength was observed . This phenomenon could be explained by the over-etching of the glass ceramic surface and adversely affecting strength . It was shown that 16.8% HF etching for 30 s seemed to over-etch the glass surface, resulting in lower bond strength than 16.8% HF etching for 5 s . A very recent study showed that 4.9% HF gel treatment of a lithium disilicate ceramic for 2 min produced the highest shear bond strength . Barghi et al. reported that low leucite content ceramics etched with 9.5% HF gel for 1 min showed the highest shear bond strength. They further revealed that with a similar concentration of HF, the gel (9.5%) produced better bond strengths than a liquid etchant (10.0%). Moreover, ceramics with various leucite concentrations could also have an effect on the bond strength. To date, it seems that 4% and 10% HF etching for 1–2 min is the most acceptable etching procedure for silica-based ceramics, depending on the HF material state (gel or liquid) as well as the number, size, and distribution of the crystals in the ceramic.
Some additional procedures have been attempted after the HF was rinsed off with water in order to enhance the ceramic-resin bond by eliminating excess acid . One study evaluated the application of a neutralizing powder on the HF etched ceramic surface. It was not a recommended procedure for surface treatments of oxide ceramics because a significant reduction of microtensile bond strength in the neutralization group was found . Another study presented a similar result in neutralization application . Inasmuch as HF etch is not acting as an acid to etch the surface, such studies were erroneous in rationale and showed neutralization of the acid was pointless.
4.3.1.2
Substitutes of HF
Although HF can effectively enhance the bond between ceramics and resin, it is highly corrosive, can be absorbed into the blood and bone through the skin and may even lead to cardiac arrest . Hence, a number of chemicals were introduced to substitute HF, e.g. phosphoric acid, acidulated phosphate fluoride and ammonium hydrogenfluoride.
The phosphoric acid used in dentistry is orthophosphoric acid, which is an inorganic acid with the chemical formula H 3 PO 4 . It is mainly used to clean the ceramic surface and create a rough surface for better bonding. However, a study showed that the application of 40% phosphoric acid for 5 s or 60 s did not show any obvious morphological change on the ceramic surface under SEM observation . Obviously, the acidity is not important in the etching process, whilst the role of fluoride on the atomic displacement with silicon dioxide is of greater importance.
Acidulated phosphate fluoride (APF) has a empirical formula NaH 3 PO 4 F . The APF acid seems to prefer depositing on the leucite-based ceramic surface . Ammonium bifluoride (ABF) is a glass etchant with the chemical formula NH 4 HF 2 . It reacts with the silica constituent of glass in the following manner:
It was observed that ABF mainly attacks phase boundaries and cracks that already exist or are induced by leucite, which as a consequence, created a linear defect pattern. Such a pattern was also observed when HF was applied for a shorter time and lower concentration, which implies that ABF acts in a fashion similar to a low concentration of HF acid .
4.3.1.3
Silanization
Silane coupling agents provide a chemical bond between the resin and glass ceramic. Silanes have an inorganic group that reacts with Si OH on the ceramic surface by a condensation reaction. They also have an organic group that can chemically bond to methacrylate-based resins . Silanes have to be activated by acid hydrolysis with the use of acetic acid.
Several silane coupling agents are used to enhance chemical bonding between ceramics and resin composite . The most commonly used silane in dentistry for ceramic–resin bonding is 3-methacryloxypropyltrimethoxysilane (or γ-methacryloxypropyltrimethoxysilane). The abbreviations of this silane has been published as MPS, γ-MPTS or MTS . MPS is found to enhance shear bond strength of the resin–ceramic compared to 3-methacryloxypropylmethyldimethoxysilane (MDS) . It was noted that blended silanes of 1,2-bis(trimethoxysilyl)ethane (BTS) and MPS could significantly increase the shear bond strength between a resin and a leucite-reinforced ceramic after thermocycling . Such a blended silane system was shown in a laboratory study to enhance shear bond strengths between resin and zirconia, especially after thermocycling. Thus it seems blended silane systems could be a solution to enhance surface adhesion, particularly in dentistry.
Silanes have been shown to reduce the contact angle and increase the wettability of the ceramic surface . The silane layer is normally around 10–50 nm thick. It was found that cohesive destruction of layers occurs if a successive number of silane layers are applied on the surface . Thus, it is recommended that a thin silane coating on ceramic surface should occur in order to achieve satisfactory bonding. On the other hand, heat treatment of the silane is another method to reduce the silane coating thickness . A significant increase in microtensile bond strength between leucite-reinforced ceramic and resin cement occurred after drying the silane (MPS) with a 100 °C warm air stream . However, some studies demonstrated that heat treatment could not improve the shear bond strength of the adhesion promoter MPS between a resin cement and leucite-reinforced ceramic, but enhanced the bond performance for 3-methacryloxypropylmethyldimethoxy silane (MDS), 3-methacryloxypropyltriethoxysilane (MTES), and 3-acryloxypropyltrimethoxysilane (ACPS). Thus, the chemical composition of the silane primer plays a more important role than heat treatment to optimize the bond between resin and leucite-reinforced ceramics . Another study showed that heat treatment of the silane did not improve the bond strength .
Further, not only the silane monomer, but also the other primer ingredients can have a considerable affect on adhesion . Experimental ceramic primers have been shown to exhibit a higher ceramic bond durability than commercial ceramic primers . A significant increase in shear bond strength between a resin and a leucite-reinforced ceramic after thermo-cycling was observed when hydrochloric acid (HCl) was added to the silane solution, in which HCl might act as an accelerator during the hydrolysis reaction of the methoxy part of the silane . Thiophosphoric methacrylate (MEPS) is also recommended to blend with the silane solution since MEPS can accelerate the hydrolysis of the silane monomer and promote ceramic bonding .
One study discovered that when an appropriate silane application method was used on the surface of leucite-reinforced ceramics, no significant difference in the bonding effect of a pre-activated MPS-based silane solution, acetic acid and ethanol even when it was stored for up to 1 year at room temperature .
4.3.2
Mechanical conditioning methods
4.3.2.1
Sand blasting
It is a process to roughen the ceramic surface by blasting with alumina (Al 2 O 3 ) particles. Twenty five to 50 μm alumina powder is commonly used in glass ceramics at a pressure of 0.28 MPa .
4.3.2.2
Laser irradiation
Nd:YAG and Er:YAG lasers were evaluated in a bond strength test of glass ceramics . SEM observation revealed that the Er:YAG laser created irregular lithium disilicate crystals on the surface. The higher the laser power the greater the irregularities of the ceramic surface . For feldspathic ceramics, it could be seen that the surface was randomly melted and corroded without any fissures or cracks when either an Nd:YAG or Er:YAG laser was applied to a ceramic surface .
4.3.2.3
Tribochemical (TBC) silica-coating
Tribochemical silica-coating is a sand blasting process that uses silica-coated alumina particles to coat and blast the ceramic surface, which involves blasting the powders with high pressure compressed air on to the ceramic surface. The pressurized powders generate kinetic energy that is absorbed by the surface and subsequently partially melts it. The silica-coated alumina powders are embedded into the ceramic surface and produce a surface silica layer . A silane coupling agent is then applied onto the silica-coated surface. As a result, a strong chemical bond can be formed between the ceramic and resin cement. A commercial product, Rocatec System (3M-ESPE, Seefeld, Germany) was reported to be an effective surface preparation by combining tribochemical silica-coating with silanization .
4.3.2.4
Pyrochemical silica-coating
This is a proprietary surface treatment that was introduced as the Silicoater system (Heraeus-Kulzer, Wehrheim, Germany). The substrate is passed through a flame with the injection of a silane solution. Then, a series of pyrochemical reactions occur between 150–200 °C and a silicon oxide intermediate layer is produced on the ceramic surface. After the substrate is cooled to room temperature, the silane coupling agent (3-methacryloyloxypropyl trimethoxysilane) is applied to the silicon oxide layer surface . This system was first used for enhancing the bond between resin and metal alloys. Although silicon oxide is already present in glass ceramics and this pyrochemical coating method was considered unnecessary, it was thought the deposition of a silica layer with this technique might help to improve the bond . It was shown that a significantly higher bond strength was achieved when this treatment was applied to a glass ceramic surface .
4.3.3
Comparison between different surface treatments
The comparison of bond strengths of various surface treatments is listed in Table 1 . It has been shown that HF etching on the ceramic can create higher surface roughness and consequently a higher bond strength than other methods . Della Bona et al. found that ceramics treated with HF showed significantly higher tensile bond strength values than acidulated phosphate fluoride and ammonium bifluoride treatment while no statistically significant difference was found between acidulated phosphate fluoride and ammonium bifluoride treated groups. It was also observed that higher microtensile bond strengths in leucite glass ceramics were obtained when treated with HF etching compared with phosphoric acid . Similar results were shown in another study confirming that etching with HF was a more effective surface treatment than phosphoric acid . HF etching on a feldspathic ceramic surface also showed a higher shear bond strength than either sandblasting or grinding alone when stored for either 24 h or 6 months . Despite the common procedure for the ceramics, surface roughening preparation including grinding, sandblasting and phosphoric acid application are considered as a basic preparation process of ceramics to obtain consistent adhesion.
Authors | Ceramic type | Surface treatment | Mean strength [MPa] | Test type | Storage condition | Comments |
---|---|---|---|---|---|---|
Chen et al. | a | A (5%) (0 s) | 0.0 | SBS | 24 h, 37 °C, W | |
A (5%) (5 s) | 12.3 | |||||
A (5%) (30 s) | 32.3 | |||||
A (5%) (60 s) | 35.9 | |||||
A (5%) (120 s) | 44.5 | |||||
A (5%) (180 s) | 41.0 | |||||
Nogami et al. | a | No treatment | 8.8 | SBS | 24 h, 37 °C, W | L 4-META |
L | 0.0 | E1 Porcelain Liner M | ||||
E1 | 18.9 | E2 Tokuso Ceramics Primer | ||||
L/E1 | 30.7 | |||||
B | 0.0 | |||||
E2 | 25.7 | |||||
B/E2 | 29.7 | |||||
No treatment | 0.0 | 24 h, 37 °C, W,TC | ||||
L | 0.0 | |||||
E1 | 2.3 | |||||
L + E1 (mixed) | 19.7 | |||||
B | 0.0 | |||||
E2 | 9.7 | |||||
B + E2 (mixed) | 22.4 | |||||
Taira et al. | b | No treatment | 6.8 | SBS | 60 min, RT, Dry; 24 h, 37 °C, W | |
B + E1 | 16.7 | E1 MDS | ||||
B + E1/L | 33.0 | E2 MTS | ||||
B + E2 | 18.1 | E3 GC ceramic primer | ||||
B + E2/L | 47.4 | E4 Clearfil ceramic primer | ||||
B + E3 | 29.1 | E5 Tokuso ceramic primer | ||||
B + E4 | 40.4 | E6 Porcelain Liner M | ||||
B + E5 | 46.8 | E7 Monobond Plus | ||||
B + E6 | 33.8 | L Metal primer II | ||||
B + E7 | 26.3 | |||||
Kara et al. | a | F | 2.33 | SBS | 7 d, RT, DW | |
A | 0.71 | |||||
G | 0.46 | |||||
Jedynakiewicz et al. | a | A + E | 26.2 | SBS | 24 h | |
H + E | 26.7 | |||||
c | D + E | 19.2 | ||||
H + E | 34.0 | |||||
Ruttermann et al. | a | A + E1 | 11 | SBS | 24 h, dry | E1 Monobond S |
F + E1 | 23 | E2 Pyrosil Primer MP 94 E | ||||
I + E2 | 27 | E3 experimental silane MPS | ||||
A + E3 | 12 | E4 experimental silane MPS | ||||
A + E4 | 8 | E5 Silane Primer | ||||
B + E5 | 16 | E6 Clearfil Procelain Bond Activator mixed with SE Bond Primer | ||||
B + E6 | 16 | |||||
A + E1 | 12 | TC | ||||
F + E1 | 26 | |||||
I + E2 | 30 | |||||
A + E3 | 8 | |||||
A + E4 | 8 | |||||
B + E5 | 4 | |||||
B + E6 | 16 | |||||
Stewart et al. | a | No treatment | 0–4.0 | SBS | 24 h, 37 °C, IS | 4 resin cements for each treatment: |
F | 1.1–3.5 | Nexus | ||||
E | 9.2–20.6 | Panavia 21 | ||||
F + E | 8.4–23.1 | RelyX ARC | ||||
A | 10.3–19.0 | Calibra | ||||
A + E | 16.0–21.7 | |||||
No treatment | 0 | 6 m, 37 °C, IS | ||||
F | 0 | |||||
E | 1.6–19.3 | |||||
F + E | 4.2–23.8 | |||||
A | 6.2–15.6 | |||||
A + E | 15.9–21.8 | |||||
Matsumura et al. | a | F + B | 17.1, 18.1 | SBS | 24 h, 37 °C, W | E1 Clapearl Bonding Agent |
F + B + E1 | 21.5, 32.4 | E2 Clearfil Porcelain Bond | ||||
F + B + E2 | 32.0, 33.8 | E3 Panavia Ceramic Primer | ||||
F + B + E3 | 18.1, 31.3 | 2 resin cements for each treatment: | ||||
F + B | 1.4, 11.4 | 24 h, 37 °C, DW; TC | Clapearl DC | |||
F + B + E1 | 23.0, 33.0 | Panavia 21 | ||||
F + B + E2 | 20.5, 32.2 | |||||
F + B + E3 | 12.5, 30.3 | |||||
Brum et al. | d | No treatment | 24.96 | SBS | 24 h, RT, dry; 24 h, 37 °C, W; TC | |
A + E | 44.47 | |||||
F + E | 31.05 | |||||
Hooshmand et al. | b | E (stored for 15 min) + M | 30.28 | TBS | 24 h, RT, dry | adhesive effectiveness will not deteriorate when stored for up to 1 year |
E (stored for 2 h) + M | 25.70 | |||||
E (stored for 1 m) + M | 25.70 | |||||
E (stored for 6 m) + M | 26.5 | |||||
E (stored for 1 y) + M | 30.23 | |||||
E (stored for 15 min) + M | 17.8 | 24 h, boiling water | ||||
E (stored for 2 h) + M | 14.0 | |||||
E (stored for 1 m) + M | 18.5 | |||||
E (stored for 6 m) + M | 14.8 | |||||
E (stored for 1 y) + M | 19.1 | |||||
Liu et al. | b1 | B (37%) + E1 | 16.31 | μSBS | No storage | b1 ProCAD |
B (37%) + E2 | 16.43 | b2 GN-I | ||||
B (37%) + E3 | 20.39 | E1 GC Ceramic Primer | ||||
b2 | B (37%) + E1 | 21.16 | E2 Clearfil Ceramic Primer | |||
B (37%) + E2 | 19.64 | E3 Porcelain Liner M | ||||
B (37%) + E3 | 23.02 | |||||
b1 | B (37%) + E1 | 12.00 | TC | |||
B (37%) + E2 | 13.90 | |||||
B (37%) + E3 | 12.04 | |||||
b2 | B (37%) + E1 | 13.22 | ||||
B (37%) + E2 | 15.94 | |||||
B (37%) + E3 | 16.09 | |||||
Naves et al. | b | A (10%) (10 s) + E | 19.4 | μSBS | 24 h, 37 °C, DW | |
A (20%) (10 s) + E | 22.3 | |||||
A (40%) (10 s) + E | 22.2 | |||||
A (60%) (10 s) + E | 17.8 | |||||
A (120%) (10 s) + E | 15.3 | |||||
A (10%) (10 s) + E + J | 17.4 | |||||
A (20%) (10 s) + E + J | 21.3 | |||||
A (40%) (10 s) + E + J | 21.1 | |||||
A (60%) (10 s) + E + J | 24.7 | |||||
A (120%) (10 s) + E + J | 20.4 | |||||
Della Bona et al. | b | A (9.6%) | 9.9 | μTBS | 30 d, 37 °C, W | |
C (4.0%) | 0 | |||||
E | 27.2 | |||||
A + E | 20.6 | |||||
C + E | 13.6 | |||||
d | A (9.6%) | 41.7 | ||||
C (4.0%) | 19.1 | |||||
E | 30.1 | |||||
A + E | 56.1 | |||||
C + E | 36.9 | |||||
Saavedra et al. | b | A + E | 10.2 | μTBS | No storage | |
A + K + E | 6.8 | |||||
A + E | 6.1 | MC | ||||
A + K + E | 5.0 | |||||
Amaral et al. | a | A (9%) (60 s) (gel) | 14.4 | μTBS | No storage | |
A (4%) (60 s) (gel) | 14.3 | |||||
A (5%) (60 s) (liquid) | 12.7 | |||||
A (5%) (60 s) (liquid) + K | 14.2 | |||||
A (9%) (60 s) (gel) | 13.9 | 24 h, 37 °C, DW; TC | ||||
A (4%) (60 s) (gel) | 15.5 | |||||
A (5%) (60 s) (liquid) | 12.4 | |||||
A (5%) (60 s) (liquid) + K | 10.6 | |||||
De Carvalho et al. | a | A + E | 17.6 | μTBS | 7 d, 37 °C, DW | |
A + E + M | 19.0 | |||||
E + M | 9.1 | |||||
E | 10.9 | |||||
Akyil et al. | a | No treatment | 8.02 | μTBS | 24 h, 37 °C, DW; TC | G1 Er:YAG |
A | 17.01 | G2 Nd:YAG | ||||
F | 14.58 | |||||
G1 | 5.28 | |||||
G2 | 6.51 | |||||
F + A | 9.08 | |||||
G1 + A | 12.25 | |||||
G2 + A | 11.73 | |||||
Peumans et al. | b | No treatment | 12.8 | μTBS | 24 h, 37 °C, W | |
B | 19.1 | |||||
B + E | 27.4 | |||||
B + E + J | 34.0 | |||||
A | 37.6 | |||||
A + E | 34.6 | |||||
A + E + J | 34.5 |
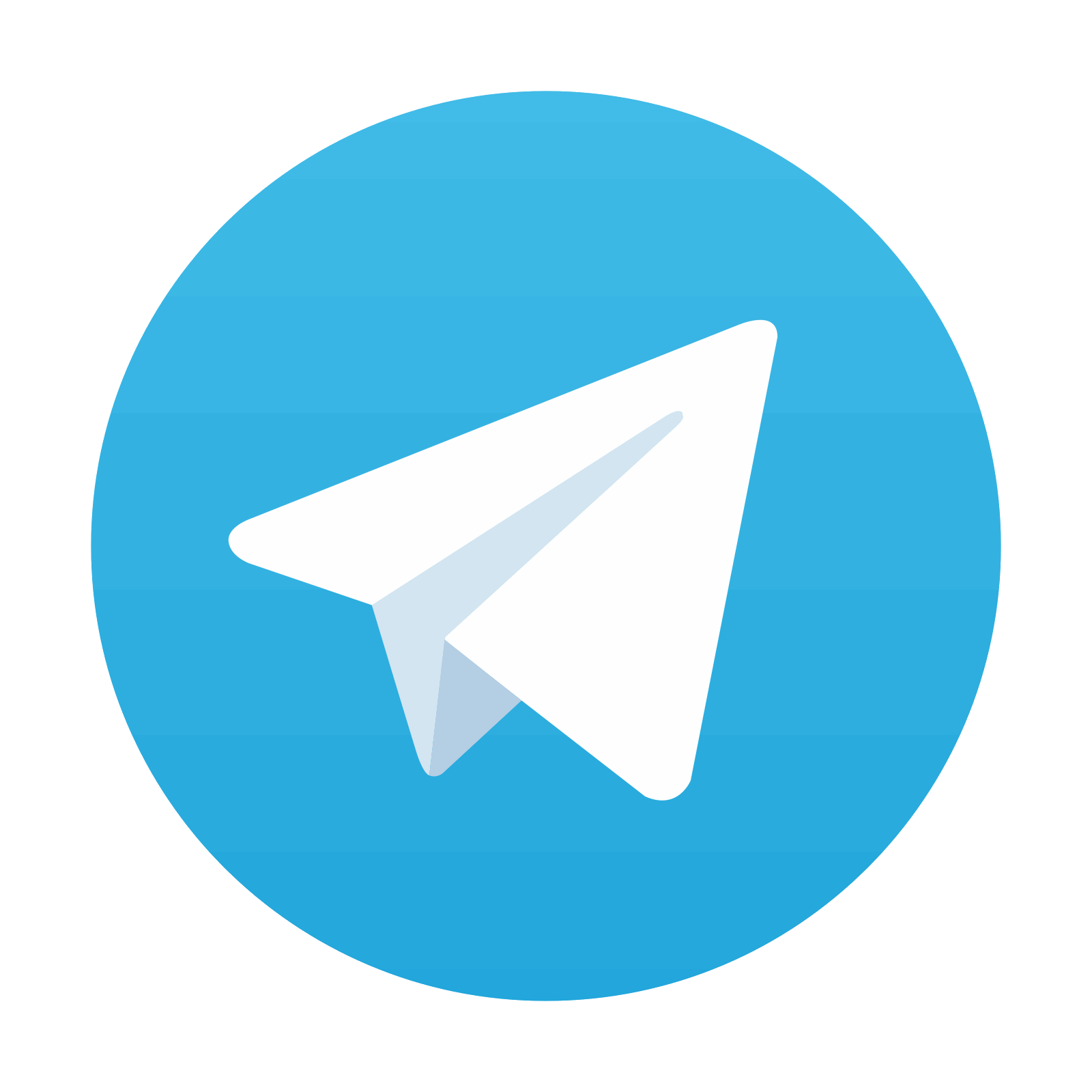
Stay updated, free dental videos. Join our Telegram channel

VIDEdental - Online dental courses
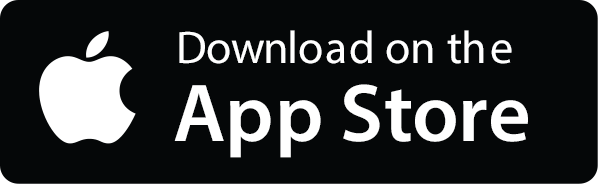
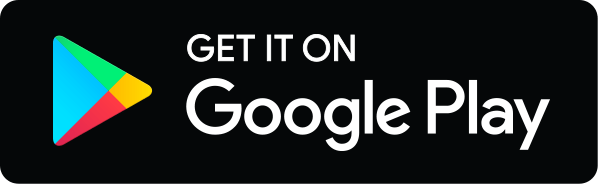
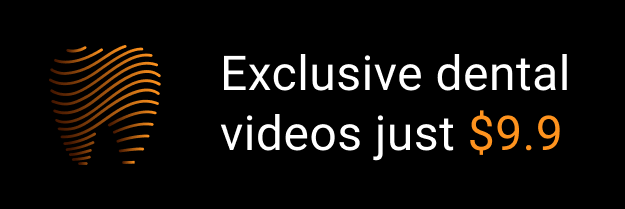