Abstract
Objective
Light-curable polymers are commonly used in restorative surgery, prosthodontics and surgical procedures. Despite the fact of wide application, there are clinical problems due to limitations of blue light penetration: application is restricted to defects exposed to the light source, layered filling of defect is required.
Methods
Combining photo-activation and up conversion allows efficient polymer hardening by deep penetrating near-infrared (NIR) light. The prerequisite 450 nm blue light to polymerize dental resins could be achieved by filler particles, which absorb the incident NIR irradiation and convert it into visible light.
Results
The on spot generated blue light results in uniform polymer hardening. Composite samples of 5 mm thickness were cured two times faster than pure polymer cured by blue light (30 and 60 s, respectively). Overall degree of monomer conversion resulted in higher values of more than 40%. The enhanced transmission of NIR light was confirmed by optical analysis of dentin and enamel. The NIR transmittance surge in the 800–1200 nm window could improve sealing of complex and deep caries lesions.
Significance
We demonstrate faster curing and an improved degree of polymerization by using upconversion filler particles as multiple light emission centers. This study represents an alternative approach in curing dental resins by NIR source.
1
Introduction
Light-curing resins have been widely used in restorative dentistry for several decades . These resins are acrylate based and can be polymerized by blue light. They consist of a viscous bisphenol-A glycidyl dimethacrylate (BisGMA), a reactive diluent (triethyleneglycol dimethacrylate, TEGDMA) and a photo-initiator such as camphorquinone . The advantage of light-curing over chemical curing in dental applications is that the process is easily controllable and fast. Furthermore, light-curing resins are frequently used in adhesive dentistry to bond ceramic restorations such as inlays, overlays, or crowns to dental hard tissues. However, despite widespread application, these resins suffer from shrinkage and incomplete curing of the resin . The application thickness per treatment is limited by the blue light penetration through dental hard tissue and the polymer itself. Current clinical practice, therefore, relies on layer-by-layer filling of the restorative polymers into a cavity ( Fig. 1 ). The multistep layer-by-layer treatment compels advanced skills in restoration procedures from a dentist and involves long treatment time at considerable costs. Layer-by-layer technique often requires destruction of healthy tooth tissues to provide complete exposure of the restorative toward blue light.

In the present work, we therefore investigate a new way to avoid problems inherent to conventional light-curing dental resins. Deep tissue penetrating near infrared light (NIR) and upconversion (UC) phosphors (a specific class of materials that allows to combine several NIR photons and emit a blue light photon, for review see ) can generate the necessary light inside the polymer to homogeneously harden a restorative resin in the tooth. Different energy of the incident NIR irradiation avoids the blue light absorbance loss problem of current dental treatments ( Fig. 2 a and b).

NIR has recently attracted tremendous interest when dealing with low radiation penetration depth in living tissue . Non-invasive excitation with NIR light has a higher transmittance in the so-called “therapeutic window” at 800–1200 nm . Following such approach, we demonstrate that a clinically relevant dental resin can be cured using NIR light in one step behind various dental hard tissue barriers.
2
Materials and methods
2.1
Preparation of NaYF 4 :Yb,Tm particles
The blue emitting hexagonal UC phosphors were prepared by solid state synthesis in the group of Karl Krämer at the University of Bern. Briefly, the rare earth fluorides (YF 3 , TmF 3 and YbF 3 ) were prepared from corresponding oxides by treating them with HF. Sintering the stoichiometric mixtures of derived salts at 550 °C in HF/Ar gas stream resulted in hexagonal NaYF 4 host matrix doped with Yb and Tm. The phase composition was analyzed by X-ray diffraction (XRD) recorded on an X’Pert PRO-MPD (PANalytical Netherlands, Cu Kα radiation, X’Celerator linear detector system, step size 0.033°, under ambient conditions). The derived UC particles were afterwards grounded and analyzed according to protocol ( Fig. 3 a and b).

2.2
Preparation of Heliobond ® /NaYF 4 :Yb,Tm composites
Polymeric matrix consisted of clinically approved ingredients: adhesive mixture of TEGDMA and BisGMA (40/60%) with camphorquinone as an initiator in Heliobond ® (Ivoclar, Vivadent, Liechtenstein) . Powders of UC phosphors were premixed with Heliobond ® under different ratios (0/100, 10/90 or 20/80) using a “speed mixer” (Hauschild Eng. DAC 150 FVZ Germany, 3000 rpm – rates per minute) during 30 s.
Then the samples were molded in cylinders (Ø1 mm) of different thicknesses (1–10 mm). Finally, the premixed and molded specimens were exposed to a NIR laser ( λ = 980 ± 5 nm, continuous wave, CNI, China) for intervals from 30 s to 5 min. For comparison, commercial non-filled polymeric samples were cured with blue light ( λ = 450 nm, 550 mW cm −2 , Optilux 500, Kerr, USA) for 60 s, following the manufacturer’s specification.
To evaluate the curing time of pure polymer and composite samples, a needle was manually pressed through the surface of the sample. The absence of a visible needle penetration proved that the sample was hardened.
Scanning electron microscope (SEM; LEO 1530 Gemini, Zeiss, Germany) images characterized the morphology and particles’ distribution in the UC phosphor/polymer composites.
2.3
Absolute UC luminescence spectroscopy
Absolute UC luminescence spectra were measured using an integrating sphere ( Fig. 3 c) following a method described by Ivanova et al. . NIR excitation radiation was delivered through a Ti:sapphire laser pumped by a continuous wave argon ion laser (Coherent Innova 90, USA) and focused on an integrating sphere containing the sample. The excitation power was controlled by a photodiode and adjusted using neutral density filters. The total UC luminescence was collected in a Spectralon™ sphere (Labsphere Inc., USA) with 5 cm diameter (AvaSphere, Avantes BV, Netherlands). The 1 cm diameter sample port was covered with Spectralon™. Emitted light was transmitted by an optical fiber to a spectrometer (AVASpec-1024TEC). The setup (integrating sphere and spectrometer) was calibrated by a halogen tungsten lamp (10 W, fan-cooled Avalight-HAL, Avantes BV, Netherlands).
2.4
Upconversion phosphors preparation and characterization
The UC phosphors were grounded in ball-mill (Pulverisette 7, Tracomme, Switzerland) (10 mm ZrO 2 balls; 1:1 weight ratio) in isopropyl alcohol (technical grade) during 1–5 cycles for 30 min. The hydrodynamic particle size distribution was measured (2 wt.% UC powder in ethylene glycol) using an X-ray disk centrifuge particle size analyzer (Brookhaven Instruments, USA).
2.5
Polymer conversion rate
Cured samples ( n = 10) were ground into fine powders by 200 μm corn sandpaper and then mixed with KBr powder (2 wt.%). The absorbance peaks were measured using diffuse reflection in a Fourier transform infrared spectrometer (FTIR, Tensor 27, Bruker Optics, 500–4000 cm −1 wavelength range, resolution 2 cm −1 , 64 scans per sample). Non-cured polymer samples were examined with a Bruker Optics Vertex 70v FTIR spectrometer (500–4000 cm −1 wavelength range, resolution 2 cm −1 , 64 scans per sample).
The degree of conversion of each composite ( n = 3) was determined from the ratio of the absorbance intensities of C C (peak at 1638 cm −1 ) to one of the C⋯C (peak at 1608 cm −1 ) which measures the monomer content before and after curing :
DC ( % ) = 1 − [ ( C = C ) / ( C ⋯ C ) ] after curing [ ( C = C ) / ( C ⋯ C ) ] before curing × 100 %
2.6
NIR and blue light transmittance of teeth
Bovine enamel and dentin cross-sections of 0.5 mm and 1 mm thickness were analyzed for light transmission. The specimens ( n = 5) were stored in water at 5 °C prior to analysis. Samples were examined under dry and wet conditions using an UV–Vis–NIR spectrophotometer (Cary 6000i, Varian, USA).
2.7
Temperature rise
Tooth chamber temperature rise upon curing with NIR laser was measured by K-type thermocouple (Thermocoax, Suresnes, France), which was placed in the prepared cylindrical cavities (1 mm × 3 mm) at ambient conditions ( T = 25 °C). The thermocouple was placed at 1 and 2 mm distance from the cavity filled with resin samples ( n = 10) ( Fig. 4 a).

2.8
Data analysis
All data are stated as mean values ± standard deviation. Statistical significance ( p < 0.05) between pure polymer and UC phosphors/polymer composite was evaluated by Student’s t -test for curing performance experiments. Results were taken as significant at p < 0.05 for optical parameters for dentin and enamel.
2
Materials and methods
2.1
Preparation of NaYF 4 :Yb,Tm particles
The blue emitting hexagonal UC phosphors were prepared by solid state synthesis in the group of Karl Krämer at the University of Bern. Briefly, the rare earth fluorides (YF 3 , TmF 3 and YbF 3 ) were prepared from corresponding oxides by treating them with HF. Sintering the stoichiometric mixtures of derived salts at 550 °C in HF/Ar gas stream resulted in hexagonal NaYF 4 host matrix doped with Yb and Tm. The phase composition was analyzed by X-ray diffraction (XRD) recorded on an X’Pert PRO-MPD (PANalytical Netherlands, Cu Kα radiation, X’Celerator linear detector system, step size 0.033°, under ambient conditions). The derived UC particles were afterwards grounded and analyzed according to protocol ( Fig. 3 a and b).
2.2
Preparation of Heliobond ® /NaYF 4 :Yb,Tm composites
Polymeric matrix consisted of clinically approved ingredients: adhesive mixture of TEGDMA and BisGMA (40/60%) with camphorquinone as an initiator in Heliobond ® (Ivoclar, Vivadent, Liechtenstein) . Powders of UC phosphors were premixed with Heliobond ® under different ratios (0/100, 10/90 or 20/80) using a “speed mixer” (Hauschild Eng. DAC 150 FVZ Germany, 3000 rpm – rates per minute) during 30 s.
Then the samples were molded in cylinders (Ø1 mm) of different thicknesses (1–10 mm). Finally, the premixed and molded specimens were exposed to a NIR laser ( λ = 980 ± 5 nm, continuous wave, CNI, China) for intervals from 30 s to 5 min. For comparison, commercial non-filled polymeric samples were cured with blue light ( λ = 450 nm, 550 mW cm −2 , Optilux 500, Kerr, USA) for 60 s, following the manufacturer’s specification.
To evaluate the curing time of pure polymer and composite samples, a needle was manually pressed through the surface of the sample. The absence of a visible needle penetration proved that the sample was hardened.
Scanning electron microscope (SEM; LEO 1530 Gemini, Zeiss, Germany) images characterized the morphology and particles’ distribution in the UC phosphor/polymer composites.
2.3
Absolute UC luminescence spectroscopy
Absolute UC luminescence spectra were measured using an integrating sphere ( Fig. 3 c) following a method described by Ivanova et al. . NIR excitation radiation was delivered through a Ti:sapphire laser pumped by a continuous wave argon ion laser (Coherent Innova 90, USA) and focused on an integrating sphere containing the sample. The excitation power was controlled by a photodiode and adjusted using neutral density filters. The total UC luminescence was collected in a Spectralon™ sphere (Labsphere Inc., USA) with 5 cm diameter (AvaSphere, Avantes BV, Netherlands). The 1 cm diameter sample port was covered with Spectralon™. Emitted light was transmitted by an optical fiber to a spectrometer (AVASpec-1024TEC). The setup (integrating sphere and spectrometer) was calibrated by a halogen tungsten lamp (10 W, fan-cooled Avalight-HAL, Avantes BV, Netherlands).
2.4
Upconversion phosphors preparation and characterization
The UC phosphors were grounded in ball-mill (Pulverisette 7, Tracomme, Switzerland) (10 mm ZrO 2 balls; 1:1 weight ratio) in isopropyl alcohol (technical grade) during 1–5 cycles for 30 min. The hydrodynamic particle size distribution was measured (2 wt.% UC powder in ethylene glycol) using an X-ray disk centrifuge particle size analyzer (Brookhaven Instruments, USA).
2.5
Polymer conversion rate
Cured samples ( n = 10) were ground into fine powders by 200 μm corn sandpaper and then mixed with KBr powder (2 wt.%). The absorbance peaks were measured using diffuse reflection in a Fourier transform infrared spectrometer (FTIR, Tensor 27, Bruker Optics, 500–4000 cm −1 wavelength range, resolution 2 cm −1 , 64 scans per sample). Non-cured polymer samples were examined with a Bruker Optics Vertex 70v FTIR spectrometer (500–4000 cm −1 wavelength range, resolution 2 cm −1 , 64 scans per sample).
The degree of conversion of each composite ( n = 3) was determined from the ratio of the absorbance intensities of C C (peak at 1638 cm −1 ) to one of the C⋯C (peak at 1608 cm −1 ) which measures the monomer content before and after curing :
DC ( % ) = 1 − [ ( C = C ) / ( C ⋯ C ) ] after curing [ ( C = C ) / ( C ⋯ C ) ] before curing × 100 %
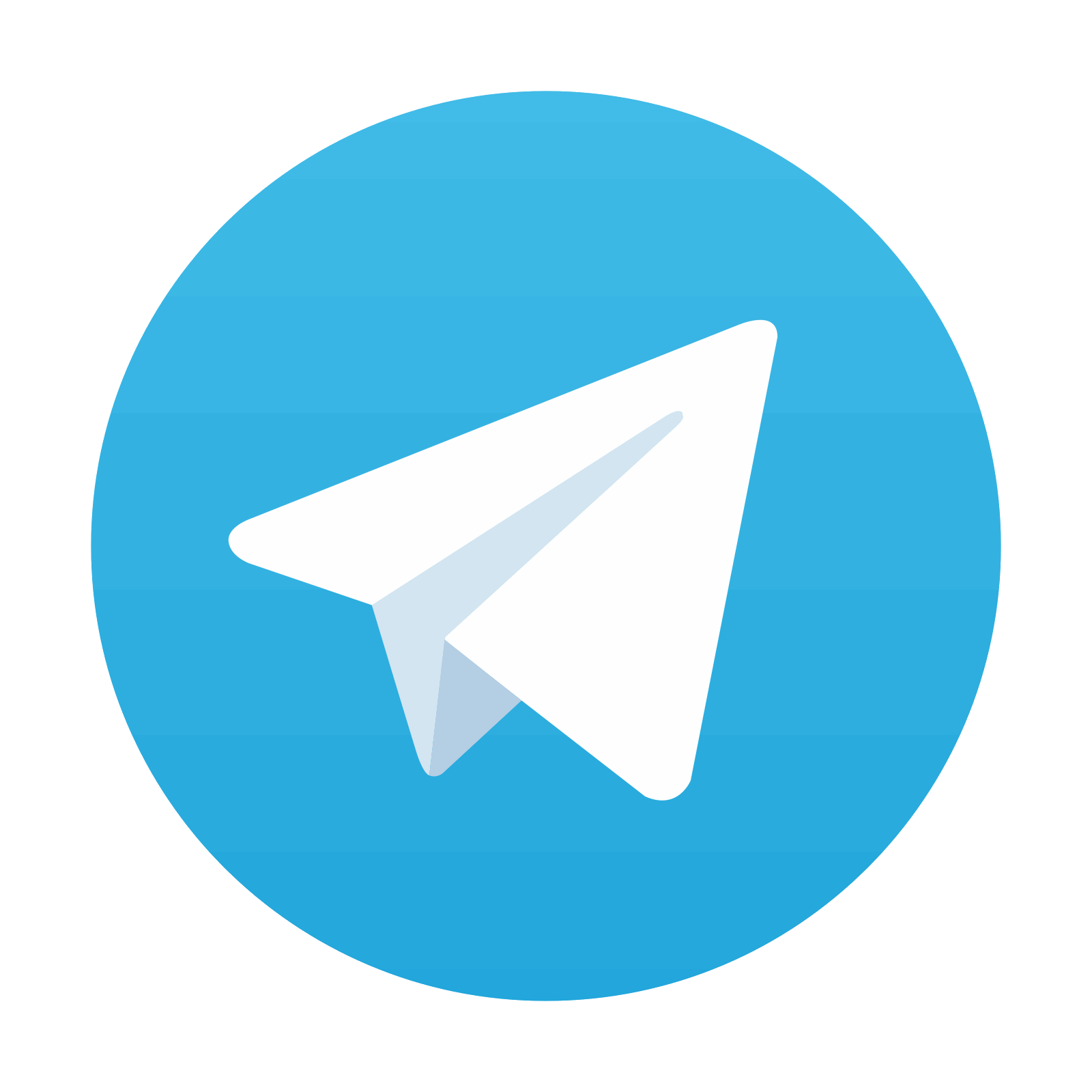
Stay updated, free dental videos. Join our Telegram channel

VIDEdental - Online dental courses
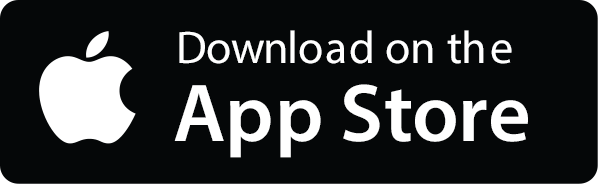
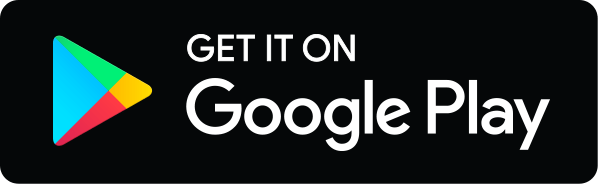