Abstract
Objectives
To test the null hypotheses that photoactive resin composites containing a Type I photoinitiator would exhibit reduced DC or increased monomer elution at substantially short curing times compared with materials based on a Type 2 ketone/amine system.
Methods
Two experimental resin composites were prepared, using either Lucirin-TPO or camphorquinone/DMAEMA. Specimens were light-cured using appropriate spectral emission that coincided with the absorption properties of each initiator using different irradiation protocols (0.5, 1, 3, 9 s at 500, 1000 and 2000 mW/cm 2 for Lucirin-TPO based composites and 20 or 40 s at 1000 mW/cm 2 for Lucirin-TPO and camphorquinone-based composites). Degree of conversion (DC) was measured by Raman spectroscopy, propagating radical concentrations were collected by means of electron paramagnetic resonance (EPR) and monomer leaching was characterized using high-performance liquid chromatography (HPLC).
Results
The null hypotheses were rejected, except for a single irradiation protocol (0.5 s @ 500 mW/cm 2 ). Lucirin-TPO-based composites could cure 20 times faster and release at least 4 times less monomers in comparison to camphorquinone-based composites. At 1000 mW/cm 2 , and 1 s irradiation time for curing times of 1 s, Lucirin-TPO based composites displayed 10% higher DC. The difference in polymerization efficiency of Lucirin-TPO compared with camphorquinone-based resin composites were explained using EPR; the former showing a significantly greater yield of radicals which varied logarithmically with radiant exposure.
Significance
Lucirin-TPO is substantially more efficient at absorbing and converting photon energy when using a curing-light with an appropriate spectral emission and otherwise a limitation noted in several previous publications. At concentrations of 0.0134 mol/L, Lucirin-TPO-based composites require a minimum light intensity of 1000 mW/cm 2 and an exposure time of 1 s to provide significantly improved DC and minimal elution compared with a conventional photoinitiator system. The use of a wide range of curing protocols in the current experiment has realized the significant potential of Lucirin-TPO and its impact for clinical applications, in replacement to materials using camphorquinone.
1
Introduction
The degree of conversion (DC) of dental resin-based composites is a key physical property of the polymer since functional material characteristics, such as mechanical properties, volumetric shrinkage, wear resistance and monomer elution are significantly correlated . Incomplete monomer conversion is a well-known drawback of dental resin composites, and the resulting monomer release could at least partially account for failures observed clinically, including persistant post-operative sensitivity and/or the need for endodontic treatment, as well as secondary caries . Further, the release of un-reacted monomers was suggested to be responsible for undesirable biological responses, such as cytotoxicity or disturbance of pulp progenitor cells differentiation even at non-toxic concentrations . Therefore, incomplete polymer conversion and the increased potential for monomer release is likely to affect the delicate balance between healing and chronic inflammation in damaged pulp tissues . Moreover, sub-optimal polymerization of resin composites may promote bacterial colonization at its surface . It has also been suggested that release of degradation by-products from the monomers in dental resin composites can potentially influence biofilm formation and microbial survival at the material surface .
Clinically, the most common strategy to maximize DC and minimize monomer elution is to provide sufficient energy to the system by increasing curing time. Several works focusing on commercially available composites have emphasized the need to apply at least 20 s, but more likely 40 s irradiation to minimize the amount of eluted substances, even with the use of high irradiance LED lights . As stated by Polydorou et al. , even 40 s irradiation “seems insufficient in order to prevent a high release of monomer”. Currently, the general consensus in the clinics to light-cure a 2 mm layer of CQ-based commercial composites is 20 s at about 1000 mW/cm 2 with commercial LED polywave lights . The industry is challenged to combine improvement of DC and decrease of monomer elution with a curing time as short as possible to satisfy the demands of a time-conscience dental practitioner. One interesting approach to significantly reduce curing time (3 s) while reaching similar or improved DC is to replace the conventional camphorquinone/amine system (CQ) (Type II initiator) with a Norrish Type I monoacylphosphine oxide photoinitiator, namely Lucirin-TPO (TPO) . In a subsequent paper by the same group, it was reported the increased reaction speed did not lead to any increase in shrinkage stress, cusp deflection or marginal leakage, but significantly improved mechanical properties . However, the impact of replacing a Type 2 with a Type 1 photoinitiator with regards to monomer elution has not been investigated to date.
Hence, the aim of the present study was to test the null hypotheses that at very short curing times, TPO-based resin composites would not exhibit any difference with regard to (1) DC or (2) monomer elution compared to a conventional CQ-based experimental composite.
2
Materials and methods
2.1
Materials
Two experimental resin composites were prepared, based on a 70/30 wt% Bis-GMA/TegDMA resin (Sigma Aldrich), using two different photoinitiator systems in equimolar concentrations (0.0134 mol/L): either camphorquinone and dimethylaminoethyl methacrylate 0.2/0.8 wt% (Sigma Aldrich), or Lucirin-TPO 0.42 wt% (BASF), subsequently referred as CQ and TPO, respectively. CQ concentrations were chosen in order to be within the region of maximum DC according to Yoshida and Greener . The corresponding equimolar concentration of TPO also falls within the range described as adequate with regards to DC, since Miletic and Santini reported that any concentration in excess of 0.43 wt% resulted in only very small increase in DC values . Hence, the concentration of both photoinitiators can be considered to be in the optimal range, which enables the comparison of both initiating systems in optimal conditions.
The following fillers were introduced to the resin matrix: silanated barium glass (G018-186/K6, d 50 = 3 ± 1 μm, Schott AG, Germany) and methacrylsilane treated fumed silica (12 nm, Evonik Industries, Germany) at 65 and 10 wt%, respectively. This is summarized in Table 1 .
Composite | Monomers (ratio) | Photoinitiators | Fillers |
---|---|---|---|
CQ | Bis-GMA: 70 wt% | Camphorquinone: 0.2 wt% a | Micro/nano (65/10 wt%) b |
TegDMA: 30 wt% | 2-(Dimethylamino)ethyl methacrylate: 0.8 wt% a | ||
TPO | Bis-GMA: 70 wt% | Lucirin: 0.42 wt% a | Micro/nano (65/10 wt%) |
TegDMA: 30 wt% | |||
Bis-GMA—bisphenol A glycol dimethacrylate | |||
TegDMA—triethyleneglycol dimethacrylate | |||
Micro—silanated barium glass Ø 3 μm | |||
Nano—methacrylsilane treated fumed silica Ø 12 nm |
a Percentage of monomer content only.
b Percentage of total composite mass, including monomer and photoinitiator.
2.2
Light curing
Specimens were polymerized in Teflon moulds of 2 mm thickness and 5 mm diameter, covered on each side by Mylar films, resting on a glass slide. For the EPR measurements, rectangular specimens of dimensions 5 × 2 × 2 mm were prepared, also in Teflon moulds. Light-curing was carried out with an AURA light device (Lumencor, USA) providing narrow spectral outputs. Irradiation could be applied independently at two wavelength ranges: 395–415 nm and 455–485 nm for TPO and CQ-based composites, respectively as shown in Fig. 1 . Light irradiance calibrations were carried out directly at the tip of the optic fiber light guide (6 mm diameter) with good linear fit ( R 2 > 0.98), using a calibrated and NIST traceable deuterium tungsten light source (DH2000-CAL, Ocean Optics, Dunedin, USA). Calibration and spectral irradiance measurements were performed using a spectrophotometer (USB4000, Ocean Optics) with an 8 μm diameter fiber connected to a 3.9 mm opaline glass cosine corrector aligned concentrically with the light guide of the curing light device. Light irradiance in each range could be varied from 60 to 3240 mW/cm 2 and 30 to 1360 mW/cm 2 , for TPO and CQ-based resin composites, respectively. Light curing was carried out with the light guide tip in direct contact with the upper Mylar film. As mentioned above, while 20 s are recommended in clinical practice, it is safer in terms of monomer elution to use 40 s for CQ-based materials. For these reasons, controls in the present work were experimental CQ-based composites light-cured at 1000 mW/cm 2 for 20 and 40 s. However, TPO-based experimental composites may require much shorter irradiation times and were cured at 2000 mW/cm 2 for 9 s, 3 s, 1 s or 0.5 s, 1000 mW/cm 2 for 40 s, 20 s, 9 s, 3 s, 1 s or 0.5 s and 500 mW/cm 2 for 9 s, 3 s, 1 s or 0.5 s. This is summarized in Table 2 . Irradiation time was adjusted using an automatic sequence mode available in the controlling software which allowed for flashes as short as 0.2 s with precise execution. Radiant energy (J/cm 2 ), defined as the product of irradiance (W/cm 2 ) and irradiation time (s) ranged from 0.25 to 40 J/cm 2 and 20 to 40 J/cm 2 for TPO and CQ-based composites, respectively ( Table 3 ).
Composite type | I irr (mW/cm 2 ) | t irr (s) |
---|---|---|
CQ | 1000 | 40 and 20 |
TPO | 2000 ( H irr ) | 9, 3, 1 and 0.5 |
1000 ( M irr ) | 40, 20, 9, 3, 1 and 0.5 | |
500 ( L irr ) | 9, 3, 1 and 0.5 |
Curing time (s)/irradiance (mW/cm 2 ) | Radiant exposure (J/cm 2 ) | Bis-GMA (wt/wt%) | TegDMA (wt/wt%) | DC (%) | |
---|---|---|---|---|---|
TPO | 0.5/500 | 0.25 | 25.5 A (2.2) | 18.4 D (1.9) | 51 (3) UPPER |
38 (6) LOWER | |||||
1/500 | 0.5 | 8.2 B (0.9) | 4.5 E (0.4) | 57 (2) UPER | |
51 (2) LOWER | |||||
3/500 | 1.5 | 2.4 C (0.4) | 0.4 F (0.1) | 59 (4) | |
9/500 | 4.5 | 1.6 C (0.1) | b.l | 60 (4) | |
0.5/1000 | 0.5 | 9.1 B (1.8) | 5.1 E (1.1) | 62 (0.4) UPPER | |
55 (2) LOWER | |||||
1/1000 | 1 | 2.9 C (0.9) | 0.7 F (0.3) | 64 (4) | |
3/1000 | 3 | 1.3 C (0.3) | b.l | 64 (2) | |
9/1000 | 9 | 1.3 C (0.3) | b.l | 62 (3) | |
20/1000 | 20 | 1.2 C (0.2) | b.l | 62 (3) | |
40/1000 | 40 | 1.3 C (0.2) | b.l | 63 (2) | |
0.5/2000 | 1 | 2.7 C (0.4) | 0.8 F (0.3) | 57 (5) | |
1/2000 | 2 | 1.4 C (0.1) | b.l | 59 (2) | |
3/2000 | 6 | 1.2 C (0.2) | b.l | 60 (4) | |
9/2000 | 18 | 1.2 C (0.3) | b.l | 58 (4) | |
CQ | 20/1000 | 20 | 7.2 B (1.9) | 3.6 E (1.1) | 51 (3) |
40/1000 | 40 | 6.8 B (1.3) | 3.2 E (0.7) | 51 (3) |
2.3
Degree of conversion
DC was measured by Raman spectroscopy, carrying out three measurements for each sample surface (upper and lower), at least 20 min following irradiation ( n = 3). The equipment used was a Raman Spectrometer (DXR Raman Microscope, Thermo Scientific, Madison, WI, USA), with a 1 μm spatial resolution and 3 cm −1 spectral resolution. The samples were excited at 780 nm by a frequency-stabilized single mode diode laser through a microscope objective (×50) with the following conditions: microhole, 50 μm; irradiation time, 60 s; number of accumulations, 5; grating 400 lines/mm. The DC was then calculated as the percentage of vinyl functions converted to aliphatic functions based on the decrease in the C=C bond peak at 1640 cm −1 compared to the uncured sample; the aromatic C=C peak at 1610 cm −1 was used as the internal standard.
2.4
Free radical concentration
Post-curing signals from trapped free radicals were analyzed using a MINISCOPE MS200 EPR spectrometer (Magnettech, Germany), equipped with a rectangular cavity (TE102) with parameters previously described . Briefly, the height of the fourth peak (from 336.1 to 336.6 mT of the magnetic field, g-factor 2.02) was measured after 5 min, 1 h, 24 h, 1 week and 1 month after irradiation ( n = 3) to determine the relative concentration of trapped propagating radicals.
2.5
Monomer elution
Immediately after light curing, specimens were weighed and placed in 10 mL of 75/25 vol% ethanol/water solution. Elution medium was prepared using absolute ethanol NORMAPUR ® (purity 99.8%, CAS: 64-17-5, VWR) and Milli- Q ® water (Millipore, Merck). 1 mL eluates were taken for HPLC analysis at 1 h, 6 h, 24 h, 72 h and 1 week ( n = 3), each removed volume being immediately replaced by an equivalent amount of fresh ethanol/water solution. Specimens were stored in the dark at 37 °C between measurements.
Analysis by reverse phase HPLC was carried out on a Hitachi D-7000 module equipped with a Waters Symmetry C18 3.5 μm column of dimensions 4.6 × 75 mm, along with a Hitachi L-7455 Diode Array Detector. Analysis was carried out at 210 nm for both monomers. Solvents used were water (H 2 O, A) and acetonitrile (ACN, B) of HPLC grade. Mixtures of A and B were applied as follows: gradient mode, flow rate of 1.2 mL/min, starting at 100:0 (A:B), maintained for 1 min, then decreased to reach 0:100 in 6 min, then maintained for 1 min.
Calibration curves were determined for both monomers introduced in the composites (Bis-GMA, and TegDMA) by measuring absorption peak areas at different concentrations (from 10 −2 to 10 4 μg/mL). Bis-GMA produced several noticeable peaks. Commercial monomers contain a variety of isomers and by-products from synthesis and hence may release different components (Y. Imai, 2000). For quantification of Bis-GMA, the most intense peak noted was selected since the supplier indicated a 92% purity level. In both calibrations, the goodness of fit was excellent ( R 2 = 0.998 and 0.999 for Bis-GMA and TegDMA, respectively). The entire specimen surfaces were considered to be exposed to the elution fluid (0.12 cm 2 ). Following the recommendations of Van Landuyt et al. , amounts leached were expressed in terms of relative amounts of their initial content (wt/wt, in %). Quantities released were referred to as Q Bis-GMA and Q TegDMA .
2.6
Statistical analysis
The JMP 8 software was used for all statistical analyses. These were performed on DC (individual and average: mean of DC of upper and lower surfaces), propagating radical concentration, Q Bis-GMA and Q TegDMA , using one-way analysis of variance (ANOVA) and Tukey’s test for multiple comparisons. The interactions between DC, photoinitiator type, irradiation parameters, radiant energy and time intervals (when appropriate) were studied by multivariate analysis (pairwise comparison). Sets of variables were transformed to obtain normal distributions.
2
Materials and methods
2.1
Materials
Two experimental resin composites were prepared, based on a 70/30 wt% Bis-GMA/TegDMA resin (Sigma Aldrich), using two different photoinitiator systems in equimolar concentrations (0.0134 mol/L): either camphorquinone and dimethylaminoethyl methacrylate 0.2/0.8 wt% (Sigma Aldrich), or Lucirin-TPO 0.42 wt% (BASF), subsequently referred as CQ and TPO, respectively. CQ concentrations were chosen in order to be within the region of maximum DC according to Yoshida and Greener . The corresponding equimolar concentration of TPO also falls within the range described as adequate with regards to DC, since Miletic and Santini reported that any concentration in excess of 0.43 wt% resulted in only very small increase in DC values . Hence, the concentration of both photoinitiators can be considered to be in the optimal range, which enables the comparison of both initiating systems in optimal conditions.
The following fillers were introduced to the resin matrix: silanated barium glass (G018-186/K6, d 50 = 3 ± 1 μm, Schott AG, Germany) and methacrylsilane treated fumed silica (12 nm, Evonik Industries, Germany) at 65 and 10 wt%, respectively. This is summarized in Table 1 .
Composite | Monomers (ratio) | Photoinitiators | Fillers |
---|---|---|---|
CQ | Bis-GMA: 70 wt% | Camphorquinone: 0.2 wt% a | Micro/nano (65/10 wt%) b |
TegDMA: 30 wt% | 2-(Dimethylamino)ethyl methacrylate: 0.8 wt% a | ||
TPO | Bis-GMA: 70 wt% | Lucirin: 0.42 wt% a | Micro/nano (65/10 wt%) |
TegDMA: 30 wt% | |||
Bis-GMA—bisphenol A glycol dimethacrylate | |||
TegDMA—triethyleneglycol dimethacrylate | |||
Micro—silanated barium glass Ø 3 μm | |||
Nano—methacrylsilane treated fumed silica Ø 12 nm |
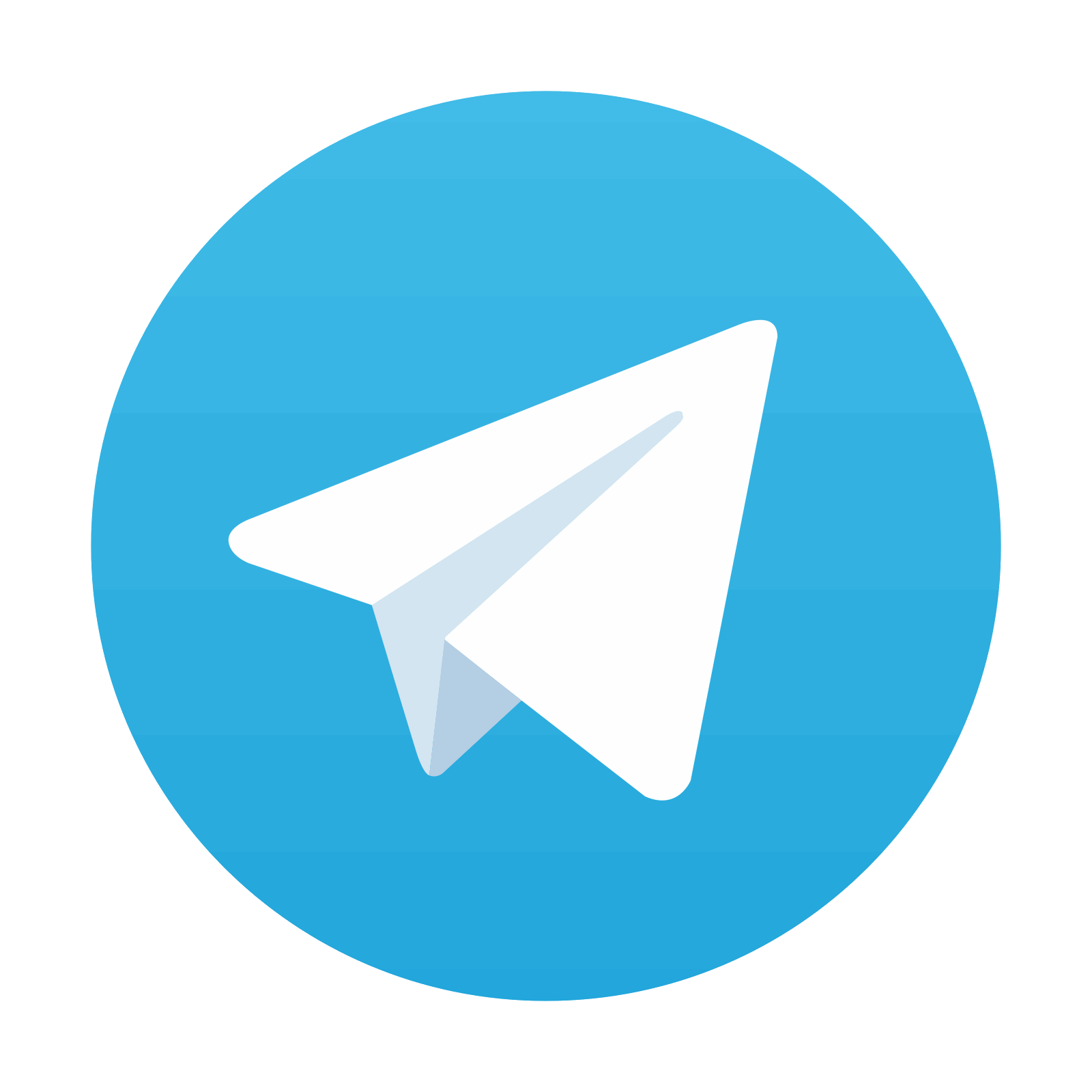
Stay updated, free dental videos. Join our Telegram channel

VIDEdental - Online dental courses
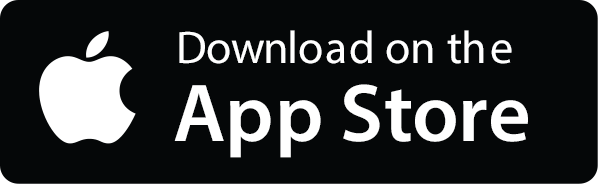
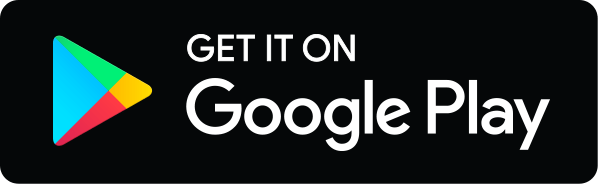
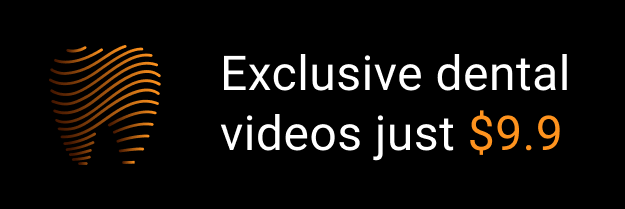