Highlights
- •
Different types of prefabricated paediatric crowns showed variant wear patterns.
- •
All self-adhesively cemented composite crowns remained intact after testing.
- •
The use of self-adhesive cements lowered wear of composite & stainless steel crowns.
- •
No impact of cementation on wear was observed for zirconia crowns.
Abstract
Objectives
The purpose of this in vitro study was to assess the two-body wear and fracture behaviour of an experimental additive manufactured composite crown in comparison to zirconia and stainless steel crowns and its cementation protocol for primary molars.
Material and methods
Three different paediatric crowns – experimental composite crowns (CCs, 3M), zirconia crowns (ZCs, NuSmile), and stainless steel crowns (SSCs, 3M)–were cemented with an experimental resin-modified glass ionomer cement (RMGIC, 3M) and two self-adhesive cements (SACs; RelyX Unicem Automix 2, 3M; BioCem, NuSmile). Seven groups, each with eight specimens, were thermally cycled (55 °C/50 °C) and dynamically loaded (50N/ 1.2Hz) in a masticatory simulator with steatite antagonists. The areal and volumetric material loss of all specimens before and after 1,200,000 masticatory cycles was evaluated with a 3D profilometer. Light and scanning electron microscopy were used for qualitative analysis. Pairwise comparisons between all the groups were performed using the Mann–Whitney U test (p < 0.05).
Results
Microscopic imaging revealed different wear patterns for each material. Lowest fracture rates were documented for the CCs. In contrast, all the SSCs showed perforations. The CCs cemented with RMGIC showed the highest significant volumetric wear (6.3 ± 0.72 mm³), followed by the SSCs cemented with RMGIC (3.6 ± 1.79 mm³) and CCs cemented with SAC (3.5 ± 1.92 mm³). No significant differences were found in terms of the wear among all the other groups, ranging between 0.4 ± 0.25 and 0.6 ± 0.32 mm³.
Conclusion
The volume loss of the tested crowns differed for each material and was dependent on the type of cementation. With regard to in vitro wear and fracture patterns, cementation with SAC may increase the clinical performance of CC paediatric crowns.
1
Introduction
Prefabricated paediatric crowns are frequently used to restore primary molars with severe carious destruction due to their less technique-sensitive clinical application technique and excellent longevity [ ]. The documented annual failure rates for stainless steel crowns (SSCs) were found to be in the range of 0–14%, which was lower than most other restorative techniques [ , ]. While the success of prefabricated SSC is well shown, the lack of aesthetics has been increasingly questioned by caregivers in daily dental practice. Therefore, veneered stainless steel or full zirconia crowns were introduced in the dental market. However, veneered SSCs have the disadvantage that ceramic veneers may chip off or, in the case of zirconia crowns, tooth preparation prior to crown placement is much more invasive than that required for placing SSCs [ ]. Nevertheless, aesthetic paediatric crowns also show high 3-year retention rates of 76–94% [ , , ]. Aiming to overcome the above mentioned limitations, a new experimental composite crown (CC) based on a homogeneous methacrylate resin matrix with silica/ zirconia nanocluster filler particles was recently developed (3M, Seefeld, Germany) due to parental request for tooth-coloured restorations [ ]. The major advantage of using CC might be the beneficial bonding to the composite-based luting cements. Further, an easier dental handling in terms of occlusal corrections and no material chip-off can be assumed compared to that of conventional SSCs, pre-veneered paediatric crowns or Zirconia crowns (ZC).
Crowns are industrially manufactured in additive technology, which has recently gained importance alongside subtractive manufacturing due to the feasibility of efficiently fabricating complex geometries in a minimum amount of time and saving material [ ]. Until recently, there have been no scientific data available about the laboratory and clinical performance of this new and innovative paediatric crown. Therefore, the primary objective of this in vitro study was to preclinically evaluate the two-body wear and fracture behaviour of additively manufactured CCs in comparison with different available paediatric crowns and cementation procedures. The initially formulated null hypothesis was that there would be no difference in terms of the fracture and wear resistance for the chosen paediatric crowns and their cementation protocols.
2
Materials and methods
2.1
Study design
This in vitro study on the two-body wear and fracture behaviour included three different pre-fabricated, paediatric crowns (CC, SSC, ZC) which were not individualised during cementation.
Composite crown (3M): methacrylate based resin matrix with silicia/zirconia nanocluster)
Zirconia crown (Nusmile): 80–96% ZrO 2 , 4–10% Y2O3, <5% HfO2, <5% organic binder, 1–4% pigments) = Y-TZP dental Zirconia.
Stainless steel crown (3M): Fe-Ni-Cr
which were cemented using two self-adhesive cements (SAC) and a resin modified glass-ionomer cement (RMGIC) on standardized tooth stumps.
2.1.1
Standardization and fabrication of standardized test dies
Considering the non-availability of appropriate and sufficient material for human teeth, a cavitated primary molar (tooth 75) was reconstructed initially and a 3D scan of a natural human tooth was preformed afterwards, which was later digitally optimized. Subsequently, three duplicates of this primary molar were milled from a composite resin Lava Ultimate block (3M) due to the material properties which are comparable to dentin [ , ]. Next, each of these fabricated duplicates were prepared according to requirements for the included crown types. In detail, CC and ZC needed an occlusal reduction of approximately 1.5 mm, proximal separation (∼1.5 mm), circumferential reduction of approximately 1 mm and shaping/ bevelling of all the edges until the selected crown passively fitted exactly over the prepared duplicate tooth stump. SSC required an occlusal reduction (∼1.5 mm) and proximal separation (∼1.5 mm). Following this, the prepared tooth stumps for each crown type were also reproduced using the previously mentioned CAD/CAM-based workflow. In total, 56 standardized tooth stumps (CCs = 16, ZCs = 24, SSCs = 16) were milled from Lava Ultimate blocks (3M), and each specimen was embedded in cold-curing methyl methacrylate resin (Technovit 4004, Heraeus Kulzer, Wehrheim, Germany) which was chosen because of its module of elasticity (2.000–2.300 N/mm 2 ) closely to human bone (spongiosa: 1.000 N/mm 2 ; corticalis: 10.000 N/mm 2 ), according to the mounting requirements for the two-body wear simulation [ ]. Before crown cementation, each tooth stump was cleaned with airborne particles with aluminium oxide (<1 bar and particle size ≤50 μm), steamed off and the specimens were dried with compressed water- and oil-free air. Finally, all tooth stumps were degreased with iso-propanol before cementation.
2.1.2
Cementation of crowns using self-adhesive cement 1 (SAC-1; RelyX Unicem 2 Automix)
Then, the crown was cemented as follows: (1) excess material was removed; (2) the crown was cured for 5 s (s) after it was installed and excess material was removed from each crown; and (3) the crown was light cured for 10 s on each of the buccal, oral and occlusal surfaces (Elipar S10, 3M). Immediately after polymerization, all the specimens were stored in distilled water at 37 °C for 24 h (Jouan EU3, innovens Ovens, ThermoFisher Scientific Inc., Waltham, MA, USA).
2.1.3
Cementation of the zirconia crowns using self-adhesive cement 2 (SAC-2; NuSmile BioCem. NuSmile)
In addition, zirconia crowns were cemented with the specifically recommended luting cement according to the manufacturer’s instructions. In detail, excess material was removed after flash curing (<5 s), then the crowns were additionally light cured for 10 s on the buccal and oral surfaces, and all the specimens were also stored in distilled water at 37 °C for 24 h.
2.1.4
Cementation of the crowns using experimental resin-modified glass ionomer cement (Exp. RMGIC, 3M)
The experimental RMGIC is composed of methacrylated polycarboxylic acid, HEMA, ethyl acetate, FAS glass, non-reactive zirconia silica filler, titanium dioxide, potassium persulfate and a photoinitiator. Subsequently to automixing each crown was filled with the experimental RMGIC and fixed over the tooth stump. Light curing and removal of excess material was performed similar to the SAC-1 group.
2.1.5
Two-body wear testing
Specimens and antagonists were mounted in a chewing simulator (CS-4, SD Mechatronic, Feldkirchen-Westerham, Germany). The cyclic fatigue test was applied to each crown with a round steatite sphere with a diameter of 4 mm. The sphere was placed onto the vestibular cusp, and the dynamic loading contained an additional horizontal shift of 2 mm in the central direction of the abutment. A force of 50 N was applied for 1,200,000 cycles at a frequency of 1.2 Hz. The loading speed was 20 mm/s, and the lifting speed was 60 mm/s. Cyclic loading was examined every ∼100,000 cycles to evaluate the possible destruction or failure of the specimen. While the mechanical force was applied, thermocycling was simultaneously conducted by changing the water temperature every 30 s from 5 °C to 55 °C.
2.1.6
Evaluation of wear
After cementation, water storage for 24 h at 37 °C and before artificial ageing, all the specimens were optically scanned (baseline scan, Laser scanner LAS-20, SD Mechatronic). A second scan was applied after artificial ageing (test scan). Both datasets were superimposed (Geomagic Qualify 2012, Morrisville, USA) with a best-fit algorithm in an iterative approach using the equator and non-abraded areas as a reference to analyse the quantity of wear. The parameters were the volume of wear, surface of wear and maximum and mean wear depth. Failure analysis was performed with a light microscope (BMS 74956, Breukoven, Essebann, The Netherlands) to detect and verify the crack lines, (micro)perforations, fractures and/or loss. In addition, specimens were further examined with a scanning electron microscope (SEM, DSM 982, Zeiss, Oberkochen, Germany) for obtaining images of the fracture pattern. To investigate the filler dimension and distribution of the CC material, five samples were observed by SEM sputtering and after heat exposure for one minute in a flame.
2.1.7
Statistical evaluation
The descriptive and explorative analysis of the data was undertaken with Microsoft Excel and SPSS Statistics for Windows, Version 21.0.1 (SPSS Inc., an IBM Company, Armonk, NY, USA). The significance level was set at p < 0.05. Pairwise comparisons between all the groups were performed using the Mann–Whitney U test.
3
Results
The two-body wear analysis showed divergent outcomes for each of the included types of crowns and their cementation. When considering first SSCs as the most frequently used paediatric crowns, gross perforations in the occlusal contact areas were the commonly observed form of failure independent of the cement type ( Table 1 , Fig. 2 ); none of the SSCs were lost. SSCs that were cemented with SAC showed, first, less extensive perforations and, second, survived longer until perforation of all the specimens (∼700,000 cycles before perforation) in comparison to the RMGIC (∼300,000). Contrary to the previously mentioned observation, brittle fractures were found to be the common fracture mode for SAC cemented ZCs ( Table 1 , Fig. 2 ); only one ZC (SAC-2 cementation) fractured in full. Perforations were mostly observed in the group for RMGIC fixed ZCs. The lowest failure rate was documented for the CCs in combination with SAC. When using the experimental RMGIC, the failures of the CCs increased ( Table 1 and Fig. 2 ).
Crown type | Cementation | Light microscopic evaluation (4.5 fold) after testing | ||||
---|---|---|---|---|---|---|
Intact | Crack lines | Micro-perforation | Perforation | Fracture & Loss | ||
Stainless steel crowns (SSCs) | RelyX Unicem 2 Automix (SAC-1) | – | – | – | 8 a | – |
Experimental RMGIC | – | – | – | 8 b | – | |
Zirconia crowns (ZCs) | RelyX Unicem 2 Automix (SAC-1) | 5 | 3 | – | – | – |
BioCem (SAC-2) c | 3 | 3 | – | – | 1 | |
Experimental RMGIC* | – | 2 | – | 6 | – | |
Exp. Composite crowns (CCs) | RelyX Unicem 2 Automix (SAC-1) | 8 | – | – | – | – |
Experimental RMGIC | 1 | 2 | 4 | – | 1 |
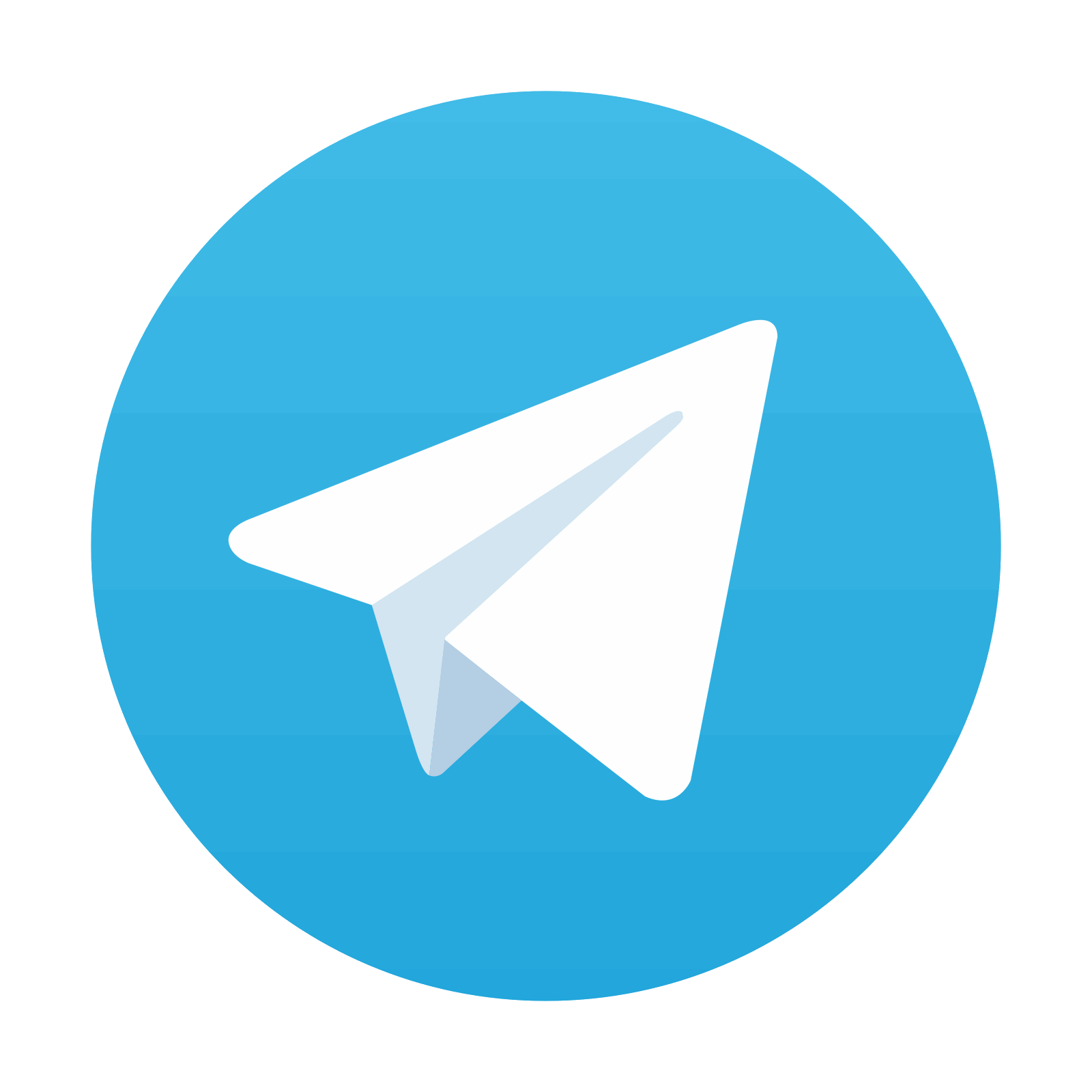
Stay updated, free dental videos. Join our Telegram channel

VIDEdental - Online dental courses
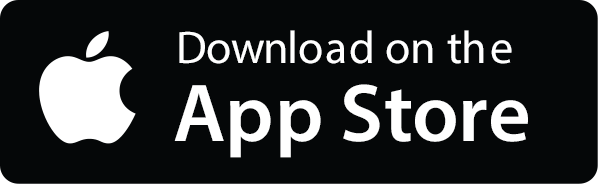
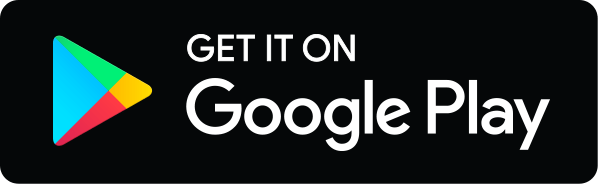
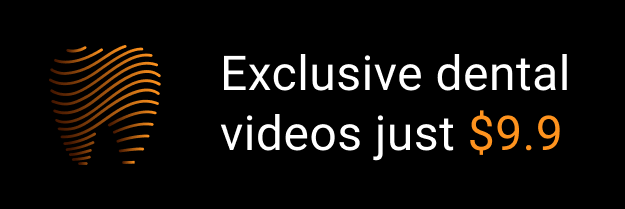