The oral and maxillofacial surgeon who offers full-spectrum treatment will likely experience nerve injuries during the course of his or her career. Acute neurosensory symptoms in lingual and inferior alveolar nerves (IANs) are present in as many as 35% of patients 1 week after routine surgeries, such as third molar removal. Although more than 90% will resolve spontaneously by 3 weeks, an important patient subset of 15% to 30% will retain abnormal sensations and impaired orofacial functions for 3 months or more, and approximately 15% of those injured will experience some level of persisting neuropathic pain. Those patients with neuropathies persisting more than 1 year after injury are considered permanent, however, and may not be surgically reversible.
Some trigeminal nerve injuries may be unavoidable because of anatomic variations and the nature of the deformities or pathologic condition requiring treatment. Especially high risks for injury to trigeminal nerves are inherent for patients treated for orofacial trauma, developmental deformities, reconstructive surgeries with endosseous implants, and, most commonly, pathologic conditions associated with third molar surgery. Unfortunately, nerve injury complications are still perceived as evidence of surgical negligence by uninformed patients.
There have been scientific advances in the diagnosis and understanding of the causes and mechanisms of traumatic neuropathies. We now have more than 2 decades of experience with surgical repairs that demonstrate satisfactory outcomes for more than half of treated nerve injury patients. We have learned that microsurgical repairs of lingual nerves are more necessary and effective than inferior alveolar repairs. Early aggressive medical and selective surgeries of acute nerve injuries may prevent transition to chronic refractory neuropathies and dysesthesias; therefore, it is recommended that patients with dysfunctional sensory deficits and intractable pain should be treated within 90 days of injury, if possible.
Results show that microsurgical repairs are likely to improve deficient sensory functions, but less likely to eliminate chronic neuropathic pain. Internal neurolysis procedures and current nerve grafting procedures have yielded disappointing outcomes, whereas microsurgical decompressions, with neuroma resection and direct reanastomoses, have yielded the most favorable results. The critical need for an effective alloplastic graft technique for use with cases of more extensive nerve tissue loss remains a research challenge.
This chapter offers six sections with information on: (1) causes, classification of nerve injuries, and determinants of nerve injury responses; (2) clinical measurement and diagnosis of trigeminal nerve injuries; (3) indications, contraindications, and techniques of surgical repair; (4) a survey of outcomes of trigeminal repairs; (5) case-specific applications for management of nerve injuries associated with facial trauma, orthognathic, anesthetic, medicament, implant, third molar-associated nerve injuries; and (6) current medicolegal issues related to nerve injuries.
▪
CAUSES, CLASSIFICATION, AND DETERMINANTS OF NERVE INJURIES
Trauma to the peripheral trigeminal nerves is a common source of orofacial dysfunction, sensory loss, and neuropathic pain. The most common causes are maxillomandibular contusions and fractures, where the inferior alveolar and infraorbital nerves are almost always involved, and chronic impairment persists in up to 35% to 50% of patients. Injuries to inferior alveolar and lingual nerves also occur during third molar surgery in approximately 1% to 4% of cases, and an estimated 13% of those injured experience permanent neuropathies. Endosseous implant surgery accounts for an increasing number of cases of mandibular nerve impairment, especially in those patients with more severe bony atrophy and in those who undergo nerve repositioning surgeries. Chronic posttraumatic neuropathies from local anesthetic injuries, although rare in comparison with the number of injections carried out, can, nevertheless, be especially painful and refractory to treatment. Similarly, nerves injured by root canal instruments or surgical medicaments may display sensory neurotoxicity that is difficult to treat. Orthognathic surgery, particularly sagittal mandibular osteotomy, has a high incidence of intraoperative nerve injuries, good levels of early spontaneous recovery, but some degree of long-term altered sensation in up to 50% of patients.
A MECHANISTIC CLASSIFICATION OF NERVE INJURIES
Sir Herbert Seddon and Sir Sydney Sunderland developed nerve injury classifications that still provide a useful framework for characterizing nerve injuries. Seddon’s hypothesis was that increasing nerve damage results in greater permanent loss of sensation. Sunderland’s scheme emphasized a progressive destruction of nerve structures with Wallerian axon degeneration and disruption of endoneurial, perineurial, and, finally, epineurial tissues. The value of combining these two classification schemes has been shown by quantitative sensory testing (QST), which has verified that the depth of trigeminal sensory loss correlates generally with increasing physical nerve destruction. The Seddon-Sunderland linear injury scales also correlate well with decreasing potential for spontaneous nerve recovery, with increasing likelihood of dysfunctional neuroma formation and with increasing need for surgical repair interventions ( Table 15-1 ).
Injury Classification | Causes | Responses | Recovery | Microsurgery |
---|---|---|---|---|
Neurapraxia (Seddon) first-degree injury (Sunderland) | Minor nerve compression stretch, thermal, acute infection | Neuritis, paresthesias, conduction block, no structural damage | Spontaneous recovery less than 2 mo | Not indicated unless foreign body preventing nerve recovery |
Axonotmesis (Seddon) second-degree injury (Sunderland) | Partial crush, traction, thermal, chemical injury, hematoma, chronic infection | Intact epineurium, isolated axon loss, episodic dysesthesia | Spontaneous recovery in 2-4 mo |
|
Third-degree injury (Sunderland) | Traction, crush, puncture thermal, chemical injury | Wallerian axon loss, some internal fibrosis, peripheral sensitization | Recovery crude senses >1 yr neuropathy, | Decompression and repair if poor function, sustained pain at 3 mo |
Fourth-degree injury (Sunderland) | Full crush, extreme stretch, high thermal, direct chemical injury | Neuroma-in-continuity, hypoesthesia, and triggered hyperpathia | Permanent injury, minimal spontaneous recovery | Repair, neuroma resection if intolerable dysfunction pain after 3 mo |
Neurotmesis (Seddon) fifth-degree injury (Sunderland) | Transection, tear, laceration avulsion of nerve trunk | Amputation neuroma, anesthetic, evolving deafferentation pain | Permanent injury, no spontaneous recovery | Neuroma resection with neurorrhaphy or graft if intolerable dysfunction and intractable neuropathic pain |
Sunderland Type 1 (Seddon “neurapraxia”) injuries result from ischemic effects of minor nerve trunk traction, mild compression from edema, hematoma, or temporary increases in local inflammation. Paraneural heat in the 48 °C to 54 °C range, generated by rotating surgical instruments, may produce Type 1 damage. The neurologic effects are primarily conduction block, resulting in slowed stimulus responses. Although isolated sites of neural demyelination may occur, Wallerian axon degeneration is not expected, and there are no grossly visible effects on the epineurium. Rapid spontaneous recovery toward normal sensation and sensory functions is expected within days to a few weeks. Microsurgery is not indicated, except for the removal of compressive paraneural foreign bodies.
Sunderland Type 2 (Seddon “axonotmesis”) injuries result from more forceful crush or abrupt nerve traction greater than 25 g. Partial compression from implants or displaced bone may induce this level of injury. QST reveals deeper loss of sensory responses, particularly for fine touch discrimination. Some degree of axon degeneration may occur, but the endoneurium, perineurium, and epineurium remain intact. Therefore axonal regeneration and sensory recovery is expected within 2 to 4 months. Extraneural hematoma and secondary scarification may be associated with this level of injury and perpetuate mild neuropathy. In such cases, surgical decompression may enhance recovery.
Type 3 Sunderland injuries are caused by more severe mechanical crush, puncture, chemical, and thermal traumas and result in Wallerian degeneration at the injury site and some degree of cell loss in the trigeminal ganglion. The endoneurium is also damaged, and some intraneural bleeding and scarification may potentially interfere with complete axon recovery. For this reason, this injury level may be associated with permanent neurosensory disturbances. Painful burning paresthesias and hyperphenomena may be seen within hours of Type 2 and Type 3 injuries and may prompt surgical exploration and repair if sensory test responses fail to show satisfactory recovery within 3 months.
Type 4 Sunderland injuries result from extreme nerve crush, thermal effects above 55 °C, intraneural local anesthetic injections, or caustic medicaments in contact with the nerve. The epineurium is grossly intact, although contused, and may be devascularized. Internal nerve structures are damaged and replaced by disorganized scar tissue. Trigeminal ganglion cell necrosis occurs, and central deafferentation effects can be measured as high as thalamic levels, resulting in centralized and spontaneous pain syndromes along with severe peripheral sensory loss. QST reveals deep hypoesthesia to all stimuli, and percussion of the injury site may elicit a hyperpathic aching pain and paresthesia. Neuromain-continuity has formed in such cases, and microsurgical resection and repair will be required if sensory reflex recovery is to be improved.
Sunderland Type 5 (Seddon “neurotmesis”) is the most extreme injury type, where there has been complete transection of the nerve trunk, loss of significant trigeminal cells with centralized effects, and amputation neuromas forming at the injury site. Patients who have sustained full-transection injuries of intrabony IAN, yet have retained good nerve-end apposition and support within the mandibular canal, may undergo satisfactory levels of spontaneous recovery. Many Type 5 injured patients, however, will experience intractable pain and poor orofacial functions and may benefit from repair. In almost all Type 5 injuries to lingual and infraorbital nerves, satisfactory spontaneous recovery will not occur and should be microsurgically repaired as soon as the nature of the injury is confirmed and sensory testing demonstrates anesthesia, with or without dysesthesia ( Table 15-1 ).
DETERMINANTS OF NERVE INJURY RESPONSES
A number of factors, both local and host-related, will determine the neurologic responses to injury. The type of injury is a primary determinant of neurologic responses. Cleanincision lacerations are less problematic than irregular or avulsive lacerations. Injuries are clinically more disruptive when they involve mixed nerve receptor types, such as combinations of somatosensory mechanoreceptors and taste receptors, and somatosensory-autonomic fiber combinations, such as maxillary nerve branches that contain postganglionic parasympathetic fibers. Injuries that occur at proximal positions on the peripheral nerve are more significant than those that occur at more distal sites because they are more likely to result in loss of trigeminal ganglion cells and initiate retrograde deafferentation effects into the central nervous system. Multiple nerve injuries are also more significant than isolated injuries because of accumulative central deafferentation effects.
Complete nerve lacerations or avulsions produce markedly different responses than partial or incomplete nerve injuries with regard to the effects on sensory perception and type of dysesthesia that result. Paradoxically, lesser neurotrauma, as seen with Sunderland Type 2 and 3 injuries and partial or puncture injuries, are more likely to be associated with early hyperesthesias, such as triggered pain, hyperalgesia, allodynia, and immediate postinjury dysesthesias. Severe Type 4 internal nerve damage and Type 5 complete laceration or avulsion injuries, by contrast, are often not immediately painful, but eventually lead to the formation of dysfunctional chronic neuroma-in-continuity and amputation neuromas. These initially anesthetic lesions are more often associated with poor orofacial function, referred and radiating forms of unpleasant paresthesias, and spontaneous pain.
Host factors are also significant determinants of the responses to nerve injury. Increasing age negatively affects recovery from all nerve injuries. Genetic factors, although inadequately understood at this time, appear to be important determinants through effects, such as increased intraneural collagenation, extraneural scar, and autonomic derangements. The presence of nerve inflammation, bacterial infections, or herpes simplex or zoster viral outbreaks in the nerve injury distributions can also interfere with the injury response and recovery process. Even low-grade repetitive irritations of the injured peripheral nerve or neuroma may induce a retrograde process of central sensitization known as “windup.”
Systemic influences also may influence nerve injury responses; they include neurovascular diseases, such as chronic arthritis, systemic lupus, and diabetic neuropathy. Exposure to environmental neurotoxins, therapeutic medicaments, nicotinic acid, and nitrosamine components of tobacco may all influence nerve injury responses by impairing Schwann cell regenerative functions.
▪
CLINICAL MEASUREMENT AND DIAGNOSIS
Clinical evaluation of patients with posttraumatic trigeminal neuropathy requires quantitative assessments of: (1) impaired global and orofacial functions, (2) pain and discomfort, and (3) abnormal sensory stimulus responses. These assessments are facilitated by the use of patient questionnaires, severity scaling, QST, and pharmacologic analyses.
ASSESSING IMPAIRED FUNCTIONS
Trigeminal nerve injuries disrupt both orofacial and general life functions. It is useful to ask that patients quantify their perceived levels of dysfunction as a basis for treatment planning and for determining the effectiveness of treatments. A suggested format for patient dysfunction scoring is presented in Table 15-2 . These same measures can be used at follow-up patient visits to monitor progress in functions during recovery from injury or surgery.
Mark on the following scale your estimate of the amount of impairment or disability that you are now experiencing as a result of your nerve injury problem: | ||||
0 | 25 | 50 | 75 | 100% |
none | mild | moderate | severe | complete |
Please circle the functions that have been impaired by your current nerve injury problem: eating talking swallowing tasting tooth brushing dental care washing face smelling smiling lovemaking sleeping working socializing other ______ |
ASSESSING PATIENT DISCOMFORT AND PAIN
A questionnaire modified from the extensively tested McGill Pain Inventory is useful for eliciting patient-perceived levels of neurosensory discomfort and pain ( Table 15-3 ). It includes a linear scale on which patients are asked to focus on their region of nerve injury and rate their “discomfort” on a global scale from zero (no discomfort) through 100% (“intolerable pain”).
Mark on the following scale your estimate of the average daily level of discomfort or pain: | ||||
0 | 25 | 50 | 75 | 100% |
no discomfort discomfort | mild pain | moderate pain | severe pain | intolerable pain |
Please circle any words that describe your current sensation or pain problems: constant intermittent rhythmic steady brief triggered spontaneous numb itching dry tickling tingling twitching wet rubbery stretched swollen woody crawling moving quivering vibrating cool warm cold hot burning pricking stinging electric cold hot burning tender sore painful sore aching excruciating cramping shocking bitter sweet sour salty tasteless |
A listing of verbal descriptors is presented to patients to elicit qualitative information about their neuropathy, and it can be helpful in revealing underlying mechanisms of sensory abnormality, pain, and dysfunction. Particular patterns of a verbal descriptor may help the clinician discriminate between different categories of pain. For example, certain forms of neuropathic pain have been found to be strongly linked to “tingling, hyperesthesia to touch, electric, and burning” descriptors.
A note of caution is given to clinicians interpreting verbal descriptor complaints after nerve injury. All English-speaking patients, when asked about altered sensation in their affected nerve distributions, use the term “numbness.” However, QST reveals that there is a wide range of objective sensory loss among patients who use the term “numbness”; some patients test severely hypoesthetic and even anesthetic, whereas other “numb patients” may display near-normal stimulus responses with objective testing. It is also important to recognize this communication factor when final assessments of the success of spontaneous recovery or surgical treatments are made. Even in cases where there have been significant improvements in objective functions and QST, some patients may interpret their recovery responses as “unsuccessful” because they still retain “numbness.”
CLINICAL NEUROSENSORY TESTING
Clinical neurosensory testing is done to determine the degree of sensory impairments, detect neuropathic states, monitor recovery, and determine whether trigeminal nerve repair is indicated ( Figure 15-1 ). Although a wide range of protocols for experimental and clinical QST are currently being evaluated, no single system is universally accepted. In standard protocols, a range of stimuli from light mechanical to noxious are presented to the patient’s region of nerve injury, and their responses are compared quantitatively with responses from contralateral uninjured tissues. The tests are designed to detect and measure both negative responses (sensory deficits) and positive responses (hyperesthetic reactions); this test scheme also recognizes the possibility of normalcy in stimulus responses (see Figure 15-1 ).
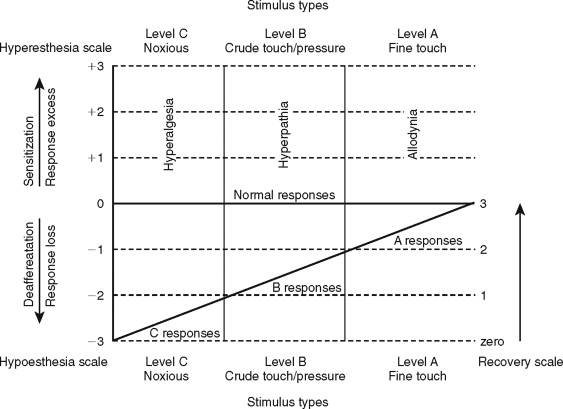
QST begins with the stimulation of large myelinated A-alpha and A-beta nerve fibers associated with fine touch discrimination, known as “Level A” testing. Level A stimulus parameters include choices, such as two-point touch discrimination, static touch stimuli with light Frey’s hairs, or detection of brush or cotton-wisp stroking. The patient’s level of correct detections in nerve-injured zones are compared with normal control tissues, and the quantified results are recorded or mapped on an orofacial drawing ( Figure 15-2 ). Level A testing may result in normal control levels of stimulus detection, or it may reveal a full range of neural deficits for fine-discriminative touch abilities (negative responses, see Figure 15-1 ). The fine touch stimuli are also used simultaneously to expose abnormal sensory “overresponses” from patients who report that the stimuli elicit paresthesias or, in some cases, pain. Uncomfortable paresthesias and pain would be recorded as a “positive” hyperesthesia (see Figure 15-1 ), in this case allodynia , defined as “pain (or heightened paresthesia) due to a stimulus which does not normally provoke pain.” If allodynia is exposed by Level A testing, patients are asked to rank their abnormal responses as mild, moderate, or severe (+1 to +3, see Figure 15-1 ). Because posttraumatic neuropathic sensitization can be due to both peripheral and central mechanisms, the finding of allodynia during QST does not serve as an indication or contraindication to peripheral microsurgery.
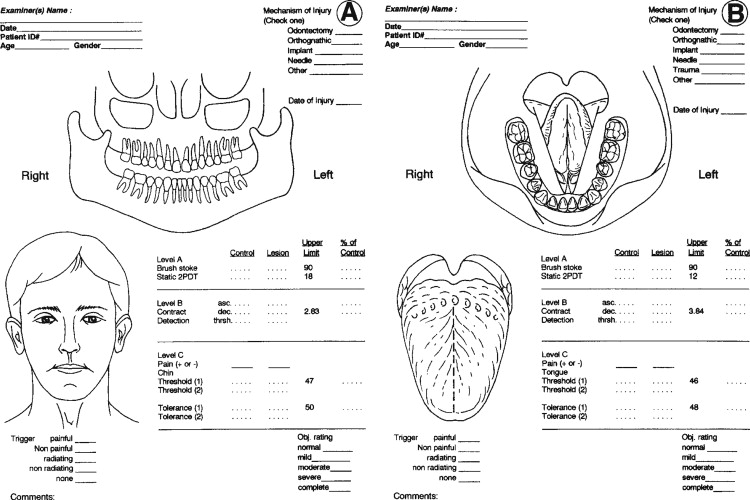
Testing next turns to Level B evaluation of the patient’s crude-touch detection abilities. More intense, blunt, and crude forms of mechanical stimuli can be used, such as thicker Frey’s filaments, nonnoxious light pin touching, and pressure stimuli from an algometer. The stimulus intensities that patients require to detect the stimuli are recorded and mapped as with Level A. Patients who display deficits for (Level A) fine discriminative stimuli, yet have good detection levels for crude-touch Level B stimuli, are not usually candidates for microsurgery because this is an acceptable functional level of neurosensory recovery.
Hyperesthesias are also evaluated at Level B testing by noting paresthesia and pain responses to the crude-touch, compression, and percussion stimuli applied over the nerve distributions. This may elicit the hyperphenomenon, known as a pathologic Tinel’s sign or hyperpathia , defined as “a painful syndrome characterized by an abnormal painful reaction to a stimulus, especially a repetitive (mechanical) stimulus, as well as increased threshold.” This “trigger response” is an indication of probable irritability of larger nociceptor A-delta fibers, the likely presence of traumatic neuroma-in-continuity, and is an anatomic target for nerve repair.
Level C examination presents noxious stimuli to the injured nerve distribution, testing for the level of C fiber protective abilities. Most common and convenient clinical stimuli are deeper pin pressures, calibrated hemostat pinches, or instruments heated to 48 °C to 50 °C. Patients who respond to noxious stimulation by reporting pain well above that experienced from stimulation of their control tissues are hyperalgesic. Hyperalgesia is defined as “an increased response to a stimulus which is normally painful.” It represents another form of neural sensitization produced by both peripheral and central nervous system mechanisms.
Patients who fail testing at levels A and B but have retained or regained basic detection of noxious stimuli are usually able to protect their tissues from secondary traumas. However, they more often have difficulties with orofacial functions, such as mastication and speech, and are candidates for microsurgery to improve on these functions. Patients who respond anesthetic to Level C noxious stimuli are obvious candidates for surgical repair.
QUANTIFYING NEUROSENSORY RECOVERY
A final purpose of clinical QST is to rate the existing stage of neurosensory recovery, using a suggested zero- to four-point recovery scale (see Figure 15-1 ). Zero represents no measurable neurosensory recovery (anesthetic), #1 the recovery range of Level C noxious detection functions, #2 the recovery range of Level B crude-touch functions, #3 the recovery range of Level A fine-tactile functions, and #4 full normal (control level) functions.
PHARMACOLOGIC TESTING
Pharmacologic testing, using lidocaine in various forms, can expand on information gained from clinical sensory testing regarding neuropathy mechanisms. It can also help predict the efficacy of treating patients with medical alternatives versus nerve-repair surgery.
Local anesthetic blocks with lidocaine are made proximal to the sites of nerve injury for patients with significant discomfort or neuropathic pain. Positive and sustained pain relief is often a reliable prediction that surgical repair will also reduce pain levels. Conversely, failure in pain relief from a successful block is a sign that irreversible centralized pain sensitization exists and that surgical repair is unlikely to improve pain levels.
Topical lidocaine and transcutaneous 12-hour release patches are less predictive of efficacy of surgery, but are useful for palliative pain management acutely after injury and during recovery from nerve repair. For some patients, long-term regular use of transcutaneous lidocaine is an acceptable alternative to surgery.
Intravenous lidocaine testing has been used successfully to predict the efficacy of centrally acting medical therapies as alternatives to surgery for patients with neuropathic pain. Lidocaine infusions at 1 mg/kg over a 5-minute time frame may attenuate centrally sensitized neuropathic states without inducing cognitive deficits. This may be helpful in directing long-term medical therapies with centrally acting agents that may be preferred by some patients over surgical repairs of painful nerve injuries.
ASSESSING PATIENTS WITH TRAUMATIC NEUROPATHIC PAIN
Previous studies of patients with trigeminal traumatic neuralgias have combined information from verbal descriptors, scaling scores, quantitative sensory and pharmacologic testing, and nerve lesions observed at the time of surgical nerve repairs. These studies have shown that patients whose complaints center on dysesthesia or altered sensation with pain after nerve injury cluster in two differing neuropathic clinical profiles: hypoesthetic pain and hyperesthetic pain. Patients with a primarily hypoesthetic pattern complain immediately after injury of “numbness” and often have minimal neuropathic pain. When examined with QST, these patients are deficient in neurosensory responses for all types and levels of QST. Days to weeks after injury, however, patients gradually report an intensifying pain perceived in the injured nerve region and complain of “constant, dull, deep, crawling, and burning” discomfort.
When presented with Level B crude-touch stimuli, patients with a hypoesthetic pattern experience intensified paresthesias and burning hyperpathias. Studies have shown that many of these patients have formed traumatic neuroma-in-continuity from Sunderland Type 3 injury or amputation neuroma from Type 4 injury. If identified within a few weeks after nerve injury, these patients may respond favorably to early surgical exploration and repair.
Unfortunately, studies have also demonstrated that with longer postinjury time, many patients with hypoesthetic traumatic neuralgias experience further increases in constant, spontaneous pain that does not respond to local anesthetic blocks applied to the injured nerve trunks, suggesting both central and peripheral sites of pain action. This pattern is central deafferentation syndrome, or “anesthesia dolorosa,” and is associated with severe nerve lesions, permanent loss of trigeminal ganglion cells, and reduced afferent input to the brainstem. It has been postulated that major deafferentation leads to “irritable foci” that develop electrophysiologically in the spinal cord and extend to thalamic and even cortical levels. Deafferentation pain syndromes, if sustained for weeks or months after nerve injury, become refractory to both medical therapies and delayed microsurgical repairs.
Patients with a primarily hyperesthetic pain pattern, in contrast to those with hypoesthetic neuralgias, have more severe early pain within hours to days of nerve injury. Their primary verbal descriptors are “shocking, tingling, burning, electric, and episodic.” Studies have shown that early, intense responses more likely follow partial nerve injuries, such as traction, compression, puncture, local anesthetic, chemical, and burn injuries.
A prime clinical feature of hyperesthetic posttraumatic neuralgias is the presence of triggered paresthesias, allodynia, and hyperalgesia, phenomena, which may be expressions of both peripheral and central mechanisms. Local anesthetic nerve blocks produce a transient but complete remission from allodynia and hyperalgesia. Patients with hyperesthetic pain patterns are candidates for surgical decompression and repair if their triggered pain and dysfunction are intolerable or refractory to medical treatments.
It is also important that hyperesthetic neuralgia patients be closely monitored through serial neurosensory examinations after injury to detect signs of emerging central sensitization, which is seen as a spread of the neuropathic nerve distributions (surround hyperalgesia) and new symptoms contralateral to the nerve injury.
Although the extreme forms of hyperesthetic and hypoesthetic neuralgia patterns are usually easy to differentiate through patient study, it is also the case that patients may have combined or mixed features. The decision to proceed with surgical repairs becomes more challenging in such cases.
▪
CLINICAL MEASUREMENT AND DIAGNOSIS
Clinical evaluation of patients with posttraumatic trigeminal neuropathy requires quantitative assessments of: (1) impaired global and orofacial functions, (2) pain and discomfort, and (3) abnormal sensory stimulus responses. These assessments are facilitated by the use of patient questionnaires, severity scaling, QST, and pharmacologic analyses.
ASSESSING IMPAIRED FUNCTIONS
Trigeminal nerve injuries disrupt both orofacial and general life functions. It is useful to ask that patients quantify their perceived levels of dysfunction as a basis for treatment planning and for determining the effectiveness of treatments. A suggested format for patient dysfunction scoring is presented in Table 15-2 . These same measures can be used at follow-up patient visits to monitor progress in functions during recovery from injury or surgery.
Mark on the following scale your estimate of the amount of impairment or disability that you are now experiencing as a result of your nerve injury problem: | ||||
0 | 25 | 50 | 75 | 100% |
none | mild | moderate | severe | complete |
Please circle the functions that have been impaired by your current nerve injury problem: eating talking swallowing tasting tooth brushing dental care washing face smelling smiling lovemaking sleeping working socializing other ______ |
ASSESSING PATIENT DISCOMFORT AND PAIN
A questionnaire modified from the extensively tested McGill Pain Inventory is useful for eliciting patient-perceived levels of neurosensory discomfort and pain ( Table 15-3 ). It includes a linear scale on which patients are asked to focus on their region of nerve injury and rate their “discomfort” on a global scale from zero (no discomfort) through 100% (“intolerable pain”).
Mark on the following scale your estimate of the average daily level of discomfort or pain: | ||||
0 | 25 | 50 | 75 | 100% |
no discomfort discomfort | mild pain | moderate pain | severe pain | intolerable pain |
Please circle any words that describe your current sensation or pain problems: constant intermittent rhythmic steady brief triggered spontaneous numb itching dry tickling tingling twitching wet rubbery stretched swollen woody crawling moving quivering vibrating cool warm cold hot burning pricking stinging electric cold hot burning tender sore painful sore aching excruciating cramping shocking bitter sweet sour salty tasteless |
A listing of verbal descriptors is presented to patients to elicit qualitative information about their neuropathy, and it can be helpful in revealing underlying mechanisms of sensory abnormality, pain, and dysfunction. Particular patterns of a verbal descriptor may help the clinician discriminate between different categories of pain. For example, certain forms of neuropathic pain have been found to be strongly linked to “tingling, hyperesthesia to touch, electric, and burning” descriptors.
A note of caution is given to clinicians interpreting verbal descriptor complaints after nerve injury. All English-speaking patients, when asked about altered sensation in their affected nerve distributions, use the term “numbness.” However, QST reveals that there is a wide range of objective sensory loss among patients who use the term “numbness”; some patients test severely hypoesthetic and even anesthetic, whereas other “numb patients” may display near-normal stimulus responses with objective testing. It is also important to recognize this communication factor when final assessments of the success of spontaneous recovery or surgical treatments are made. Even in cases where there have been significant improvements in objective functions and QST, some patients may interpret their recovery responses as “unsuccessful” because they still retain “numbness.”
CLINICAL NEUROSENSORY TESTING
Clinical neurosensory testing is done to determine the degree of sensory impairments, detect neuropathic states, monitor recovery, and determine whether trigeminal nerve repair is indicated ( Figure 15-1 ). Although a wide range of protocols for experimental and clinical QST are currently being evaluated, no single system is universally accepted. In standard protocols, a range of stimuli from light mechanical to noxious are presented to the patient’s region of nerve injury, and their responses are compared quantitatively with responses from contralateral uninjured tissues. The tests are designed to detect and measure both negative responses (sensory deficits) and positive responses (hyperesthetic reactions); this test scheme also recognizes the possibility of normalcy in stimulus responses (see Figure 15-1 ).
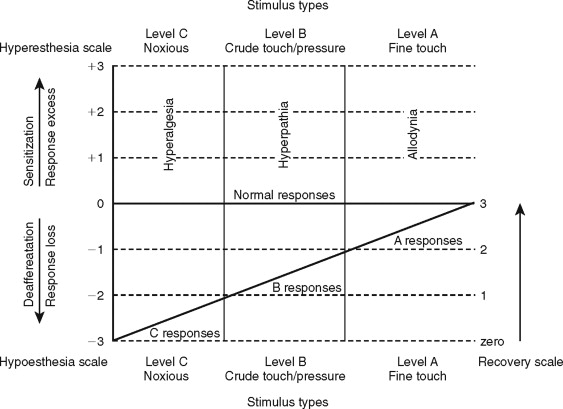
QST begins with the stimulation of large myelinated A-alpha and A-beta nerve fibers associated with fine touch discrimination, known as “Level A” testing. Level A stimulus parameters include choices, such as two-point touch discrimination, static touch stimuli with light Frey’s hairs, or detection of brush or cotton-wisp stroking. The patient’s level of correct detections in nerve-injured zones are compared with normal control tissues, and the quantified results are recorded or mapped on an orofacial drawing ( Figure 15-2 ). Level A testing may result in normal control levels of stimulus detection, or it may reveal a full range of neural deficits for fine-discriminative touch abilities (negative responses, see Figure 15-1 ). The fine touch stimuli are also used simultaneously to expose abnormal sensory “overresponses” from patients who report that the stimuli elicit paresthesias or, in some cases, pain. Uncomfortable paresthesias and pain would be recorded as a “positive” hyperesthesia (see Figure 15-1 ), in this case allodynia , defined as “pain (or heightened paresthesia) due to a stimulus which does not normally provoke pain.” If allodynia is exposed by Level A testing, patients are asked to rank their abnormal responses as mild, moderate, or severe (+1 to +3, see Figure 15-1 ). Because posttraumatic neuropathic sensitization can be due to both peripheral and central mechanisms, the finding of allodynia during QST does not serve as an indication or contraindication to peripheral microsurgery.
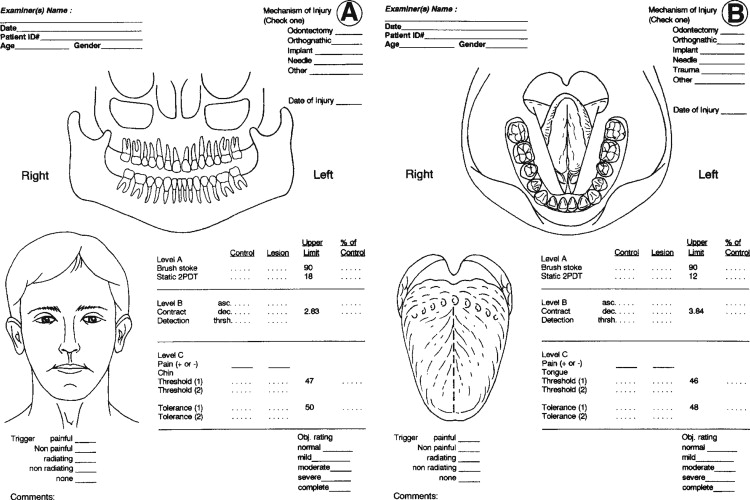
Testing next turns to Level B evaluation of the patient’s crude-touch detection abilities. More intense, blunt, and crude forms of mechanical stimuli can be used, such as thicker Frey’s filaments, nonnoxious light pin touching, and pressure stimuli from an algometer. The stimulus intensities that patients require to detect the stimuli are recorded and mapped as with Level A. Patients who display deficits for (Level A) fine discriminative stimuli, yet have good detection levels for crude-touch Level B stimuli, are not usually candidates for microsurgery because this is an acceptable functional level of neurosensory recovery.
Hyperesthesias are also evaluated at Level B testing by noting paresthesia and pain responses to the crude-touch, compression, and percussion stimuli applied over the nerve distributions. This may elicit the hyperphenomenon, known as a pathologic Tinel’s sign or hyperpathia , defined as “a painful syndrome characterized by an abnormal painful reaction to a stimulus, especially a repetitive (mechanical) stimulus, as well as increased threshold.” This “trigger response” is an indication of probable irritability of larger nociceptor A-delta fibers, the likely presence of traumatic neuroma-in-continuity, and is an anatomic target for nerve repair.
Level C examination presents noxious stimuli to the injured nerve distribution, testing for the level of C fiber protective abilities. Most common and convenient clinical stimuli are deeper pin pressures, calibrated hemostat pinches, or instruments heated to 48 °C to 50 °C. Patients who respond to noxious stimulation by reporting pain well above that experienced from stimulation of their control tissues are hyperalgesic. Hyperalgesia is defined as “an increased response to a stimulus which is normally painful.” It represents another form of neural sensitization produced by both peripheral and central nervous system mechanisms.
Patients who fail testing at levels A and B but have retained or regained basic detection of noxious stimuli are usually able to protect their tissues from secondary traumas. However, they more often have difficulties with orofacial functions, such as mastication and speech, and are candidates for microsurgery to improve on these functions. Patients who respond anesthetic to Level C noxious stimuli are obvious candidates for surgical repair.
QUANTIFYING NEUROSENSORY RECOVERY
A final purpose of clinical QST is to rate the existing stage of neurosensory recovery, using a suggested zero- to four-point recovery scale (see Figure 15-1 ). Zero represents no measurable neurosensory recovery (anesthetic), #1 the recovery range of Level C noxious detection functions, #2 the recovery range of Level B crude-touch functions, #3 the recovery range of Level A fine-tactile functions, and #4 full normal (control level) functions.
PHARMACOLOGIC TESTING
Pharmacologic testing, using lidocaine in various forms, can expand on information gained from clinical sensory testing regarding neuropathy mechanisms. It can also help predict the efficacy of treating patients with medical alternatives versus nerve-repair surgery.
Local anesthetic blocks with lidocaine are made proximal to the sites of nerve injury for patients with significant discomfort or neuropathic pain. Positive and sustained pain relief is often a reliable prediction that surgical repair will also reduce pain levels. Conversely, failure in pain relief from a successful block is a sign that irreversible centralized pain sensitization exists and that surgical repair is unlikely to improve pain levels.
Topical lidocaine and transcutaneous 12-hour release patches are less predictive of efficacy of surgery, but are useful for palliative pain management acutely after injury and during recovery from nerve repair. For some patients, long-term regular use of transcutaneous lidocaine is an acceptable alternative to surgery.
Intravenous lidocaine testing has been used successfully to predict the efficacy of centrally acting medical therapies as alternatives to surgery for patients with neuropathic pain. Lidocaine infusions at 1 mg/kg over a 5-minute time frame may attenuate centrally sensitized neuropathic states without inducing cognitive deficits. This may be helpful in directing long-term medical therapies with centrally acting agents that may be preferred by some patients over surgical repairs of painful nerve injuries.
ASSESSING PATIENTS WITH TRAUMATIC NEUROPATHIC PAIN
Previous studies of patients with trigeminal traumatic neuralgias have combined information from verbal descriptors, scaling scores, quantitative sensory and pharmacologic testing, and nerve lesions observed at the time of surgical nerve repairs. These studies have shown that patients whose complaints center on dysesthesia or altered sensation with pain after nerve injury cluster in two differing neuropathic clinical profiles: hypoesthetic pain and hyperesthetic pain. Patients with a primarily hypoesthetic pattern complain immediately after injury of “numbness” and often have minimal neuropathic pain. When examined with QST, these patients are deficient in neurosensory responses for all types and levels of QST. Days to weeks after injury, however, patients gradually report an intensifying pain perceived in the injured nerve region and complain of “constant, dull, deep, crawling, and burning” discomfort.
When presented with Level B crude-touch stimuli, patients with a hypoesthetic pattern experience intensified paresthesias and burning hyperpathias. Studies have shown that many of these patients have formed traumatic neuroma-in-continuity from Sunderland Type 3 injury or amputation neuroma from Type 4 injury. If identified within a few weeks after nerve injury, these patients may respond favorably to early surgical exploration and repair.
Unfortunately, studies have also demonstrated that with longer postinjury time, many patients with hypoesthetic traumatic neuralgias experience further increases in constant, spontaneous pain that does not respond to local anesthetic blocks applied to the injured nerve trunks, suggesting both central and peripheral sites of pain action. This pattern is central deafferentation syndrome, or “anesthesia dolorosa,” and is associated with severe nerve lesions, permanent loss of trigeminal ganglion cells, and reduced afferent input to the brainstem. It has been postulated that major deafferentation leads to “irritable foci” that develop electrophysiologically in the spinal cord and extend to thalamic and even cortical levels. Deafferentation pain syndromes, if sustained for weeks or months after nerve injury, become refractory to both medical therapies and delayed microsurgical repairs.
Patients with a primarily hyperesthetic pain pattern, in contrast to those with hypoesthetic neuralgias, have more severe early pain within hours to days of nerve injury. Their primary verbal descriptors are “shocking, tingling, burning, electric, and episodic.” Studies have shown that early, intense responses more likely follow partial nerve injuries, such as traction, compression, puncture, local anesthetic, chemical, and burn injuries.
A prime clinical feature of hyperesthetic posttraumatic neuralgias is the presence of triggered paresthesias, allodynia, and hyperalgesia, phenomena, which may be expressions of both peripheral and central mechanisms. Local anesthetic nerve blocks produce a transient but complete remission from allodynia and hyperalgesia. Patients with hyperesthetic pain patterns are candidates for surgical decompression and repair if their triggered pain and dysfunction are intolerable or refractory to medical treatments.
It is also important that hyperesthetic neuralgia patients be closely monitored through serial neurosensory examinations after injury to detect signs of emerging central sensitization, which is seen as a spread of the neuropathic nerve distributions (surround hyperalgesia) and new symptoms contralateral to the nerve injury.
Although the extreme forms of hyperesthetic and hypoesthetic neuralgia patterns are usually easy to differentiate through patient study, it is also the case that patients may have combined or mixed features. The decision to proceed with surgical repairs becomes more challenging in such cases.
▪
NERVE REPAIR SURGERIES ( Figure 15-3 )
PURPOSES AND PATIENT SELECTION
Neurosurgical interventions for patients who have sustained trigeminal injuries have two purposes: to restore orofacial sensory functions and to manage pain and discomfort. Repairs are only done on sensory trigeminal branches; the motor root is inaccessible except for major cranial-base procedures. The sensory trigeminal nerves most commonly affected and amenable to repair are the inferior alveolar, lingual, infraorbital nerves, less frequently the supraorbital and supratrochlear branches. The long buccal nerve is probably damaged with great frequency during routine oral surgeries, but they are not associated with significant levels of neuralgias or dysfunction and therefore are not routinely treated. The auriculotemporal nerves are also susceptible to injury during facial trauma and surgeries for temporomandibular joint reconstruction and parotidectomy. Although pain and dysfunction are described for auriculotemporal injuries, there are no current microneurosurgical treatments for these conditions.
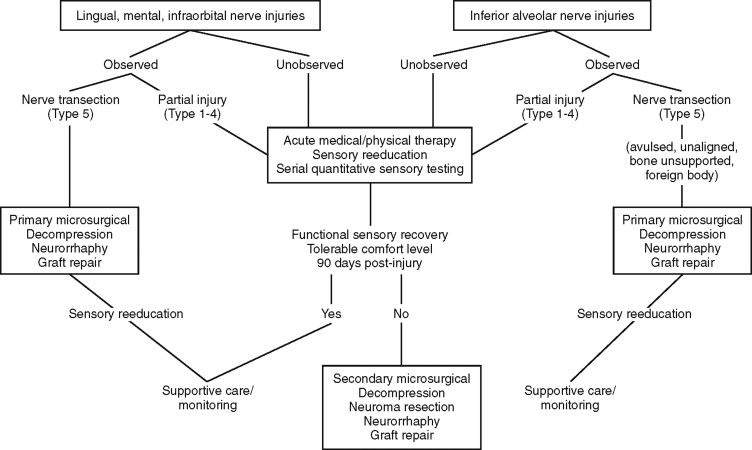
Indications for surgical exploration and repairs of trigeminal nerves are: (1) observed nerve injuries, (2) presence of paraneural foreign bodies, (3) unchanging anesthesia or dysfunctional hypoesthesia more than 2 months after injury, and (4) medically intractable neuropathic pain. Additional clinical indicators that are predictive of surgical success include presence of specific dysesthetic (hyperpathic) trigger points indicating underlying traumatic neuroma and relief of pain and paresthesia with local anesthetic nerve blocks.
Contraindications for trigeminal surgical exploration and repair are: (1) signs of improving neurosensory functions based on serial QST; (2) patient acceptance of existing impaired function and discomfort levels; (3) signs of central sensitization (spreading regional dysesthesia, secondary hyperalgesia); (4) deafferentation pain unrelieved by local anesthetic nerve blocks; (5) clinical signs of autonomic derangement (burning sensations, erythema, swelling); (6) extremes of age, and systemic or neuropathic disease; (7) excessive time since original nerve injury; and (8) unrealistic patient expectations (restoration of immediate, normal, or pain-free neurosensory functions).
ACUTE NERVE INJURY PATIENT CARE
The primary goals of early nerve injury treatments are to remove sources of ongoing or secondary nerve injury, to create a tissue environment for optimal nerve recovery, and to prevent secondary neuropathic sensitization.
A soft diet is advised, hypermobile fractures should be stabilized, and cryotherapy applied to the region. Injured nerves, if visualized, should be freed of compressing foreign bodies, bone or tooth fragments, toxic materials, or implant. Exposed nerves should be cleansed with isotonic solution, gently coapted and stabilized with temporary epineural sutures, if possible, and barrier protected. Infection and inflammation control, both locally and systemically, should be maximized. Antiinflammatory, opiate analgesic, and psychosedative agents for anxiety control and sleep promotion are used aggressively to minimize CNS excitation. This may include use of local anesthetic nerve blocks with long-acting agents. A course of systemic corticosteroids, such as dexamethasone 8 to 12 mg/day may minimize perineural inflammation during the first postinjury week. Rapid-acting anticonvulsant agents, such as clonazepam, given in divided doses at 2 to 10 mg daily may further protect the CNS. Topical lidocaine can be delivered either by p.r.n. lidocaine gels or through sustained 12-hour release 5% transcutaneous lidocaine patch applications.
A baseline examination with QST should be done and the affected nerve distribution mapped. Patients should be counseled regarding the nature of the nerve injury, the importance of immediate and sustained observation and care, the possible need for secondary consultation and microrepair, and the probability of a protracted course of nerve recovery.
TIMING OF SURGERY
Surgeries are categorized according to the time of repair relative to the injury dates. They are primary (immediate), delayed primary, and secondary. Primary repairs are done in those instances when the nerve has been exposed and the injury observed, as is common during surgeries for trauma, dentoalveolar pathologic conditions, orthognathic, implant-reconstruction, and some third molar surgeries. Immediate (primary) nerve surgeries, if technically possible, are the preferred time of repair from a biologic perspective. Axonal regeneration is known to be optimal in earlier time frames, within the first 3 weeks after injury, and before neuroma formation and restrictive secondary scarring can occur. Limited but effective primary repairs may be possible, even in office settings. Surgical loupes can enable nerve decompression, passive nerve apposition with or without epineural suturing, and protection of the exposed nerve with inert alloplastic barriers, such as Gelfoam or collagen tubule. Primary surgery may then be followed by “delayed primary” repair if needed and done within a few weeks of injury when regional tissues have recovered from acute surgical effects and plans can be made for more definitive nerve exposure and microsurgical repair.
Unobserved trigeminal nerve injuries are the more common circumstance and require secondary surgical repairs under controlled conditions and after patients have met the surgical indications’ criteria dictated by clinical conditions and serial QST. The question of optimal timing for secondary surgeries remains controversial and is the subject of ongoing research. There is much evidence that earlier surgical repairs, within 1 to a few weeks following injury, are preferred over longer delays for three basic reasons: (1) optimal regenerative capacity occurs within the first few weeks after injury, (2) early intervention may prevent the development of traumatic neuromas and chronic neuropathic sensitization, and (3) ease of technical repairs. With excessive delays, microsurgical repairs become more difficult because of retraction and progressive atrophy of nerve segments, increased extraneural and intraneural collagen deposition, and Schwann cell scarring. Outcome analyses have shown that lingual nerve repairs done within 90 days of injury can result in functional sensory recovery (FSR) levels as high as 93% at the 1-year mark. This same report showed, however, that FSR levels as high as 63% can be gained for selected cases in which surgery occurred after 90 days, and other studies supported the conclusion that there is no association between delayed repairs and sensory outcomes.
The timeline for soft tissue nerve injuries, such as lingual, infraorbital, and mental nerves, is much shorter than for intrabony nerve injuries, such as inferior alveolar. This is due to the very limited capacity for spontaneous regeneration of soft tissue nerves as compared with intrabony branches, where the mandibular canal can act as a natural conduit for nerve recovery, given enough time.
SURGICAL INSTRUMENTATION
Trigeminal nerve repairs require a quiet and controlled operating room environment. Surgical assistants familiar with the goals and procedures of the microrepair are essential. The microsurgical environment is more demanding of surgical assistants because of the limited surgical field access, the extra demands for stable retraction, hemorrhage control, and delicate tissue management. Endotracheal intubation with muscle relaxation is needed to prevent inadvertent patient movements. Hemostasis can be enhanced with reverse Trendelenburg’s positioning and local anesthesia with vasoconstrictor field blocking. Hypotensive general anesthesia may be required to assist in hemorrhage control along with bipolar electrocautery, used to prevent collateral injuries to the nerve under repair. Although nerve access, nerve release, and decompression can be accomplished with minimal magnification, such as 3× to 6× surgical loupes, more detailed microrepairs are best done with an operating microscope with multiple heads for assistant views of the operating field. Primary microsurgical instruments include a selection of small retractors, vein and nerve hooks, varied length microforceps, locking needle holders, and microscissors. Self-retaining flat vascular spreaders and vascular clamps can be used in some circumstances. Preferred sutures are nonreactive nylon or proline, 7-0 through 9-0. Nonreflective background materials, such as opaque foils, are helpful for visibility, tissue control, and to assist in atraumatic microsuctioning. Inert and nontoxic barriers, such as Gelfoam or biodegradable neurocuff materials, should be available for nerve protection after repair. Their purposes are to eliminate dead space, prevent paraneural hematoma, collagen ingrowth, and secondary scarring at the repair line and to minimize compression-ischemia.
The surgical principles for trigeminal microsurgery include gentle atraumatic tissue management, hemostasis, removal of paraneural foreign bodies and adhesive scar, excision of dysfunctional neuroma segments, and alignment and anastomosis of nerve segments with minimal tension. In those less optimal circumstances when direct neurorrhaphy is not possible, the surgical goal is to create a microenvironment that permits effective axonal down growth to nerve receptors, either through interpositional grafting or sealed interposed entubulation.
NERVE ACCESS AND MICROSURGICAL TECHNIQUES
Most microsurgical repairs can be accomplished intraorally with the exception of proximal injuries to infraorbital and periorbital nerves and IANs injured posteriorly and inferiorly. The primary steps in nerve repairs are: (1) decompression of entrapped injured nerves with removal of foreign bodies and release of paraneural compressing tissues or scars; (2) identification of injury site, diagnosis, and excision of traumatic neuroma ; (3) microsuture repair with neurorrhaphy (direct reanastomosis); and (4) interpositional graft repair if neurorrhaphy cannot be accomplished because of excessive nerve tissue loss.
DECOMPRESSION
Also known as external neurolysis, decompression is the first phase of all nerve repair procedures and involves a careful release of the injured nerve from paraneural scarring and removal of foreign bodies and impinging bone or tooth fragments. The magnifying optics at 3× to 10× are used to free the nerve while preserving its epineural vasculature. The nerve injury site is then further inspected, observing for nerve continuity, amputation neuroma, swellings, atrophy or opaque nerve regions, signifying internal fibrosis or neuroma-in-continuity. In those cases of Type 1 or 2 Sunderland nerve injuries, and when high magnification nerve inspection fails to reveal signs of neuroma, decompression may be the only surgery required. In that case, a protective neural cuff entubulation barrier may be placed before closure, using a protective sheath such as the recently developed polyglycolic acid (PGA)-collagen Neurocuffs. These alloplastic tubular conduits have been associated with pain remissions among patients with previously intractable neuropathic pain. There are no reports available, however, of their efficacy for treatment of trigeminal traumatic neuralgias.
NEUROMA RESECTION
After decompression, the mobilized nerve is controlled for repair by placing umbilical tapes proximal and distal to the nerve injury site. The nerve is inspected for discontinuity and amputation neuroma. In those cases where neuromain-continuity deformity is identified, the level of lesion is determined by high magnification inspection and transillumination of the nerve, noting nerve opacity and loss of surface microvasculature and gently compressing the nerve with microforceps to test for intraneural scarification.
When amputation or neuroma-in-continuity has been verified, the injury zone is sharply resected with a Beaver blade to approximately 3 mm proximal and distal to the visibly neuromatous tissue and submitted for histologic study. Satisfactory excision produces a normal herniation of intact fascicles from the epineurial nerve endings. Adequacy of neuroma resection can also be verified by submitting 1 mm cross-section biopsies for frozen section histopathologic verification of normal nerve fascicles. The tissue stumps are finally trimmed in preparation for microsurgical nerve repair.
In those rare cases in which inspection reveals that nerve repair is not possible, such as in the absence of the distal nerve trunk, the residual proximal nerve trunk is engaged and sutured into adjacent viable tissue, such as skeletal muscle. This procedure is known as nerve redirection and is believed to decrease the likelihood of reformation of active traumatic neuroma at the nerve stump.
INTERNAL NEUROLYSIS
A procedure used in the treatment of partial or neuromain-continuity injuries of large spinal nerves, known as internal neurolysis, has questionable utility for trigeminal nerve injury management. In this procedure, saline is injected beneath the epineurium in the nerve-injured zone. The fluid ballooned region is then incised longitudinally, exposing the internal nerve microstructure. When effective, this releases the internal nerve fibrosis and frees the nerve fascicles. Internal neurolysis has primary application for cases of mild internal fibrosis from Sunderland Type 2 and 3 injuries. If no release occurs, however, and internal nerve fibrosis remains after injection, the affected zone is excised as neuroma-in-continuity in preparation for neurorrhaphy. Internal neurolysis has a long history of successful use for treatment of spinal or digital nerves, where there are larger fascicles and thicker perineuria. Similar techniques are less feasible for the trigeminal nerves where distinctive fascicles and thicker perineurium does not exist. There are no available convincing reports of internal neurolysis being used effectively for trigeminal nerve repairs, and this author’s patient outcomes have been poor after internal neurolysis was used as the primary treatment.
NEURORRHAPHY
After neuroma resection, direct epineural microsurgical reanastomosis (neurorrhaphy) is done using 6 to 12× magnification, placing 7-0 to 9-0 nonreactive sutures usually at four to six circumferential locations. Completed neurorrhaphies should display no bulging or wrinkling between sutures, and the nerve should lie passively when released. Minimal repair line tension is significant because it has been shown that tension across nerve microrepairs greater than 25 g may produce gapping and inhibit axon down growth to the distal nerve. At the completion of neurorrhaphy, the repair site is further protected from hematoma, fibrous tissue ingrowth, and secondary neuroma formation by entubulating the nerve with an alloplastic protective barrier, such as a biodegradable PGA-collagen Neurocuff.
NERVE GRAFTING (see Figure 15-8 )
In more severe injury cases, direct neurorrhaphy repair may not be feasible. Clinical experience has shown that nerve gaps of greater than 15 to 20 mm cannot be routinely bridged by direct neurorrhaphy without requiring excessive nerve dissection or producing excessive tension at the repair site. A range of interpositional and entubulation graft procedures has been used for more extreme injuries, including autologous nerve grafts, interpositional grafting with muscle, interpositional entubulation with veins or arteries, and alloplastic tubules.
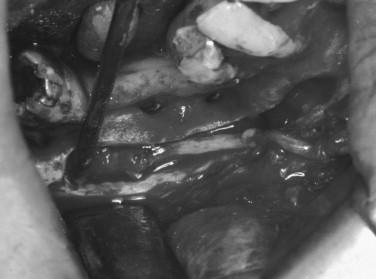
Autologous Grafts
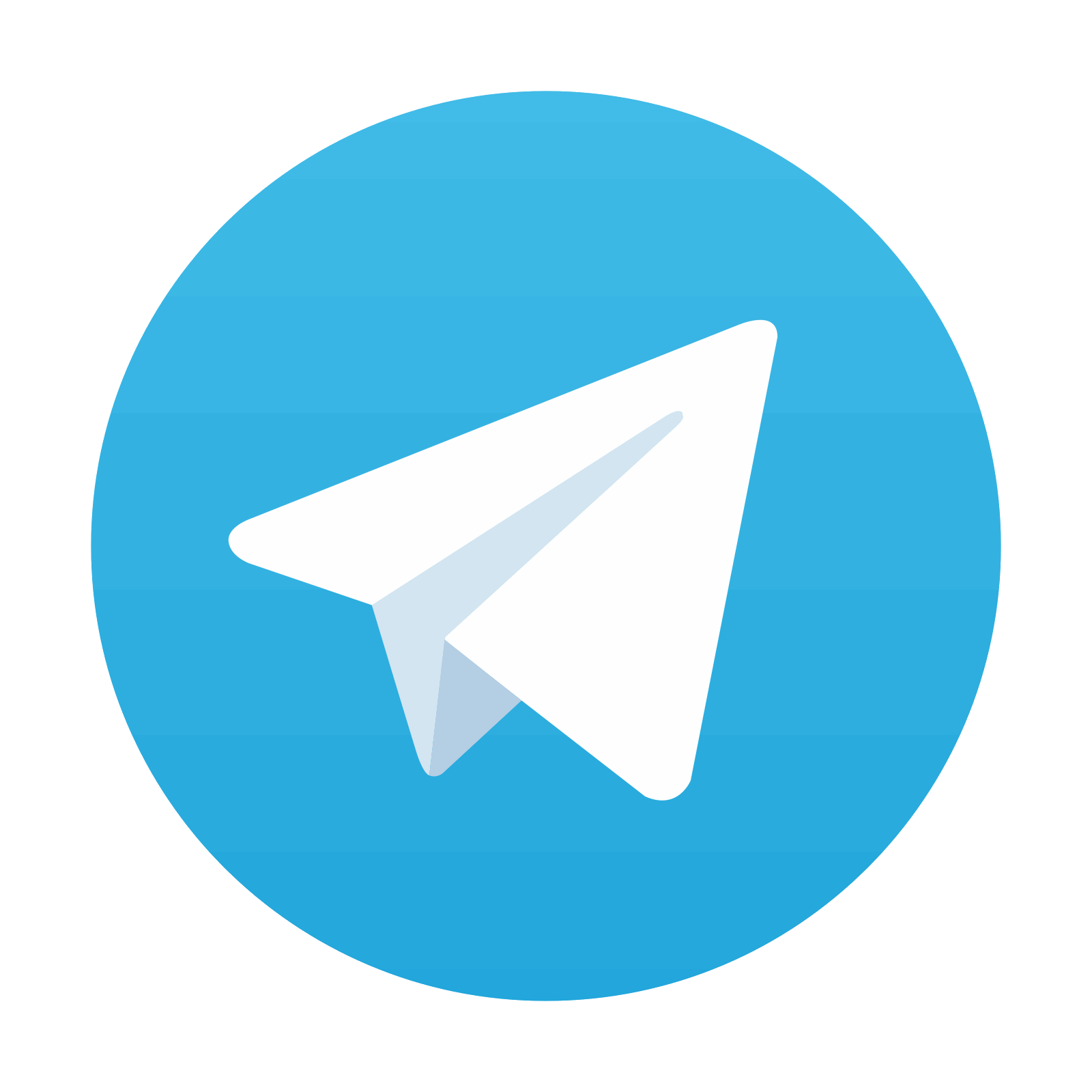
Stay updated, free dental videos. Join our Telegram channel

VIDEdental - Online dental courses
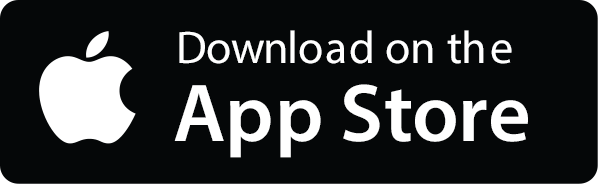
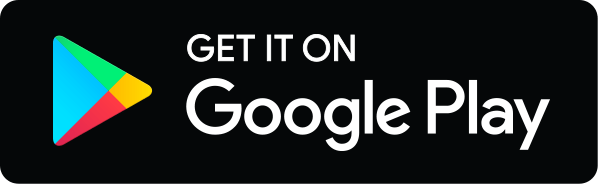