Tissue response to orthodontic forces
Brigit Thilander
Key topics
- Tooth-supporting tissues
- Physiologic tooth migration
- Orthodontic tooth movements
- Transmission of orthodontic forces into cellular reactions
- Biomechanical principles
Learning objectives
- To understand the sense of physiological tooth migration in orthodontics
- To understand and describe the hyaline phase in tooth movements
- To understand and describe the tissue reaction on the pressure and tension sides in orthodontic tooth movements
- To understand the transmission of orthodontic forces into cellular reactions
- To describe the tissue reaction in the different kinds of tooth movements
Introduction
Orthodontic treatment comprises a wealth of removable and fixed appliances. Despite differences in design, they all involve the use and control of forces acting on the teeth and adjacent structures. Changes from such forces are seen in the dentoalveolar system, resulting in tooth movements, opposite to the forces that have an influence on sutures and condyles by means of dentofacial orthopaedics (Chapter 10). An optimal orthodontic force intends to induce a maximal cellular response and to establish stability of the tissue, whereas an unfavourable one may initiate adverse tissue reactions (Chapter 11). The purpose of this chapter is to focus on tissue reactions in the periodontium during the active phase of orthodontic treatment, while those during the retention and post-retention periods will be described in Chapter 12.
Tooth-supporting tissues
Tooth movement is a complicated process, requiring changes in the gingiva, periodontal ligament (PDL), root cementum, and alveolar bone with their differences in cell population and remodelling capacity. Therefore, a brief description of the normal periodontium is given.
Gingiva
The predominant component of the gingiva is the connective tissue, which consists of collagen fibres, fibroblasts, nerves and matrix. Fibroblasts are engaged in the production of various types of fibres, but are also involved in the synthesis of the connective tissue matrix. Collagen fibres are bundles of collagen fibrils with distinct orientation and divided into circular, dentogingival, dentoperiosteal and trans-septal fibres, and provide the resilience for maintaining the architectural form of the dentogingival attachment.
Periodontal ligament
The periodontal ligament (PDL), about 0.25 mm wide, is the soft, richly vascular and cellular connective tissue that surrounds the roots of the teeth and joins the root cementum with the alveolar bone. The true periodontal fibres, principal fibres, develop along with the eruption of the tooth, and their orientation alters continuously during tooth eruption. When the tooth has reached contact in occlusion, they constitute the following well-oriented groups (Figure 9.1): alveolar crest fibres and horizontal, oblique, apical, and inter-radicular fibres. The individual bundles have a slightly wavy course, which allows the tooth to move within its socket (physiologic mobility). The presence of a PDL is essential for tooth movements in orthodontic treatment.

Figure 9.1 The PDL fibres: alveolar-crest fibres (ACF), apical fibres (AF), gingival fibres (GF), horizontal fibres (HF), oblique fibres (OF) and interradicular fibres (RF).
The fibrils are embedded in a ground substance with connective tissue polysaccharides (glycosaminoglycans), which vary with age. The tissue response to orthodontic forces, including cell mobilization and conversion of collagen fibres, is considerably slower in older individuals than in children and adolescents. During physiological conditions, collagen turnover in PDL is much higher than that in most other tissues (e.g. twice as high as that of the gingiva). The high turnover has been attributed to the fact that forces on the PDL are multidirectional, having vertical and horizontal components. The lower collagen turnover in the gingiva may result from the lowered functional stress as the trans-septal fibres function in a manner similar to tendons, providing firm anchorage of the tooth.
Root cementum
The cementum is a specialized tissue covering the root surface. It contains no blood vessels, has no innervation, does not undergo remodelling, and is characterized by continuing deposition throughout life. During root formation, a primary cementum is formed. After tooth eruption and in response to functional demands, a secondary cementum is formed that, in contrast to the primary cementum, contain cells. During the continuous formation of the primary cementum, portions of the principle fibres in the PDL adjacent to the root become imbedded and mineralized (Figure 9.2).

Figure 9.2 Area from a 22-year-old patient. Chain of cementoblasts (C) along a thick layer of cementum. Widened capillary in a cleft, where bone resorption may start during the initial stage of tooth movement (W). Darkly stained surface line containing connective tissue polysaccharides (D) and note the absence of osteoblasts along the bone surface. Embedded principal fibres in the cementum of the tooth (E).
Alveolar bone
The alveolar process forms and supports the sockets of the teeth and consists of dense outer cortical bone plates with varying amounts of spongy or cancellous bone between them. The thickness of the cortical laminae varies in different locations. The cancellous bone contains bone trabeculae, the architecture of which is partly genetically determined and partly the result of forces to which teeth are exposed during function or orthodontic treatment. The type of bone through which the tooth is displaced should be considered in the orthodontic treatment plan. Tooth movements in a mesial or distal direction displace the roots through the cancellous bone with its rapid remodelling capacity, in contrast to tooth movements labially or lingually into the thin cortical plates with risk of bony defects.
Physiologic tooth migration
Tissue reactions in the tooth-supporting tissues are connected not only with orthodontic treatment but also with eruption of the teeth and development of the occlusion. The teeth and their supporting tissues have a lifelong ability to adapt to functional demands and hence drift through the alveolar process, a phenomenon called physiologic tooth migration. When the teeth migrate, they bring the supra-alveolar fibres with them, which means remodelling of the PDL and alveolar bone, as illustrated in Figure 9.3. Osteoclasts, associated with the resorptive surface, are seen along the bone wall, towards which the tooth is migrating, while osteoblasts are seen at the bone wall, from which it is moving away (depository side).

Figure 9.3 Physiologic migration in the rat in the interdental area in direction of the black arrow. Resorptive alveolar bone surface (rB, and at open arrows). Depository alveolar bone surface (dB). Older fibres (oF) included in the new bone formation by osteoclasts. New fibrils (nF) near the bone surface and in the middle of the PDL. Osteoblasts (C) and dentin (D).
Because considerable changes in tooth position occur without any orthodontic intervention, knowledge of periodontal remodelling during physiologic tooth migration is of utmost importance when evaluating the long-term effects of an orthodontic treatment.
Orthodontic tooth movements
Basically, no great difference exists between the tissue reactions observed in physiologic tooth migration and those observed in orthodontic tooth movements. Because the teeth are moved more rapidly during treatment, the tissue changes elicited by orthodontic forces are more significant and extensive. Application of a force on the crown of the tooth leads to a response in its surrounding tissue, resulting in an orthodontic tooth movement. Already in 1904, Carl Sandstedt’s studies in dogs demonstrated that tooth movement is a process of resorption at the ‘pressure side’ and deposition of bone at the ‘tension side’ (Sandstedt, 1904). He gave the first description of the glasslike appearance of the compressed tissue, called hyalinisation, which has been associated with a standstill of the tooth movement. But it was not until 1951 that tooth movements attracted wider attention with Kaare Reitan’s classic study ‘The initial tissue reaction incident to orthodontic tooth movement’. He used the dog as an experimental model but also extracted teeth from humans, and histology was his means to address the questions of clinical importance. By means of electron microscopy, Rygh (1972) continued Reitan’s research. Rygh could explain why orthodontic tooth movements might cause damage to the tooth, probably as a sequel of the hyalinisation process. They have been my co-authors in some textbooks, and some of their unique histological material has been used even in the present chapter, by permission of Elsevier Publishers.
Our knowledge of the reactions of the supporting structures is still incomplete. From a clinical point of view it is, however, known that the initial tooth movement is followed by a stop, due to hyalinisation, whereupon the movement will continue.
Initial phase of tooth movement
Application of a force to the crown of a tooth leads to tooth movement within the alveolus and initially a narrowing of the PDL, causing compression in limited areas and by that impedes vascular circulation and cell differentiation (Rygh, 1972). Advanced cellular and vascular changes may occur within a few hours of the application of the orthodontic force (Rygh, 1973). The cells undergo a series of changes, starting with a swelling of the mitochondria and the endoplasmatic reticulum, and continuing with rupture and dissolution of the cell membrane. This leaves only isolated nuclei between compressed fibrous elements (pyknosis) and is the first indication of hyalinisation. Precursor cells along the alveolar bone wall differentiate into osteoclasts and fibroblasts in young humans after 30 to 40 hours (Reitan, 1951).
Hyalinisation phase
At the pressure side, the degradation of the cells and vascular structures gives the tissue a glasslike appearance under the light microscope, termed hyalinisation (Figure 9.4). It is caused partly by anatomic and partly by mechanical factors and is almost unavoidable in clinical orthodontics. Hyalinization represents a sterile necrotic area, characterized by three main stages: degeneration, elimination of destroyed tissue, and establishment of a new tooth attachment.

Figure 9.4 In the drawing to the left (a), location of bone resorption adjacent to the apical third of a maxillary canine. The tooth was moved continuously for 3 weeks. Compensatory formation of osteoid in open marrow spaces (o) and remnants of hyalinised tissue adhering to the root surface (h). Direct bone resorption adjacent to the apical third of the root (D). In (b) to the right, direct resorption with osteoclasts along the bone surface (D) and absence of epithelial remnants in adjacent periodontal tissue, i.e. centre of the formerly cell-free zone (C). The tooth root (R), and the blue arrows show the force and tooth movement direction.
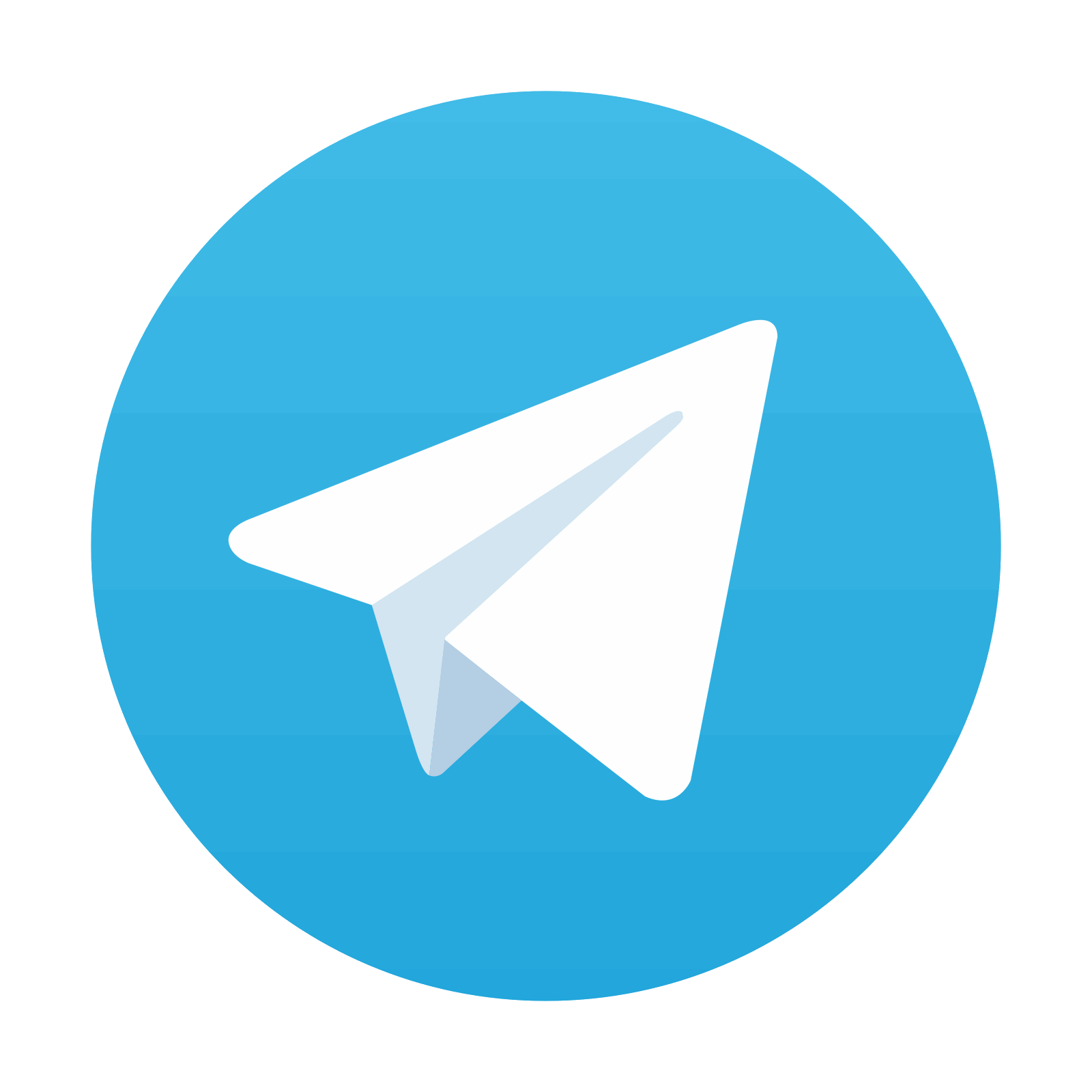
Stay updated, free dental videos. Join our Telegram channel

VIDEdental - Online dental courses
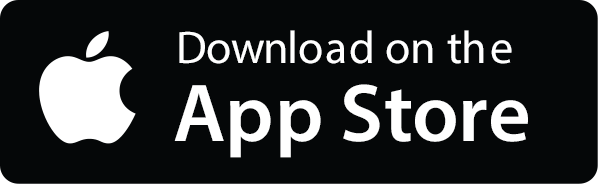
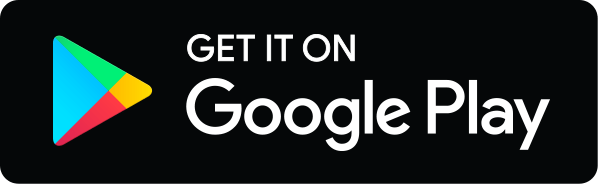