Abstract
Orthognathic surgery is indicated for the treatment of significant skeletal malocclusion and is normally prepared using conventional face bows combined with two-dimensional cephalometric analysis and manually made splints. With recent developments in oral imaging, more orthognathic surgeries are being planned using three-dimensional computer-aided design and manufacturing (3D CAD/CAM) software. The purpose of this study was to present a protocol for the design and 3D printing of final digital occlusal splints based on 3D planning of orthognathic surgery and to validate the accuracy of these splints. The 3D virtual planning was performed in PROPLAN software (Materialise). The required data were then exported into 3-matic software (Materialise) to design the splints, which were 3D printed in biocompatible material using an Objet Connex 350 printer (Stratasys). To validate the accuracy of the splints, the cases of 20 patients undergoing orthognathic surgery were analysed. The splints were assessed clinically and quantitatively by comparing the printed splints to the conventional analogue set-up (clinical standard) and recording the absolute distance errors of three landmarks. The mean absolute distance error was 0.4 mm (standard deviation 0.17 mm), which falls within clinically accepted error margins. The absolute distance error ranged from 0.12 to 0.88 mm.
Successful orthognathic surgery implies the achievement of the correct occlusal relationship, as well as facial balance and harmonization. Splints are used in orthognathic surgery to transfer the preoperative surgical plan and to reposition the jaws into the required optimised occlusion.
Recent developments in dentomaxillofacial imaging using computed tomography (CT) and cone beam CT (CBCT) have allowed further developments in computer-aided design and manufacturing (CAD/CAM) software, leading to a variety of algorithms and software for three-dimensional (3D) virtual planning of orthognathic surgery. The main advantages of 3D planning include the production of a virtual skull and dentition and the direct production of digital and subsequent physical splints, leading to more accurate planning, time savings, and better results.
Previous research has investigated the accuracy of final digital splints in small-scale validation studies with a maximum of 10 cases, or simply through proof of concept. Comparisons were made to conventional manual splints and considered as the ground truth, which is not entirely correct. Some comparison studies have reported mean values, which may underestimate the magnitude of the error, and others have reported the absolute errors.
The aims of this study were to present the steps leading to the design and printing of final digital occlusal splints based on 3D planning of orthognathic surgery and to validate the accuracy of these final digital splints in a larger scale study by comparing the printed splints to the conventional analogue set-up (clinical standard) in terms of absolute errors.
Materials and methods
Virtual 3D planning protocol
The virtual planning protocol for orthognathic surgery was implemented in PROPLAN software version 2.1 (Materialise, Leuven, Belgium). Preoperative imaging was performed with a 128-slice multi-slice spiral CT scanner (Siemens Somatom Definition Flash; Siemens AG, Erlangen, Germany) with settings of 120 kV, 161 mAS, and slice thickness 0.75 mm. DICOM (Digital Imaging and Communications in Medicine) images of the patient were imported into the project and the different components segmented (mandible, maxilla, skull) using a thresholding method. The plaster casts of the upper and lower dentitions were scanned with a high-resolution optical scanner (Activity 885; SmartOptics, Bochum, Germany) and the images saved as stereolithography (STL) files. These casts were registered to the corresponding upper and lower jaws via point-based registration to form composite models of the maxilla and the mandible. The plaster casts were put into the final required position by the surgeon (occlusion cast) and scanned by CBCT. The DICOM images of the occlusion cast were imported into a new PROPLAN project, where a 3D object was made. The virtual osteotomies were then performed and the 3D object of the occlusion cast was registered to the upper jaw and the cut lower jaw was registered to the registered occlusion cast via point-based registration. The registered casts were then exported from the PROPLAN project and imported into 3-matic software (version Medical 10; Materialise) to design the final splint. This was then 3D printed in biocompatible material using an Objet Connex 350 printer (Stratasys, Eden Prairie, MN, USA) with a slice thickness of 0.03 mm.
When this protocol was first implemented, inaccuracies were encountered during the production of the final splints, leading to an inaccurate final occlusion when compared to the final splints made manually and produced in the dental laboratory. Further investigations revealed that the errors in the final splints resulted from a lack of landmarks for the point-based registration and differences in resolution between the occlusion cast scanned by CBCT and the high resolution of the optical scanner (6 μm).
Improved protocol
In order to overcome these issues, the following alterations were made: (1) holes of 1 mm in diameter were drilled into the casts away from the dentition, covering the full arch, prior to the scans. These served as landmarks ( Fig. 1 ). (2) A special acquisition protocol for scanning the casts by CBCT was used. When different protocols for scanning the occlusion casts were investigated, it was found that only this protocol provided fairly visible visualisation of the landmarks. Table 1 and Fig. 2 provide the acquisition settings for the protocol used (special cast protocol) and another high-dose protocol developed for patients (high-dose patient protocol). (3) The registration step was then modified to the use of landmarks distributed across the full arch (minimum of four landmarks: one posterior left, one posterior right, and two in the anterior canine-to-canine region).

Special cast protocol | High-dose patient protocol | |
---|---|---|
CBCT system | Planmeca Promax 3D Max | Planmeca Promax 3D Max |
Tube current (mA) | 12.5 | 7.1 |
Gray scale (bit) | 12 | 12 |
Potential (kV) | 80 | 96 |
Exposure type | Pulsed | Pulsed |
Scan time (s) | 22.5 | 15 |
Voxel size (mm) | 0.10 | 0.15 |
FOV (mm) | 100 × 93 | 80 × 50 |
Detector type | Flat panel | Flat panel |

Validation study
A validation study was conducted to evaluate the accuracy of the final splints produced with the newly proposed protocol. Twenty patient cases were included in the study, with 14 prepared for bilateral sagittal split osteotomy (BSSO) surgery and the other six planned for bimaxillary surgery (BSSO and Le Fort I).
Ethical approval was obtained from the necessary ethics review board. No informed consent was required, as no patient identifiable data were disclosed. All testing and examinations were done on plaster casts.
Every case was assessed clinically by the surgeon performing the operations by checking the relative positions of the midlines of the upper and lower jaws and the contact points, as in the original occlusion cast. Furthermore, a quantitative evaluation based on the analysis of distance errors between landmarks was performed.
For each case, the casts used for splint production were mounted on the printed digital splint and scanned with the special cast CBCT scan protocol (referred to as scan 2), which was identical to the scan of the original occlusion cast (referred to as scan 1). The DICOM images of both scans were then imported into Amira software (FEI, Hillsboro, OR, USA). Scan 2 data were registered onto the lower jaw of scan 1 via voxel-based registration with mutual information. The 3D object of the registered cast was then exported as an STL file and imported into the PROPLAN project of the occlusion cast. Three landmarks were identified on each upper cast from the drilled holes: anterior region, posterior left, and posterior right, as shown in Fig. 3 . The distances between each landmark on the upper cast of the occlusion cast and the corresponding landmark on the digital splint cast were calculated ( Fig. 4 ), resulting in a total of 60 distance errors.

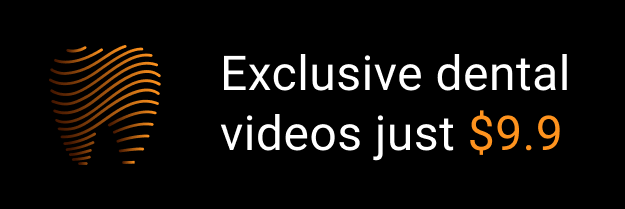