Abstract
The exact boundaries of the upper airway subregions remain undefined. Consequently, anatomical limits vary greatly among different research groups and impede unbiased comparisons. The aim of this study was to provide clinical three-dimensional anatomical limits for the upper airway subregions, translate them into accurate and reliable cephalometric landmarks in cone beam computed tomography (CBCT) data, and validate the proposed measuring protocol. The upper airway of 40 normative individuals aged 23–35 years was evaluated with Dolphin Imaging ® software. An appropriate grey-scale threshold value was pre-calculated. After adapting specific head positioning and virtual orientation protocols, the volume and minimum cross-sectional area of the nasopharynx, oropharynx, and hypopharynx, as previously defined by the authors, were calculated. Intra- and inter-observer reliability was excellent for volumes and moderate for areas. The sexual dimorphism analysis revealed a significantly greater oropharyngeal volume, hypopharyngeal volume, and minimum cross-sectional oropharyngeal area in males than in females. In conclusion, the proposed subregion definition showed technical feasibility and statistical reliability, especially for three-dimensional calculations. The reliability of two-dimensional calculations may be increased with improved head positioning during CBCT scanning and subsequent virtual head orientation. Standardization of the proposed anatomical limits has the potential to homogenize upper airway subregion analysis and permit comparisons among future studies.
During the past decade, cone beam computed tomography (CBCT) has become a widely acknowledged tool for oral and maxillofacial diagnosis and treatment planning. Besides its irrefutable advantages of a lower effective radiation dose, lower costs, easy access, and shorter acquisition times in comparison to conventional multidetector CT (MDCT), its high spatial resolution between soft tissue and empty space provides an unprecedented method to analyse the airway three-dimensionally.
Recently, a systematic review of the scientific literature on CBCT imaging and analysis of the upper airway was performed. It was found that three-dimensional (3D) analysis of the upper airway using CBCT is accurate and reliable, although important obstacles still need to be addressed. These include the impact of respiration phase, influence of tongue position and mandible morphology, longitudinal and cross-sectional 3D CBCT upper airway evaluation, and 3D CBCT definition of the anatomical boundaries of the upper airway. Regarding the latter aspect, it was found that the anatomical definitions of the airway subregions from the nasal and oral cavities to the larynx were extremely variable among different authors. What is more, many studies included in the systematic review did not specify the anatomical boundaries of the particular region of interest in their methodology. As a result, comparisons among studies, even between those claiming to analyse the same airway subregion, is often difficult and prone to error.
In this context, the objectives of this paper were the following: (1) to provide 3D anatomical limits for the different subregions of the upper (pharyngeal) airway; (2) to precisely translate these anatomical limits into cephalometric landmarks that can be used reliably in CBCT analysis; and (3) to validate a measuring protocol for the predefined subregions of the upper airway using widespread third-party software.
Materials and methods
The study protocol obtained local ethics review board approval. CBCT scans from 40 healthy individuals (20 females and 20 males) were prospectively obtained. Inclusion criteria were the following: (1) Caucasian; (2) age between 23 and 35 years; (3) Angle Class I molar relationship; (4) class I facial profile and no clinically obvious facial asymmetries; (5) normal body mass index (BMI); (6) no prior surgery in the head and neck. Individuals at risk of sleep-disordered breathing according to the Epworth Sleepiness Scale, and with congenital or acquired craniofacial anomalies were excluded from the evaluation.
Scans were performed using a standardized scanning protocol (i-CAT™, Imaging Sciences International, Inc., Hatfield, PA, USA) between March and May 2011. The period of data acquisition was strictly limited to these dates in order to ensure the same calibration parameters. In addition, calibration of the CBCT apparatus was checked daily during the data acquisition period. Vertical scanning was performed in ‘extended field’ modus (field of view (FOV) 17 cm diameter, 22 cm height; scan time 2 × 20 s; voxel size 0.4 mm) at 120 kV (according to DICOM field 0018,0060 KVP) and 48 mA (according to DICOM field 0018,1151 X-ray tube current). Special attention was paid to proper patient positioning for the scan: patients were instructed to sit upright and position themselves in a natural head position (NHP) with the help of a mirror and a laser beam. They were also taught to place the mandible at maximum intercuspation without the use of a wafer in order to avoid tongue displacement. They were asked to rest the tongue in a relaxed position, breathe lightly, and avoid any other motor reaction.
The DICOM (Digital Imaging and Communications in Medicine) data were processed using third-party software (Dolphin Imaging ® , version 11.0, Chatsworth, CA, USA). Prior to airway evaluation, all 40 CBCT databases were preliminarily assessed in order to determine the most appropriate threshold value for upper airway analysis in this study. The automatically fixed threshold value was manually increased for each dataset until the nasopharyngeal airway was adequately depicted. The average threshold value of all the patients thus defined was 70 (range 48–81) and was established as the reference threshold for this study.
Subsequently, all CBCT datasets were evaluated separately by the two investigators of this study (RGM, GRJS) in order to determine the inter-observer error. In addition, each patient was reassessed separately by each investigator 4 weeks after the first evaluation with the aim of establishing the intra-observer error. Separate spreadsheets were used to record the first and second measurements in order to avoid analysis bias from the first results.
On multiple planar reconstruction images, the skull was reoriented to the Frankfort horizontal (FH) using the following guidelines: (1) In the frontal view, the mid-sagittal plane was fixed through the centre of the anterior nasal spine (ANS) and the axial plane was constructed through both infraorbitale skeletal landmarks. When a bifid ANS was encountered in the frontal view, the mid-sagittal plane was established at the midpoint between the two bony prominences. (2) In the right sagittal view, the axial plane was placed through the right porion and right infraorbitale landmarks. For standardization, the left sagittal view was not processed in order to avoid orientation problems due to asymmetrically positioned porions. (3) In the transversal view (patient facing down or endocranial view), the mid-sagittal plane was constructed through crista galli and basion. In the opposite transversal view (patient facing up or exocranial view), it was ensured that no ‘yaw’ (transversal rotation) of the mandible or the zygomatic arches was present. Figure 1 illustrates the coronal, sagittal, and axial reconstruction slices after head orientation in a male patient.

In the sagittal view, the cranial base angle and cranio-cervical inclination were also measured. The cranial base angle of the analysed population sample was used for descriptive purposes; the cranio-cervical inclination was evaluated in order to test the homogeneity of head inclination during scanning, and hence the effectiveness of our head positioning protocol. The cranial base angle was measured between the points nasion, sella and basion, as described by Enlow ( Fig. 2 ). Following other author recommendations, the cranio-cervical inclination was measured as the angle between the line formed by connecting C2od (tangent point at the most superior–posterior point of the odontoid process of C2) and C2ip (the most inferior–posterior point of the body of C2) and the SN line (sella–nasion) ( Fig. 3 ).


The process of airway segmentation was systematized as follows: A virtual marker for the region-of-interest definition, referred to in the software as the ‘seed point’, was placed in the airway region immediately anterior to C2ia (the most inferior–anterior point of the body of C2). The automatic threshold thus determined by the program was manually increased to the value of our predefined airway sensitivity (70). Subsequently, the limits of the nasopharynx, oropharynx, and hypopharynx were outlined by the investigator. Within each separate region, the software calculated the total airway volume (mm 3 ) and minimum cross-sectional area (mm 2 ) automatically. The anatomical limits of each subregion were established by the authors according to clinical experience and previous work. The ensuing technical boundaries were determined with the aim of defining reproducible limits based on reliable anatomical cephalometric landmarks that are easily identifiable on CBCT studies. Table 1 details the anatomical and technical boundaries of each subregion of the upper airway. Figures 4–6 exemplify the independent evaluation of the nasopharynx, oropharynx, and hypopharynx according to these limits. In the case of the hypopharynx, care was taken to add a second virtual ‘seed point’ when the epiglottis was positioned halfway across the air space of the airway, in order to adequately include all the air within the predefined limits ( Fig. 7 ).
Region | Limits | Anatomical | Technical |
---|---|---|---|
Nasopharynx | Anterior | Frontal plane perpendicular to FH passing through PNS | = |
Posterior | Soft tissue contour of the pharyngeal wall | Frontal plane perpendicular to FH passing through C2sp | |
Upper | Soft tissue contour of the pharyngeal wall | Transversal plane parallel to FH passing through the root of the clivus | |
Lower | Plane parallel to FH passing through PNS and extended to the posterior wall of the pharynx | = | |
Lateral | Soft tissue contour of the pharyngeal lateral walls | Sagittal plane perpendicular to FH passing through the lateral walls of the maxillary sinus | |
Oropharynx | Anterior | Frontal plane perpendicular to FH passing through PNS | = |
Posterior | Soft tissue contour of the pharyngeal wall | Frontal plane perpendicular to FH passing through C2sp | |
Upper | Plane parallel to FH passing through PNS and extended to the posterior wall of the pharynx | = | |
Lower | Plane parallel to FH plane passing through C3ai | = | |
Lateral | Soft tissue contour of the pharyngeal lateral walls | Sagittal plane perpendicular to FH passing through the lateral walls of the maxillary sinus | |
Hypopharynx | Anterior | Frontal plane perpendicular to FH passing through PNS | = |
Posterior | Soft tissue contour of the pharyngeal wall | Frontal plane perpendicular to FH passing through C2sp | |
Upper | Plane parallel to FH plane passing through C3ai | = | |
Lower | Plane parallel to FH connecting the base of the epiglottis to the entrance to the oesophagus | Plane parallel to FH connecting the base of the epiglottis to C4ai | |
Lateral | Soft tissue contour of the pharyngeal lateral walls | Sagittal plane perpendicular to FH passing through the lateral walls of the maxillary sinus |




The statistical analysis was performed using SPSS for Windows, version 19.0 (SPSS, Chicago, IL, USA). Reproducibility was evaluated by calculating the inter- and intra-observer error with Dahlberg’s formula. Intraclass correlation coefficients (ICC) were obtained. A descriptive analysis was used to obtain preliminary normative data for the sample as a whole and also when stratified by gender. After corroborating the normal distribution of the sample with the Kolmogorov–Smirnov test, a Student’s t -test for independent samples was used to compare the sex groups. Repeated measures analysis of variance (ANOVA) was used to compare the three subregions of the upper airway. Friedman’s test was used to stratify the analysis by gender. Statistical significance was fixed at α = 0.05.
Results
Of the initial 40 patients whose CBCTs were analysed, one male and one female were excluded because of inadequate localization of the crista galli for skull orientation purposes, and three females were excluded for pathological findings on radiology (hypertrophic adenoids in one case and inadequately ventilated sinuses in the two others). Thus, the studied sample comprised 35 subjects, of whom 19 were male (54.3%) and 16 female (45.7%). Adequate hypopharyngeal analysis was impossible for three of the 19 males because the FOV did not include the most anterior–inferior point of the body of C4 (C4ai); hence, only the nasopharynx and oropharynx were quantified for these three male patients.
Tables 2 and 3 summarize the intra- and inter-observer reproducibility analysis, respectively. Whereas the intra-observer error for volumetric calculations was below 4% for both investigators, it ranged between 11% and 20% for minimum cross-sectional area calculations. In terms of ICC, volumetric measurements ranged between 0.981 and 0.999 and thus were highly reproducible, but area measurements ranged between 0.780 and 0.937 and hence were moderately reproducible. The fact that all confidence intervals included 0, ruled out a bias tendency when performing the second measurement for both investigators. The inter-observer error analysis revealed excellent reproducibility for cranial base angle and cranio-cervical inclination measurements (ICC 0.983 and 0.992, respectively), good reproducibility for volumetric calculations (ICC between 0.986 and 0.998), and again moderate reproducibility for cross-sectional area calculations (ICC between 0.837 and 0.876).
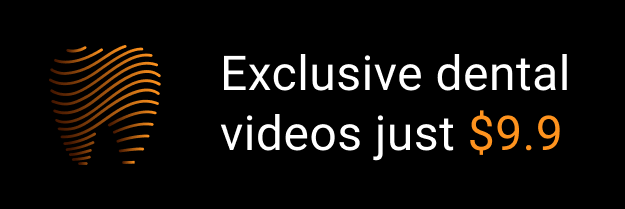