Abstract
Objective
In recent years, numerous studies have analyzed the role of bioactive glass (BAG) as remineralizing additives in dental restorative composites. This current review provides a critical analysis of the existing literature, particularly focusing on BAGs prepared via the melt-quench route that form an “apatite-like” phase when immersed in physiological-like solutions.
Methods
Online databases (Science Direct, PubMed and Google Scholar) were used to collect data published from 1962 to 2020. The research papers were analyzed and the relevant papers were selected for this review. Sol–gel BAGs were not included in this review since it is not a cost-effective manufacturing technique that can be upscaled and is difficult to incorporate fluoride.
Results
BAGs release Ca 2+ , PO 4 3− and F − ions, raise the pH and form apatite. There are numerous published papers on the bioactivity of BAGs, but the different glass compositions, volume fractions, particle sizes, immersion media, time points, and the characterization techniques used, make comparison difficult. Several papers only use certain characterization techniques that do not provide a full picture of the behavior of the glass. It was noted that in most studies, mechanical properties were measured on dry samples, which does not replicate the conditions in the oral environment. Therefore, it is recommended that samples should be immersed for longer time periods in physiological solutions to mimic clinical environments.
Significance
BAGs present major benefits in dentistry, especially their capacity to form apatite, which could potentially fill any marginal gaps produced due to polymerization shrinkage.
1
Introduction
In 2013, the intergovernmental negotiating committee agreed on the Minamata Convention on Mercury due to the health concerns regarding mercury exposure to people and the environment [ ]. Resin-based composites (RBCs) are the commonly used alternative to amalgam restorations in posterior teeth due to the absence of mercury emissions and their improved aesthetics. They are generally based on a resin matrix and an inert filler. However, the major issue of current RBCs is the formation of a marginal gap upon polymerization ( Fig. 1 ) [ ].

When a monomer is converted into a polymer, polymerization shrinkage of 1.7–6 vol% [ , ] occurs, depending on the resin composition and the filler volume fraction. This shrinkage creates a marginal gap between the restorative material and the tooth surface, which is sufficient enough for marginal leakage to occur, allowing for the penetration of bacteria, fluids and ions into the gap between the restorative material and cavity walls, resulting in the failure of the dental composite [ ].
RBCs do not generally release calcium and phosphate ions or react to form hydroxyapatite (HAp) on the surface of the tooth unless a reactive glass filler is used [ ]. The aim of bioactive glasses (BAGs) are to form an apatite-like phase in the marginal gap providing a marginal seal, prevent acid attack by raising the local pH, reducing the likelihood of failure of the composite. Therefore, bioactive dental composites may increase the longevity and clinical outcomes of restorations [ ]. These materials also aim to aid in remineralization of hard carious dentin and therefore require less invasive and minimal cavity preparation, as less removal of the carious dentin will be required, and atraumatic restorative treatment type can be performed.
In recent years, numerous studies have analyzed the role of bioactive glass (BAG) as remineralizing additives in dental restorative composites. This current review provides a critical analysis of the existing literature, particularly focusing on BAGs prepared via the melt-quench route that form an “apatite-like” phase when immersed in physiological-like solutions.
2
Bioactivity
There are many definitions for the term ‘bioactive’ discussed in an editorial paper by Vallittu et al., which considers both the medical and dental field [ ]. The authors suggest that this term is used too often for advertising purposes and that a consensus should be made by dental materials opinion leaders. It is stated that a material that forms a bond between the tissues and material is bioactive [ ], but other definitions suggest that a material is bioactive if it releases ions and induces the formation of HAp when in contact with physiological fluids [ , ]. In 2018, 50 key opinion leaders discussed the definition of bioactivity in the context of restorative materials and stated that a material can also be bioactive if it contains “component(s) that dissolve and have antimicrobial activity (this includes high-pH materials)” [ ]. However, according to this definition, amalgam fillings will be considered bioactive since they release beneficial ions such as silver (Ag + ), tin (Sn 2+ ) and copper (Cu 2+ ), even though they do not induce the formation of apatite.
A recently published review on bioactive materials have classed materials containing calcium phosphate particles to be bioactive [ ], as they have been reported to release Ca 2+ and PO 4 3− , have a remineralizing capacity and form apatite [ ]. However, it is important to note that when they are immersed in physiological fluids, they dissolve and will form pores in the material due to the dissolution of the calcium phosphate particles. This would subsequently result in a reduction of the mechanical properties. This does not occur with BAG fillers, as shown in the SEM images by Al-eesa et al. where the glass particles were very clearly reacting upon immersion into physiological fluid, but no pores were formed ( Fig. 2 ) [ ]. It is also very difficult to manipulate the refractive indices of crystalline calcium phosphates, which hinders achieving translucency and good final aesthetics.

Glass ionomer cements (GICs) are also considered bioactive according to several research papers due to their capacity to release F − , inhibit demineralization and promote remineralization [ ]. The glasses used in GICs have often been incorporated into composites. Here it is important to note that these glasses degrade under acidic conditions (pH 4–5) by hydrolysis of Al–O–Si bonds and they release only small amounts of F − Ca 2+ /Sr 2+ and PO 4 3− ions unless the pH is acidic [ ]. They do not significantly degrade under neutral conditions, which is where remineralization and apatite formation are likely to occur. Ionomer glasses do not have the capacity to form apatite upon immersion in physiological fluids, unlike BAGs. Thus, they are not as attractive as BAGs for incorporation into RBCs.
The aim of this paper is to review the published literature on bioactive glass (BAG) containing restorative composites by discussing the BAG composition, effect of network connectivity, refractive indices, silanization, bonding and the particle size of the BAG fillers on the bioactivity of the restorative composite. To maintain the focus of this review paper, only melt-quenched BAGs used for applications such as varnishes, adhesives, bonding agents, air abrasion and filling materials will be discussed. For the purposes of this review, a BAG is defined as a glass capable of forming an apatite layer when immersed in physiological solutions.
3
Methods
Online databases (Science Direct, PubMed and Google Scholar) were used to collect data published from 1962 to 2020. The research papers were analyzed and the relevant papers were selected for this review. Sol–gel BAGs were not included in this review as it is not a cost-effective manufacturing technique that can be upscaled. Furthermore, sol–gel glasses exhibit fluorine volatilization.
4
Bioactive glasses
Hench et al. first developed BAGs in 1969 and suggested that a BAG dissolves or degrades in physiological-like fluids, forming hydroxycarbonated apatite (HCA) [ ]. They were originally developed as bone substitutes, but in the last fifteen years have been increasingly used as an additive in remineralizing desensitizing hypersensitivity toothpastes [ ]. These glass compositions are based on a SiO 2 –P 2 O 5 –CaO–Na 2 O system. Some typical BAG compositions are provided in Table 1 .
Glass | mol % | NC | RI | ||||
---|---|---|---|---|---|---|---|
SiO 2 | P 2 O 5 | CaO | Na 2 O | CaF 2 | |||
45S5 [ ] | 46.10 | 2.60 | 26.90 | 24.40 | 0.00 | 2.11 | 1.56 |
45S5F [ ] | 46.10 | 2.60 | 16.90 | 24.40 | 10.00 | 2.55 | 1.53 |
S53P4 [ ] | 53.80 | 1.70 | 21.80 | 22.70 | 0.00 | 2.54 | 1.54 |
BAG-F [ ] | 42.70 | 4.00 | 26.20 | 26.10 | 1.00 | 2.11 | 1.55 |
BioMinF [ ] | 36.00–40.00 | 4.00−6.00 | 28.00–30.00 | 22.00–24.00 | 1.50–3.00 | 2.16 | 1.52 |
QMNA1 [ ] | 35.00 | 6.06 | 43.00 | 6.00 | 10.00 | 2.24 | 1.57 |
Example 7 (Cention N) [ ] | 48.00 | 0.00 | 31.00 | 8.00 | 10.00 | 2.38 | 1.50 |
Hench explained the mechanism of dissolution and apatite formation ( Fig. 3 ) in many stages [ ]. However, stages 1 and 2 does not specify the type of glass present, therefore one can assume that window glass, which is a Q 3 /Q 4 type glass since there are three to four bridging oxygens bonded to the silicon, can be bioactive according to this mechanism. Yet, it was later observed that glasses are bioactive when the network connectivity (NC) is around 2 (Q 2 type glass, where there are two bridging oxygens bonded to the silicon). Therefore, bioactivity is highly dependent on NC (discussed further in Section 5 ). Moreover, this mechanism only considers Na + cations, when in reality other cations will be present, most commonly Ca 2+ . The addition of small amounts of CaF 2 to the glass composition and its capacity to form fluorapatite (FAp) [ ] is not considered in this mechanism, and therefore this mechanism is restricted to the formation of HCA, although many different apatite phases can be present such as FAp, chlorapatite, HAp, strontium-substituted apatite, and mixed apatites.

FAp, Ca 5 (PO 4 ) 3 F, is more acid resistant and less degradable than both HCA and HAp as the OH − ion in apatite distorts the lattice slightly due to its large ionic radius, whereas the F − ion has a smaller ionic radius, and fits perfectly in the hexagonal apatite lattice perfectly, thus FAp is more chemically and thermodynamically stable [ ]. This makes fluoride attractive to dentistry, as the oral environment is often exposed to acidic conditions [ ]. The critical pH respectively for HAp and FAp is approximately 5.5 and 4.5 respectively, depending on the individual [ ].
5
Network connectivity model
Hill et al. questioned the BAG degradation mechanism in Fig. 3 and developed a network connectivity (NC) model (NC old ) for predicting the dissolution and apatite forming capability of BAG fillers (Eq. (1) ) [ ]. This is important in BAG design, as the NC will determine the overall dissolution and bioactive properties of the final restorative composite material. This model treats BAGs as linear silicon-based polymers and calculates the NC of the silicate network. In a pure SiO 2 glass, each silicon is linked via a bridging oxygen (a Si–O–Si) to four other silicons, providing a NC of 4.0 (Q 4 ). When network modifying oxides (NMOs) are introduced (such as Na + Ca 2+ , Sr 2+ or Mg 2+ ), the glass network is disrupted, giving rise to non-bridging oxygens (NBOs). In a calcium metasilicate glass containing equimolar ratios of CaO and SiO 2 , each CaO introduces two NBOs (O − –Si–O–), breaking up the three-dimensional network. Each silicon becomes linked to two other oxygens and the NC = 2.0 (Q 2 glass). The glass corresponds to a linear silicate chain and may be considered as a silicate polymer of [SiO 3 2− ] n . Reducing the CaO content results in the NC increasing beyond 2.0 and the polymeric chains become increasingly cross-linked.
NCold=4SiO2-2[∑NMO][SiO2]
Eq. (1) : The original NC model before discovering phosphate is present as orthophosphate. NMO is the network modifying oxide concentration (in mol%) [ ].
Based on this concept, dissolution and bioactivity would theoretically be expected to be highly dependent on the NC and an average sharp cut off for bioactivity would be expected at a NC of 2.0 assuming that only the linear crosslinked chains are present. Experimentally, dissolution and bioactivity often extend above a NC of 2.0, towards a cut off value of 2.4 although Edén et al. stated that bioactivity is present up to a NC of 2.6 [ , ].
The NC old model predicted the bioactivity and apatite forming capacity reasonably well for simple silicate glasses that are phosphate-free but does not adequately work for glasses with higher phosphate contents than the 45S5 glass or compositions containing MgO or ZnO. Another problem when calculating NC is that structural assumptions must be made about the role of the various oxides. In the NC old it was assumed that the phosphate formed Si–O–P bonds, however O’Donnell et al. [ ] later showed the phosphorus formed, orthophosphate (PO 4 3− ), was locally charge balanced by Ca 2+ and Na + ions and was therefore not part of the silicate network. This lead to the modification of the NC model (NC new ) in Eq. (2) , considering that phosphate is present as orthophosphate (by Eq. (1) ) [ ]. Note that when NC is discussed from this point on, it refers to NC new . Increasing the NMOs at the expense of SiO 2 decreases the NC, thus making the glass more degradable.
NCnew=(4SiO2-2[∑NMO]+6P2O5)[SiO2]
Eq. (2) : The modified NC model after discovering phosphate is present as orthophosphate. NMO is the network modifying oxide concentration (in mol%) [ ].
O’Donnell et al. investigated the structural role of phosphate in BAGs and the influence of phosphate on the physical properties, dissolution behavior and capacity of the glasses to form apatite in simulated body fluid (SBF) [ ]. Phosphorus was shown to exist as orthophosphate and when the phosphate content was increased, whilst maintaining a fixed NC by adding additional CaO and Na 2 O, the time to form an apatite-like phase decreased and the amount of apatite present increased. Edén et al. developed a split network model and calculated values that correspond exactly to those for the modified NC and also demonstrated the importance of the phosphate content by stating that if phosphorus remains as orthophosphate and the NC remains constant, the bioactivity is enhanced with increasing phosphate content of the BAG. [ ].
Attempts were also made to produce high phosphate content BAGs with fluoride, but problems were encountered with crystallization of the compositions. Mneimne et al. reported that the amount of phosphate plays a crucial role in apatite formation since increasing the phosphate content of the glass whilst keeping the sodium content low and NC constant increases the amount and speed of apatite formation [ ]. These investigators also reported that a low phosphate-containing glass takes approximately three days to start forming apatite, whereas high phosphate-containing glasses take less than a day.
6
Refractive index of bioactive glass fillers
Having a refractive index (RI) match (within 0.02) between the resin and BAG fillers is important to eliminate the light scattering at the glass-resin interface both for the efficient light curing and the final aesthetics of the composite to give translucency. Most commonly used dental resins have a RI range from 1.47 to 1.56, depending on the composition of the resin [ ]. In the case of fluoride-free glasses, the RI of the glass can be calculated (Eq. (3) ) using Appen factors ( Table 2 ), which are empirically derived factors calculated based on previous measurements of RI.
RI=∑i=1nnd,ici100
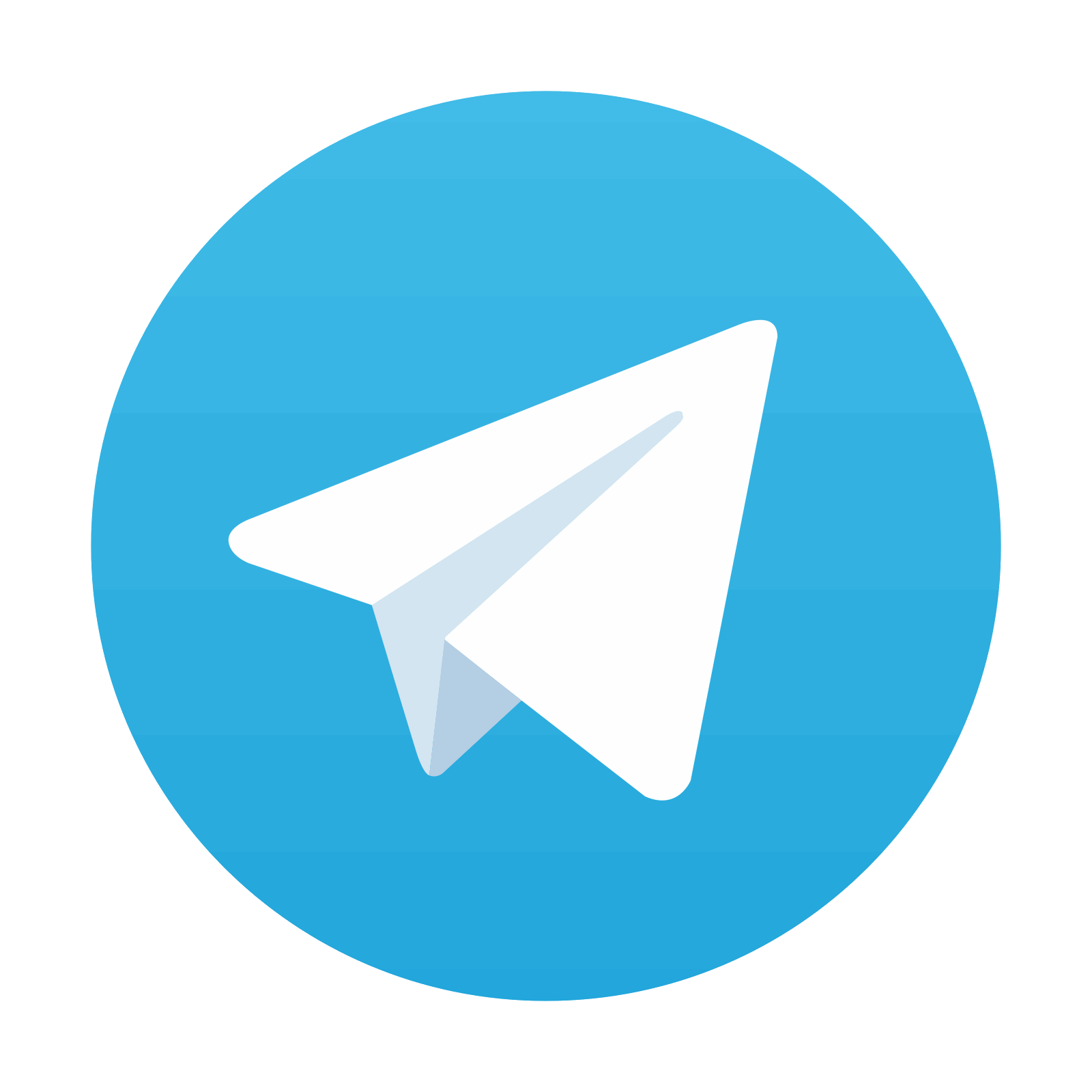
Stay updated, free dental videos. Join our Telegram channel

VIDEdental - Online dental courses
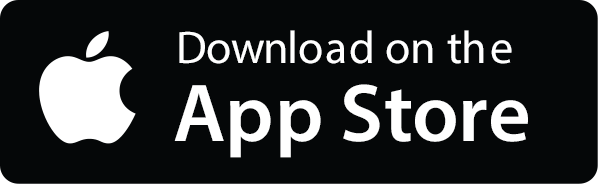
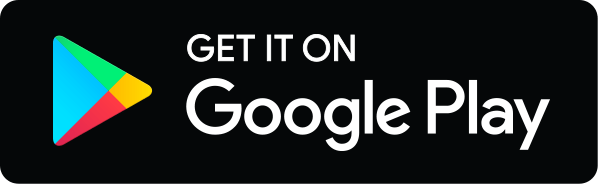
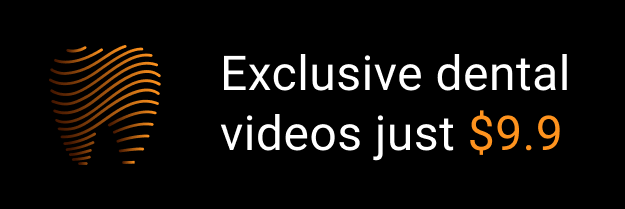