Abstract
Objectives
To investigate the role of enamel thickness and refractive index ( n ) on tooth colour.
Methods
The colour and enamel thickness of fifteen extracted human central incisors were determined according to CIELab colour scale using spectrophotometer (Crystaleye) and swept-source optical coherence tomography (SS-OCT), respectively. Subsequently, labial enamel was trimmed by approximately 100 μm, and the colour and remaining enamel thickness were investigated again. This cycle was repeated until dentin appeared. Enamel blocks were prepared from the same teeth and their n were obtained using SS-OCT. Multiple regression analysis was performed to reveal any effects of enamel thickness and n on colour difference (ΔE 00 ) and differences in colour parameters with CIELCh and CIELab colour scales.
Results
Multiple regression analysis revealed that enamel thickness (p = 0.02) and n of enamel (p < 0.001) were statistically significant predictors of ΔE 00 after complete enamel trimming. The n was also a significant predictor of ΔH’ (p = 0.01). Enamel thickness and n were not statistically significant predictors of ΔL’, ΔC’, Δa* and Δb*.
Conclusions
Enamel affected tooth colour, in which n was a statistically significant predictor for tooth colour change.
Clinical significance
Understanding the role of enamel in tooth colour could contribute to development of aesthetic restorative materials that mimic the colour of natural tooth with minimal reduction of the existing enamel.
1
Introduction
Aesthetic treatments have become widely requested as the standard of care in restorative dentistry. Among factors necessary for aesthetic restorations, tooth colour evaluation is an important step not only for patients who wish to enhance their smile or appearance, but also for dentists who would like to select appropriate materials with the correct shade and provide the best treatment . The perception of tooth colour is a complex phenomenon and can be influenced by a number of factors, including the visual state of the observer, the context in which the tooth is viewed, the type of incident light, and the optical properties and colour in dental tissue . Optical properties are intrinsic properties resulting from of a combination of various factors, such as specular and diffuse reflection (light scattering) at the surface, specular transmission of light through the tissue, and absorption and scattering of light within the tissue . These properties are in turn affected by the composition and histological structure of the tissue .
Coronal dentine is covered by an enamel layer with thicknesses that vary according to the type of the tooth and anatomical location, being approximately 1.0 mm on the average . Nevertheless, the colour of a tooth is largely determined by properties of dentine . On the other hand, the optical properties of enamel have been widely studied, since enamel has minor, yet noticeable effects on the colour and translucency of natural tooth . The thickness and translucency property of enamel might control chromatic influence of dentine on natural tooth appearance. Moreover, direct restorations placed over remaining dentin will need to mimic the properties of enamel optically. It is well known that enamel has fluorescence and opalescence . While fluorescence does not contribute to visually observed colour of the tooth , enamel affects tooth colour through opalescence, which is defined as scattering of light in the blue wavelength range . Recently, it was demonstrated that tooth colour parameters (hue, chroma, lightness) were influenced by variability in the mineral composition of enamel, namely the size and degree of the carbonization of the enamel hydroxyapatite (HA) crystals . On the other hand, mineral content of enamel was reported to affect its local refractive index ( n ) , which is associated with light scattering properties inside the tissue. However, there is no published research on the effects that n of enamel and its thickness may have on tooth colour.
It was recently reported that the n of dental hard tissues could be investigated by swept source optical coherence tomography (SS-OCT) . SS-OCT is a rapidly growing non-invasive interferometric technique, which enables cross-sectional imaging of internal biological structures by differentiating between scattered and transmitted or reflected photons using broad-band, near-infrared (near-IR) light sources on micrometer scale resolution . SS-OCT has been validated to accurately determine the optical thickness of dental tissue in a nondestructive manner . Therefore, the purpose of this study was to investigate the role of enamel thickness and n , using SS-OCT, on human tooth colour. In this study, tooth colour evaluation was performed by measurement of the CIELab L* (lightness), a* (green-red coordinate) and b* (blue-yellow coordinate) value, and by calculation of the CIE2000 ΔL’ (differences in lightness), ΔC’ (differences in chroma), ΔH’ (differences in hue) and ΔE 00 (colour difference). The null hypotheses tested were that the enamel thickness and n are not predictors of tooth colour change (ΔE 00 ) after trimming of enamel, and also they are not predictors of ΔL’, ΔC’, ΔH’, Δa* and Δb* after trimming of enamel.
2
Materials and methods
2.1
Measurement of colour of the teeth
Fifteen extracted human central incisors, without endodontic treatment or restorations and stored at 4 °C water were used in this study, according to a protocol approved by the Human Research Ethics Committee, Tokyo Medical and Dental University, Japan (No. 725). The teeth were placed in a jaw model; in order to enable repositioning in the same location, molds were fabricated from silicon putty (EXAFINE PUTTY TYPE, GC, Tokyo, Japan). The jaw model was placed in a black box (inspection kit, OLYMPUS, Tokyo, Japan) which was shielded from external light. The colour of all these intact teeth were then measured using a dental spectrophotometer (Crystaleye, OLYMPUS, Tokyo, Japan) with capture time of 0.2 s, in which 7 light emitting diodes (LEDs) are used as an illumination source corresponding to the standard illuminant D65 with 45°/0° geometry. Prior to data acquisition, the spectrophotometer was calibrated using a calibration plate (OLYMPUS, Tokyo, Japan) and positioned at a consistent distance of 16 mm from the specimen surface using the special contact cap for object measurement. All measurements were performed by the same researcher who was extensively trained. The spectral data was acquired from the captured image of the tooth . The reflectance values for each pixel in the range of 400–700 nm with 1 nm intervals were transferred from the spectrometer to a personal computer with a Crystaleye software (Crystaleye Application version 1.4, Olympus, Tokyo, Japan) . In this study, evaluation area (1.0 mm × 1.0 mm) was identified on the captured image of the tooth ( Fig. 1 a), in which the colour was analysed using Commission Internationale de lÉclairage colour coordinates L* (lightness), a* (green-red coordinate) and b* (blue-yellow coordinate), known as CIELAB colour coordinates . The Crystaleye software also provided tooth colour shade in minimum area of 0.5 mm × 0.5 mm by comparing the database of VITA Classical (VITA, Bad Säckingen, Germany) with the data acquired from the digital image of the teeth.

2.2
Optical enamel thickness measurement using SS-OCT
The optical enamel thickness of the teeth were investigated using SS-OCT (OCT-2000, Santec Co., Komaki, Japan) . The system incorporates a high-speed frequency swept tuneable external cavity laser with wavelength range of 1260–1360 nm (centred at 1310 nm) at a 20-kHz sweep rate. The laser beam is projected over the object, and the backscattered light is coupled back to the SS-OCT system, in which the interference signal is digitized in time scale, and then analysed in the Fourier domain to reveal the depth information of the object. The axial and lateral resolutions of the system in air were 11 and 17 μm, respectively. The system acquired the image data (B-scan) in 0.3 s, including the processing time. The imaging window range in this study was 5 mm (width) by 6.6 mm (height) forming a 2000 × 1019 pixel image. The sensitivity of this system and the shot-noise limited sensitivity were 106 and 119 dB, respectively . Enamel thickness measurement location was a fixed window of interest (1.0 mm × 1.0 mm) on labial mid-coronal surface ( Fig. 1 a). Image analysis software (ImageJ 1.42q; National Institutes of Health, Bethesda, MD, USA) was used to perform SS-OCT image analysis.
2.3
Evaluation of colour and remaining enamel thickness after trimming
Labial enamel of the teeth were trimmed to remove approximately 100 μm thickness using 600-grit and 1000-grit silicon carbide (SiC) papers (Sankyo, Saitama, Japan) under running water. The surface was polished using 1500-grit SiC papers under running water and the optical thickness of remaining labial enamel at the window was investigated on SS-OCT images. Digital colour images of the teeth were also obtained by Crystaleye after their repositioning on the jaw model using the silicon putty mold. Enamel trimming, and optical thickness measurement and spectrophotometer colour determination cycles were repeated until enamel was completely removed and dentine appeared. Each tooth was kept moist at all times in order to avoid its dehydration. In order to evaluate the colour change with enamel trimming, CIEDE2000 color difference (ΔE 00 ) between before and after enamel trimming at each trimming step were calculated via Microsoft Excel spreadsheet at Sharma’s website . The CIEDE2000 color-difference formula is based on the CIELAB color space . Given a pair of color values in CIELAB space L* 1 ,a* 1 ,b* 1 and L* 2 ,a* 2 ,b* 2 , we denote the CIEDE2000 color difference between them . The CIEDE2000 color difference formula is given as follows :
Δ E 00 = ( Δ L ′ K L S L ) 2 + ( Δ C ′ K C S C ) 2 + ( Δ H ′ K H S H ) 2 + R T ( Δ C ′ K C S C ) ( Δ H ′ K H S H )
ΔL’, ΔC’, and ΔH’ between before and after enamel trimming at each trimming step are the differences in lightness, chroma, and hue between before and after enamel trimming and R T is a function (the so-called rotation function) that accounts for the interaction between chroma and hue differences in the blue region. S L , S C , and S H are the weighing functions for the lightness, chroma, and hue components, respectively. K L , K C , and K H are the parametric factors to be adjusted according to different viewing parameters.
ΔL’, ΔC’, and ΔH’ were calculated in the following equations as two step :
1. Calculate: <SPAN role=presentation tabIndex=0 id=MathJax-Element-2-Frame class=MathJax style="POSITION: relative" data-mathml="Ci’,hi'”>C′i,h′iCi’,hi’
C i ‘ , h i ‘
C i , a b * = ( a i * ) 2 + ( b i * ) 2 i = 1 , 2
C ¯ a b * = C 1 , a b * + C 2 , a b * 2
G = 0.5 ( 1 − C ¯ a b * 7 C ¯ a b * 7 + 25 7 )
a i ‘ = ( 1 + G ) a i * i = 1 , 2
C i ‘ = ( a i ‘ ) 2 + ( b i * ) 2 i = 1 , 2
h i ‘ = { 0 b i * = a i ‘ = 0 tan − 1 ( b i * , a i ‘ ) o t h e r w i s e i = 1 , 2
2. Calculate: ΔL’, ΔC’, ΔH’
Δ L ′ = L 2 * − L 1 *
Δ C ′ = C 2 ‘ − C 1 ‘
Δ h ′ = { 0 h 2 ‘ − h 1 ‘ ( h 2 ‘ − h 1 ‘ ) − 360 ( h 2 ‘ − h 1 ‘ ) + 360 C 1 ‘ C 2 ‘ = 0 C 1 ‘ C 2 ‘ ≠ 0 ; | h 2 ‘ − h 1 ‘ | ≤ 180 ° C 1 ‘ C 2 ‘ ≠ 0 ; ( h 2 ‘ − h 1 ‘ ) > 180 ° C 1 ‘ C 2 ‘ ≠ 0 ; ( h 2 ‘ − h 1 ‘ ) < − 180 °
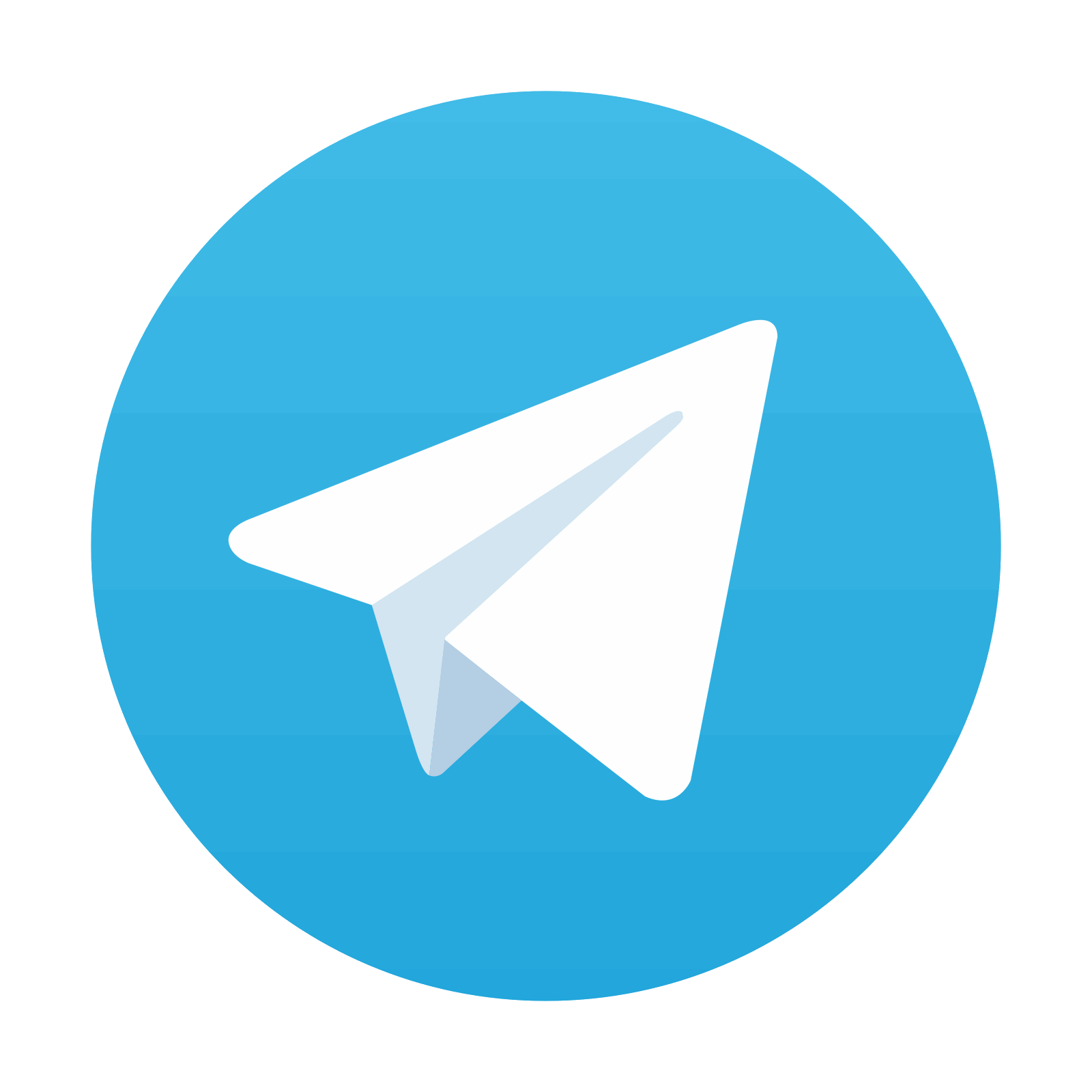
Stay updated, free dental videos. Join our Telegram channel

VIDEdental - Online dental courses
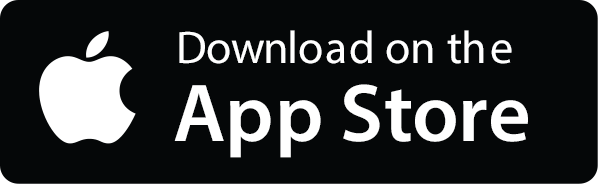
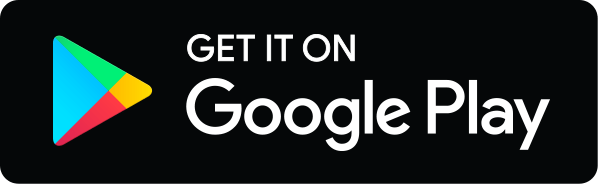