Abstract
Objectives
To investigate the influence of bonding resin thixotropy and viscosity on dentine tubule penetration, blister formation and consequently on dentine bond strength as a function of air-blowing pressure (air-bp) intensity.
Methods
Two HEMA-free, acetone-based, one-bottle self-etch adhesives with similar composition except disparate silica filler contents and different bonding resin viscosities were investigated. The high-filler-containing adhesive (G-Bond) featured a lower viscous bonding resin with inherent thixotropic resin (TR) properties compared to the low-filler-containing adhesive (iBond) exhibiting a higher viscous bonding resin with non-thixotropic resin (NTR) properties. Shear bond strength tests for each adhesive with low (1.5 bar; 0.15 MPa; n = 16) and high (3.0 bar; 0.30 MPa; n = 16) air-bp application were performed after specimen storage in distilled water (24 h; 37.0 ± 1.0 °C). Results were analysed using a Student’s t -test to identify statistically significant differences ( p < 0.05). Fracture surfaces of TR adhesive specimens were morphologically characterised by SEM.
Results
Statistically significant bond strength differences were obtained for the thixotropic resin adhesive (high-pressure: 24.6 MPa, low-pressure: 9.6 MPa). While high air-bp specimens provided SEM images revealing resin-plugged dentine tubules, resin tags and only marginally blister structures, low air-bp left copious droplets and open dentine tubules. In contrast, the non-thixotropic resin adhesive showed no significant bond strength differences (high-pressure: 9.3 MPa, low-pressure: 7.6 MPa).
Conclusions
A pressure-dependent distinct influence of bonding resin thixotropy and viscosity on dentine bond strength has been demonstrated. Stronger adhesion with high air-bp application is explained by improved resin fluidity and facilitated resin penetration into dentine tubules.
Clinical significance
Filler particles used in adhesive systems may induce thixotropic effects in bonding resin layers, accounting for improved free-flowing resin properties. In combination with high air-bp this effect allows an easy plugging of dentine tubules and elimination of blister structures, both resulting in superior dentine bond strength.
1
Introduction
The clinical success and longevity of adhesive restorations strongly depend on the reliability and performance of bonding agents used . As the application of etch-and-rinse adhesives involves several disadvantages summarized under the term “technique sensitivity” , the interest in using self-etch adhesives and particularly one-step bonding systems is growing. Nevertheless their clinical application is still troublesome as the solvents used in these systems simultaneously dissolve inherently immiscible hydrophilic and hydrophobic components . Hence, solvent evaporation in combination with air-drying plays a fundamental role in achieving durable adhesion to tooth structures .
Although several compressed air-based effects on dentine bond strength for one-bottle self-etch adhesives are discussed in the literature [air drying time , air drying pressure , air drying temperature , air drying distance and technique sensitivity ], according to our findings, no publication has yet described the influence on the thixotropy and viscosity of bonding resin layers left on dentine surfaces. This aspect is particularly interesting because some manufacturers claim the application of a strong (maximum) air-bp for their adhesive systems to achieve adequate bond strength to the tooth structure. Until now such recommendations are solely discussed in the literature with respect to complete solvent and water evaporation to avoid unwanted blister and droplet formation of the solvent residues inside the bonding resin layer, jeopardizing bond strength. This approach provided an explanation for improved adhesion values obtained with higher or longer air-bp application and, in particular, different results for low- and high-pressure application when using the same adhesive system . However, the influence of bonding resin viscosity was never discussed and no conclusive explanations were presented about which factors other than solvent properties influenced the facility and effectiveness of blister elimination and air bubble removal.
To the authors’ knowledge, no literature is available evaluating the viscosity influence of pure bonding resin layers left on tooth surfaces and the impact of their thixotropic properties on blister formation, dentine tubule penetration and consequently dentine bond strength. Therefore, the aim of this study was to investigate similar adhesive systems with different bonding resin viscosities under well-defined air-bp conditions using 1.5 bar [0.15 MPa] and 3.0 bar [0.30 MPa]. We hypothesize that bonding resin thixotropy and differences in viscosity influence dentine bond strength. The null hypotheses to be tested were twofold: (1) Bonding resin thixotropy and viscosity in combination with high air-bp application have no influence on dentine bond strength and (2) air-bp application on a thixotropic bonding resin has no influence on resin penetration into dentine tubules and dentine bond strength.
2
Materials and methods
Two HEMA-free (2-hydroxyethyl methacrylate) commercially available, one-bottle self-etch adhesives were selected [G-Bond (GC, Tokyo, Japan), iBond (Heraeus Kulzer, Hanau, Germany)]. Both adhesives contained a mixture of acetone and water as solvent components but showed a considerably different silica filler percentage ( Table 1 ), indicating differing bonding resin viscosities as well as thixotropic resin (TR) and non-thixotropic resin (NTR) properties. While G-Bond contains 4-MET in combination with a phosphoric acid ester as the adhesion promoting functional monomer, for iBond the corresponding anhydride (4-META) is quoted. However, organic anhydrides readily undergo hydrolysis in an aqueous solution to yield the corresponding acids . Therefore it can be assumed that iBond likewise contained 4-MET. Furthermore, it was previously reported that iBond features a no further specified amount of glutaraldehyde .
Adhesive # | Commercial product; Manufacturer | Composition* | Ash content (wt%) $ | FTIR peaks of ash content ‡ |
---|---|---|---|---|
NTR | iBond; Heraeus Kulzer; Hanau, Germany Lot: 010702 |
4-META, UDMA, acetone, water, photoinitiator | 0.9 ± 0.1 | 3436 cm −1 (m), 1630 cm −1 (w), fingerprint: 1115 cm −1 (s), 805 cm −1 (w), 476 cm −1 (m) |
TR | G-Bond; GC; Tokyo, Japan Lot: 1308221 |
4-MET, phosphoric acid ester monomer, UDMA, silica filler, acetone, water, photoinitiator | 10.7 ± 0.2 | 3430 cm −1 (m), 1631 cm −1 (w), fingerprint: 1118 cm −1 (s), 807 cm −1 (m), 729 cm −1 (w), 475 cm −1 (s) |
Pure bonding resins were isolated by means of dispensing 1.0 mL aliquots of each adhesive liquid on separate watch glasses and subsequent storing for 5 d in a dark and dry oven at 37 °C . The remaining homogeneous yellowish bonding resin residues were examined without further treatment (weight loss: < 0.02 wt%, 1 d, 37 °C). Special attention was paid at all times to protect the isolated resin samples from light irradiation. The filler content was confirmed by ashing in air ( Table 1 ). Peak assignment for the FTIR spectra of the isolated fillers (ash content) was in accordance with previously described results for silica except for one peak of the TR-filler at 729 cm −1 ( Table 1 ). This peak seemed to be filler specific or could have been generated during the ashing process. All investigations were carried out under ambient laboratory conditions (23 ± 1 °C; 50 ± 5% relative humidity).
2.1
Bonding resin viscosity
Viscosity properties of the bonding resin residues (TR, NTR) were investigated using a controlled stress rheometer ( HAAKE RheoStress 600 , Thermo-Fisher, Waltham, USA) at 23 ± 1 °C and 50 ± 5% relative humidity. A plate–plate geometry was selected (20.0 mm diameter, 0.10 mm distance) with shear rates ranging from 0.1 to 100 s −1 (t = 180 s). Up- (r up ) and down- (r down ) ramps were measured back-to-back for each resin residue (n = 3) .
The fluidity was assessed and visualised placing aliquot amounts of the TR and NTR samples on glass slides with equal altitude. The slides were arranged in an upright position. Low (1.5 bar [0.15 MPa]) and high (3.0 bar [0.30 MPa]) air-bp was applied on each resin bead at an exact distance of 10.0 mm (NTR: 10 s; TR: 5 s) to simulate the air-drying process (Section 2.2 .). Before and after each air-bp application photographs were taken.
2.2
Specimen preparation for shear bond strength test
Sixty-four bovine mandibular incisors extracted from two- to three-year-old cattle were used as a dentine-substitute for human teeth . All operations, including tooth conditioning, storage, embedding and bonding surface preparation (only coronal dentine), were performed in accordance with the ISO Technical Specification and were conducted at ambient laboratory conditions (23 ± 1 °C, 50 ± 5% relative humidity). Before adhesive application, the dentine surfaces of the mounted teeth were gently air-dried with oil-free compressed air, avoiding dehydration. All adhesive bottles were thoroughly shaken before use, and adhesives were applied according to manufacturer’s instructions as follows:
NTR adhesive was dispensed into a well and immediately applied using a micro brush to generate a saturated layer on the dentine surface, followed by gentle agitation for 20 s. Subsequently air-bp (1.5 bar [0.15 MPa] or 3.0 bar [0.30 MPa]), adjusted exactly using a pressure regulator (Minifix, Riegler & Co. KG, Bad Urach, Germany) and carefully monitored using a micro-processor-controlled digital manometer (LEO 2, Keller AG, Winterthur, Switzerland), was applied for 10 s while swaying specimens on a flat table plate at a fixed distance of exactly 10.0 mm. To guarantee a constant air-bp, the regulator inside the three-way-syringe handpiece was removed to achieve a maximum flow rate, which was equivalent to the value resulting from a fully pressed regulator button. Finally, curing of the resulting resin layer was performed for 20 s (Elipar ® Trilight, 3 M Deutschland GmbH, Seefeld, Germany; 800 mW/cm 2 ).
TR adhesive was similarly applied from a well using a disposable applicator to generate a uniform layer of adhesive liquid on the dentine surface. In contrast to the NTR adhesive, this layer was left alone for 10 s without further treatment. Subsequently, air-bp was applied in the same manner as described above for the NTR adhesive with the modification of a 5-s application time. In contrast to the NTR adhesive, curing of the resulting layer was performed for 15 s (Elipar ® Trilight).
Immediately after each adhesive polymerization, a cylinder (2.33 mm diameter) of light-curing core build-up composite (Clearfil Photo Core, Kuraray, Okayama, Japan, Lot 0175AA) was mounted using a bonding clamp and for each specimen a new bonding mould insert of the UltraTester™ system (Ultradent Inc., South Jordan, Utah). The composite was first polymerized on top for 40 s (Elipar ® Trilight) and after careful removal of the mould, four times on opposing sides for 30 s respectively. For each adhesive system and air-bp 16 specimens were prepared (in total n = 64) and stored in distilled water (24 h; 37.0 ± 1.0 °C) before shear bond strength testing.
2.3
Shear bond strength test
The dentine bond strength was determined using a shear bond test kit of the UltraTester™ system (Ultradent Inc., South Jordan, Utah) in combination with a Zwick 1454 universal testing device (Zwick/Roell, Ulm, Germany) at a crosshead speed of 1 mm/min. Shear load was applied until fracture occurred to calculate the shear bond strength values in MPa.
2.4
SEM analysis
For the TR adhesive and each air-bp, a morphological characterisation of the surface structure for both fracture pieces was performed using an Amray 1610 Turbo scanning electron microscope (KLA-Tencor, Milpitas, California, USA) at an accelerating voltage of 10 kV and a magnification of 1000× (dentine surface) and 1250× or 1260× (composite surface). Each piece was observed directly after the shear bond test, fixed to specimen holders (Plano, Wetzlar, Germany) and sputtered with gold. To expose resin tags for SEM analysis the rear side of the dentine fracture surfaces was immersed in 32% hydrochloric acid for 5 h to remove mineralized components followed by extensive rinsing with distilled water and subsequent air drying. All observations were conducted by one person.
2.5
Statistical analysis
Means and standard deviations of the shear bond strength values were calculated. All data were subjected to a Kolmogorov-Smirnov test to check for normal distribution and to a Levene test to check for homogeneity of variances. Because the test results revealed, for all groups, normal distributions and homogeneity of variances, parametric statistics (Student’s t -test for independent samples) were applied to identify significant differences between the air-bps for each self-etch adhesive system and between the same pressures for different adhesive systems. All statistical analyses were performed using SPSS for Windows (release 22.0.0.0, SPSS Inc., Chicago, IL, USA) at a significance level of p < 0.05.
2
Materials and methods
Two HEMA-free (2-hydroxyethyl methacrylate) commercially available, one-bottle self-etch adhesives were selected [G-Bond (GC, Tokyo, Japan), iBond (Heraeus Kulzer, Hanau, Germany)]. Both adhesives contained a mixture of acetone and water as solvent components but showed a considerably different silica filler percentage ( Table 1 ), indicating differing bonding resin viscosities as well as thixotropic resin (TR) and non-thixotropic resin (NTR) properties. While G-Bond contains 4-MET in combination with a phosphoric acid ester as the adhesion promoting functional monomer, for iBond the corresponding anhydride (4-META) is quoted. However, organic anhydrides readily undergo hydrolysis in an aqueous solution to yield the corresponding acids . Therefore it can be assumed that iBond likewise contained 4-MET. Furthermore, it was previously reported that iBond features a no further specified amount of glutaraldehyde .
Adhesive # | Commercial product; Manufacturer | Composition* | Ash content (wt%) $ | FTIR peaks of ash content ‡ |
---|---|---|---|---|
NTR | iBond; Heraeus Kulzer; Hanau, Germany Lot: 010702 |
4-META, UDMA, acetone, water, photoinitiator | 0.9 ± 0.1 | 3436 cm −1 (m), 1630 cm −1 (w), fingerprint: 1115 cm −1 (s), 805 cm −1 (w), 476 cm −1 (m) |
TR | G-Bond; GC; Tokyo, Japan Lot: 1308221 |
4-MET, phosphoric acid ester monomer, UDMA, silica filler, acetone, water, photoinitiator | 10.7 ± 0.2 | 3430 cm −1 (m), 1631 cm −1 (w), fingerprint: 1118 cm −1 (s), 807 cm −1 (m), 729 cm −1 (w), 475 cm −1 (s) |
Pure bonding resins were isolated by means of dispensing 1.0 mL aliquots of each adhesive liquid on separate watch glasses and subsequent storing for 5 d in a dark and dry oven at 37 °C . The remaining homogeneous yellowish bonding resin residues were examined without further treatment (weight loss: < 0.02 wt%, 1 d, 37 °C). Special attention was paid at all times to protect the isolated resin samples from light irradiation. The filler content was confirmed by ashing in air ( Table 1 ). Peak assignment for the FTIR spectra of the isolated fillers (ash content) was in accordance with previously described results for silica except for one peak of the TR-filler at 729 cm −1 ( Table 1 ). This peak seemed to be filler specific or could have been generated during the ashing process. All investigations were carried out under ambient laboratory conditions (23 ± 1 °C; 50 ± 5% relative humidity).
2.1
Bonding resin viscosity
Viscosity properties of the bonding resin residues (TR, NTR) were investigated using a controlled stress rheometer ( HAAKE RheoStress 600 , Thermo-Fisher, Waltham, USA) at 23 ± 1 °C and 50 ± 5% relative humidity. A plate–plate geometry was selected (20.0 mm diameter, 0.10 mm distance) with shear rates ranging from 0.1 to 100 s −1 (t = 180 s). Up- (r up ) and down- (r down ) ramps were measured back-to-back for each resin residue (n = 3) .
The fluidity was assessed and visualised placing aliquot amounts of the TR and NTR samples on glass slides with equal altitude. The slides were arranged in an upright position. Low (1.5 bar [0.15 MPa]) and high (3.0 bar [0.30 MPa]) air-bp was applied on each resin bead at an exact distance of 10.0 mm (NTR: 10 s; TR: 5 s) to simulate the air-drying process (Section 2.2 .). Before and after each air-bp application photographs were taken.
2.2
Specimen preparation for shear bond strength test
Sixty-four bovine mandibular incisors extracted from two- to three-year-old cattle were used as a dentine-substitute for human teeth . All operations, including tooth conditioning, storage, embedding and bonding surface preparation (only coronal dentine), were performed in accordance with the ISO Technical Specification and were conducted at ambient laboratory conditions (23 ± 1 °C, 50 ± 5% relative humidity). Before adhesive application, the dentine surfaces of the mounted teeth were gently air-dried with oil-free compressed air, avoiding dehydration. All adhesive bottles were thoroughly shaken before use, and adhesives were applied according to manufacturer’s instructions as follows:
NTR adhesive was dispensed into a well and immediately applied using a micro brush to generate a saturated layer on the dentine surface, followed by gentle agitation for 20 s. Subsequently air-bp (1.5 bar [0.15 MPa] or 3.0 bar [0.30 MPa]), adjusted exactly using a pressure regulator (Minifix, Riegler & Co. KG, Bad Urach, Germany) and carefully monitored using a micro-processor-controlled digital manometer (LEO 2, Keller AG, Winterthur, Switzerland), was applied for 10 s while swaying specimens on a flat table plate at a fixed distance of exactly 10.0 mm. To guarantee a constant air-bp, the regulator inside the three-way-syringe handpiece was removed to achieve a maximum flow rate, which was equivalent to the value resulting from a fully pressed regulator button. Finally, curing of the resulting resin layer was performed for 20 s (Elipar ® Trilight, 3 M Deutschland GmbH, Seefeld, Germany; 800 mW/cm 2 ).
TR adhesive was similarly applied from a well using a disposable applicator to generate a uniform layer of adhesive liquid on the dentine surface. In contrast to the NTR adhesive, this layer was left alone for 10 s without further treatment. Subsequently, air-bp was applied in the same manner as described above for the NTR adhesive with the modification of a 5-s application time. In contrast to the NTR adhesive, curing of the resulting layer was performed for 15 s (Elipar ® Trilight).
Immediately after each adhesive polymerization, a cylinder (2.33 mm diameter) of light-curing core build-up composite (Clearfil Photo Core, Kuraray, Okayama, Japan, Lot 0175AA) was mounted using a bonding clamp and for each specimen a new bonding mould insert of the UltraTester™ system (Ultradent Inc., South Jordan, Utah). The composite was first polymerized on top for 40 s (Elipar ® Trilight) and after careful removal of the mould, four times on opposing sides for 30 s respectively. For each adhesive system and air-bp 16 specimens were prepared (in total n = 64) and stored in distilled water (24 h; 37.0 ± 1.0 °C) before shear bond strength testing.
2.3
Shear bond strength test
The dentine bond strength was determined using a shear bond test kit of the UltraTester™ system (Ultradent Inc., South Jordan, Utah) in combination with a Zwick 1454 universal testing device (Zwick/Roell, Ulm, Germany) at a crosshead speed of 1 mm/min. Shear load was applied until fracture occurred to calculate the shear bond strength values in MPa.
2.4
SEM analysis
For the TR adhesive and each air-bp, a morphological characterisation of the surface structure for both fracture pieces was performed using an Amray 1610 Turbo scanning electron microscope (KLA-Tencor, Milpitas, California, USA) at an accelerating voltage of 10 kV and a magnification of 1000× (dentine surface) and 1250× or 1260× (composite surface). Each piece was observed directly after the shear bond test, fixed to specimen holders (Plano, Wetzlar, Germany) and sputtered with gold. To expose resin tags for SEM analysis the rear side of the dentine fracture surfaces was immersed in 32% hydrochloric acid for 5 h to remove mineralized components followed by extensive rinsing with distilled water and subsequent air drying. All observations were conducted by one person.
2.5
Statistical analysis
Means and standard deviations of the shear bond strength values were calculated. All data were subjected to a Kolmogorov-Smirnov test to check for normal distribution and to a Levene test to check for homogeneity of variances. Because the test results revealed, for all groups, normal distributions and homogeneity of variances, parametric statistics (Student’s t -test for independent samples) were applied to identify significant differences between the air-bps for each self-etch adhesive system and between the same pressures for different adhesive systems. All statistical analyses were performed using SPSS for Windows (release 22.0.0.0, SPSS Inc., Chicago, IL, USA) at a significance level of p < 0.05.
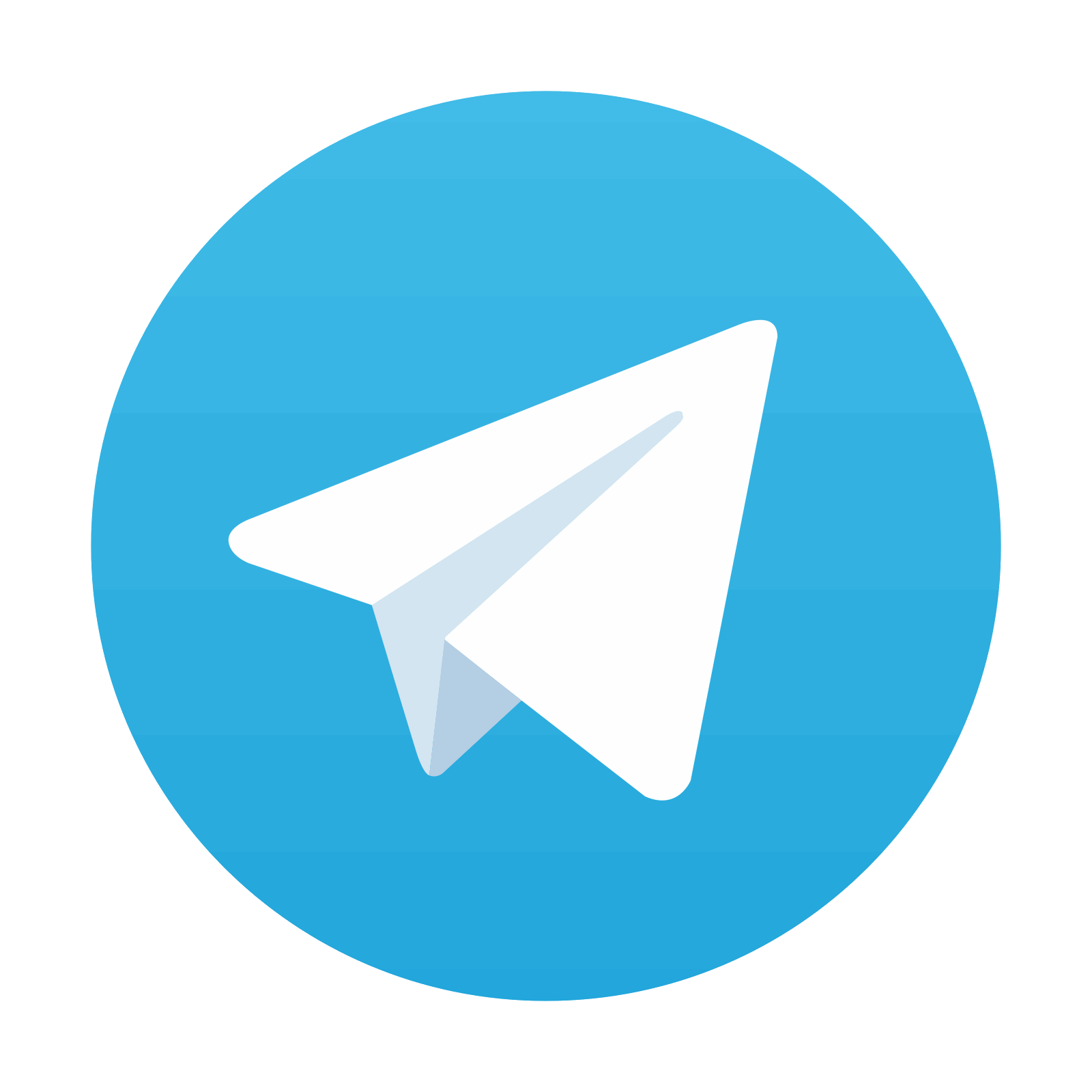
Stay updated, free dental videos. Join our Telegram channel

VIDEdental - Online dental courses
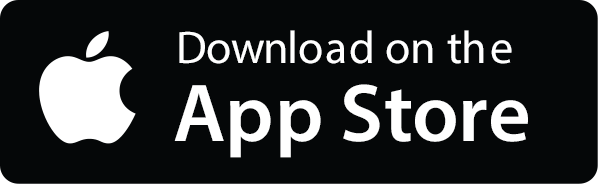
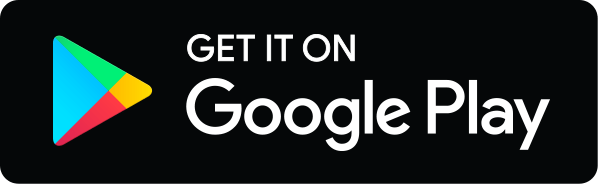