4
The Peripheral Nerve and Local Anesthesia
This chapter reviews the microanatomy of peripheral nerves, nerve cell membranes, neurophysiology, and the mechanism governing local anesthetic action.
Peripheral Nerve Microanatomy
Neurons
Nerve cells, called neurons, are the anatomic and functional units of the nervous system. While all human cells are characterized by a difference in potential (voltage) across their membranes, with a negatively charged interior and positively charged exterior near the surface, only muscle and nerve cells are excitable, meaning that they are able to alter that potential in response to a stimulus. Neurons, moreover, can convey electrical impulses along their membranes between the central nervous system (CNS) and the rest of the body, and vice versa. Neurons have three main constituents.
- The body, soma, neurocyton, or perikaryon (peri = around, karyon = nucleus), the bulbous part of the cell that contains the nucleus and subcellular organelles (endoplasmic reticulum [ER], Golgi apparatus, mitochondria, etc.), provides vital metabolic support for the entire neuron.
- The dendrites (dendron = tree branch) are thin cytoplasmic filaments projecting off the cell body. They receive electrochemical impulses that they propagate to the soma.
- The axon, a longer and thicker extension than the dendrites, carries electrochemical impulses from the soma to other organs and nerves.
Depending on function and morphology, neurons can be divided into three basic types: (i) motor or efferent (typically multipolar) neurons that conduct impulses from the Central Nervous System (CNS) to effectors (such as muscles); (ii) sensory or afferent (pseudo‐unipolar or unipolar) neurons, discussed below; and (iii) association or interneurons.
Sensory Neurons
These cells are also known as unipolar or pseudo‐unipolar neurons because the single axon (trunk or extension) that projects off the body subsequently divides into two. One part courses to the periphery, ending in this case in the gums, teeth, or bones, where sensory receptors capture stimuli for propagation to the soma, while the other carries stimuli to other CNS neurons. Although anatomically the former would be a dendrite and the latter an axon, as functionally they act as a single long axon, thus that is the term used for both (Figure 4.1).
The body of these neurons lies not in the CNS but in the dorsal root ganglion (spinal cord), while the maxillofacial sensory nerves lie in the semilunar or trigeminal (Gasserian) ganglion.
Axons
Axons are the trunks or axis cylinders that extend off the bodies of sensory nerves. They are sheathed by a series of cells known as Schwann cells. Although the functions of the latter are not fully understood, they are associated with the modulation of nervous conduction, the provision of direct metabolic support in the form of energy or protein synthesis, and the release of trophic factors to enhance axon function. Together the axon and its Schwann cells, called nerve fibers, constitute a functional element separated from the surrounding tissue by a basal lamina. Axons consist essentially of two parts.
- The axoplasm or neuron cytoplasm, like the cytoplasm in the soma, is a viscous liquid five times denser than water. It contains subcellular organelles such as mitochondria or the ER and provides the cell membrane with metabolic support (Meymaris 1975).
- The nerve membrane or axolemma or neurilemma that separates the axoplasm from extracellular liquids is the element around which this discussion revolves as it is where nervous excitation and electrochemical conduction take place. It is discussed in detail below.
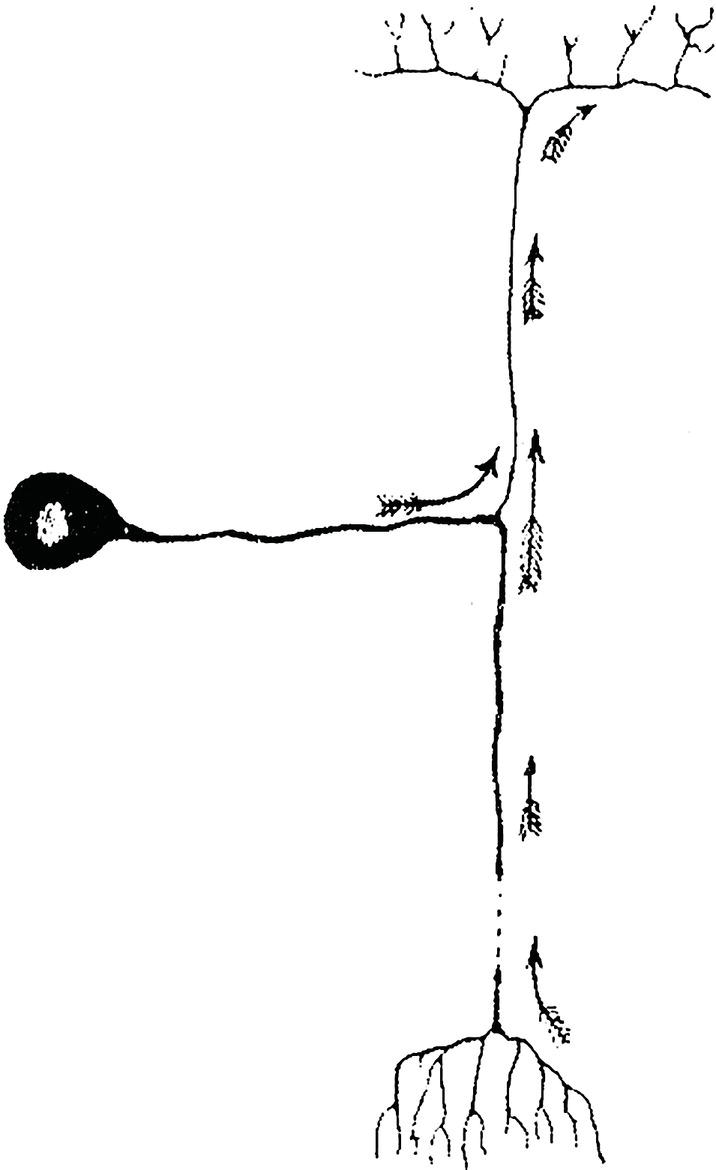
Figure 4.1 Sensory or unipolar neuron.
Source: Redrawn from Cajal (1899).
Membranes
The cell membrane is a semipermeable structure just 7–10 nanometers (nm) thick that separates the axoplasm from extracellular fluids. Although the molecular organization of the membrane is not understood in full detail, a number of important features have been described (Singer and Nicolson 1972; Meymaris 1975; Wildsmith 1986; Guyton and Hall 2007).
- It has a phospholipid bilayer (25%) in which each layer consists of (i) polar (hydrophilic) phosphate groups oriented toward the membrane surface, in other words, in the inner layer toward the axoplasm and in the outer toward the extracellular fluids, and (ii) apolar or nonpolar (hydrophobic) fatty acid groups in the center, oriented inward in both layers and attracted to one another. The hydrophobic part blocks the flow of water and hydrosoluble ions such as sodium (Na+) and potassium (K+) while allowing the flow of liposoluble compounds.
- Cholesterol and neutral lipids (13%) dissolved in the phospholipid bilayer.
- Proteins (55%) distributed along and across the membrane. There are two types: (i) integral or intrinsic proteins spanning the entire width of the membrane from the axoplasm to the extracellular fluids; (ii) peripheral or extrinsic proteins found only on either the inner or outer surface that do not penetrate the entire membrane. All these proteins have many functions, such as antigen or other markers, receptors (for hormones for instance), enzymatic control of reactions on the membrane surface or molecular transport across the membrane. The fourth is the type of protein of major interest here.
- Small amounts (3%) of carbohydrates on the outer surface only, bound to lipids (glycolipids) or proteins (glycoproteins).
As the membrane is not solid its constituent phospholipids and proteins can move laterally. The proteins, which float in a “sea” of phospholipids, are not uniformly distributed (Figure 4.2) but change constantly in keeping with the so‐called “fluid mosaic model of the plasma membrane” (Singer and Nicolson 1972). Some however, bound to the cytoskeletal proteins, help maintain the general shape of the cell.
Nerve Fibers and Myelin
Schwann cells may surround the axon in one of two ways, either forming myelin or not.
Myelinated Fibers
Myelinated fibers are enveloped in a layer of myelin, a substance comprising alternating concentric layers of lipids and proteins. These layers are actually the Schwann cell membrane that winds around the axon with scarcely any cytoplasm between layers (Figure 4.3).
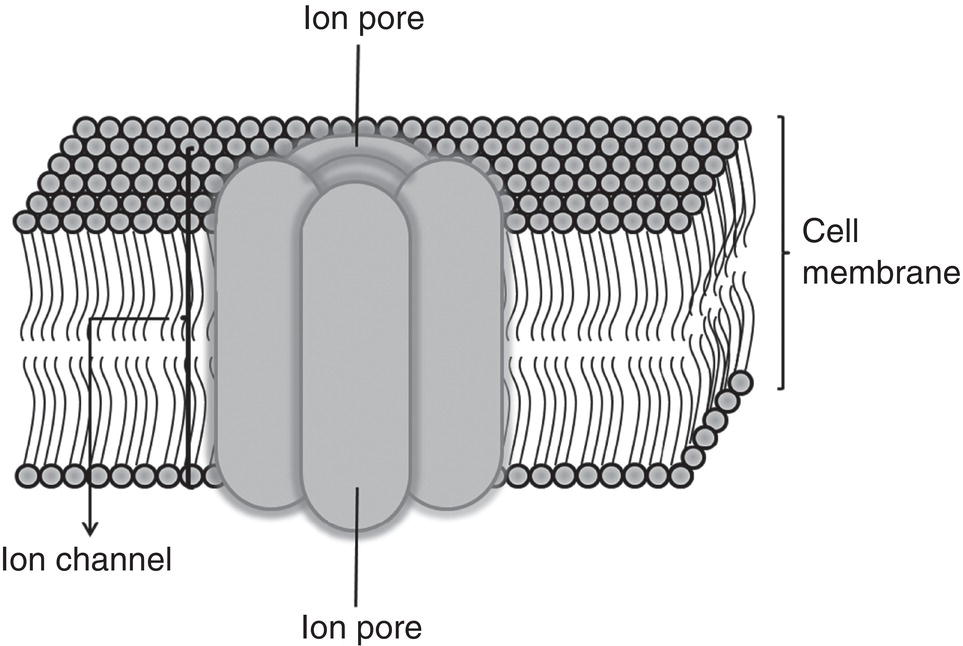
Figure 4.2 Diagram of cell membrane.
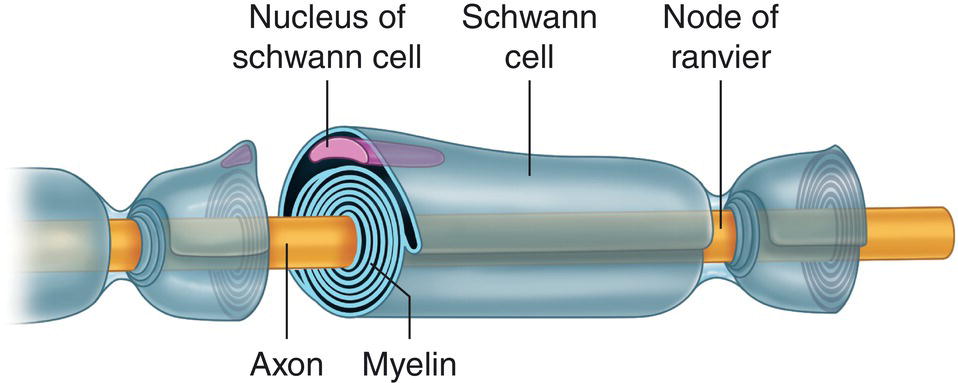
Figure 4.3 Myelinated fiber, Schwann cell, and nodes of Ranvier.
Source: Redrawn from De Jong (1977).
The most peripheral layer of the myelin sheath, the Schwann cell cytoplasm where the nucleus is located, is called the neurilemma or neurolemma (Jastak et al. 1995). At regular 0.2–0.3‐mm intervals in myelinated fibers, the neurilemmas of adjacent Schwann cells interconnect. As there is no myelin in this region the cover narrows, exposing the axon directly to extracellular fluids. Myelin affords powerful insulation that drastically reduces ion flow in the membrane by impeding contact between it and extracellular fluids because it leaves a tiny extracellular space, measuring barely 10–15 nm.
These gaps in the myelin layer, known as the nodes of Ranvier (Figure 4.3), while just 0.5–1.5 microns (μm) long, are extraordinarily important. It is here that ionic exchange takes place between the axoplasm close to the membrane and the extracellular fluids and where local anesthetics act.
The larger the diameter of the axon the thicker the myelin sheath and the greater the internodal distance (distance from one node of Ranvier to the next). Accordingly, the nodes of Ranvier are spaced at a fairly constant 100–200 times the axon diameter (Jastak et al. 1995). In myelinated fibers the nervous impulse jumps from node to node of Ranvier (Tasaki 1953) in “saltatory conduction”, accelerating propagation speed and requiring less ion movement. This arrangement is more efficient than conduction in unmyelinated axons because it calls for less energy and less metabolic activity.
The myelin sheath itself is interrupted at intervals by oblique incisions, Schmidt–Lantermann clefts, that connect it to the exterior and enhance elasticity by stretching the nerve (Heasman and Beynon 1987).
Unmyelinated Fibers
Unmyelinated fibers have an axon surrounded by the myelin‐free part of Schwann cells. In these fibers the Schwann cell membrane and cytoplasm normally sheathe several axons (Wildsmith 1986). Nerve impulse conduction in smaller diameter unmyelinated fibers is continuous, slower, and more energy‐intensive than in myelinated axons.
Nerve Fiber Classification
Table 4.1 lists the three main categories, A, B, and C, of peripheral nerve fibers and their subdivisions. Axon diameter, location, and function are also given in the table, along with the presence or otherwise of myelination.
Nerve impulse conduction depends primarily on two factors (Jack 1975): diameter, the greater the speedier, and the presence of myelin, which enhances speed. Myelinated A fibers, which are the thickest, exhibit the highest conduction speed. Conversely, C fibers are the thinnest and slowest. A delta (Aδ) and B fibers are often nearly undistinguishable and can only be differentiated on the grounds of electrophysiological properties not shown in the table (Jastak et al. 1995). The following are the fibers of greatest interest here.
- Myelinated Aδ fibers conduct first (sharp, immediate, and localized) pain that disappears with the pain stimulus.
- Type IV sensorial unmyelinated polymodal C fibers transmit second (diffuse or scantly localized, dull) pain that persists beyond the duration of the stimulus.
The first mandibular premolar dental pulp has been shown to have around 300–500 Aδ fibers and 1500–1800 polymodal C fibers (Johnsen et al. 1983). While the electrical pulp tester stimulates the former, it barely affects the latter (Lin and Chandler 2008; Abd‐Elmeguid and Yu 2009; Sampaio et al. 2012). Furthermore, polymodal C fibers seems more resistant to the effects to local anesthetics (Saha et al. 2016).
Peripheral Nerve Structure
Peripheral nerve trunks comprise hundreds or thousands of myelinated and unmyelinated nerve fibers, supported and protected by surrounding connective tissue (Figure 4.4) consisting of the following structures starting from the innermost layer (closest to the nerve) to the outermost layer (Sunderland 1965; Wildsmith 1986):
- The endoneurium, the innermost sheath of connective tissue, runs parallel to, bathes, and separates the nerve fibers, and its capillaries provide nutrients for the tissues (Wildsmith 1986). These blood vessels form the intrinsic system, are mainly nutritive, and have minimal adrenergic receptors (Myers and Heckman 1989).
- The perineurium is connective tissue surrounding the endoneurium that runs concentrically, obliquely, and parallel to nerve fibers, bundling from 500 to 1000 axons known as a fascicle. The perineurium carries blood vessels (vasa nervorum) which, in the form of a terminal capillary and precapillary network, penetrate the endoneurium. These blood vessels form the extrinsic system and are under adrenergic control (Myers and Heckman 1989). The innermost layer of the perineurium consists of a sort of membrane with several layers of cells known as the perilemma or perineural epithelium that hampers the diffusion of the anesthetic solution into nerve fascicles (Sunderland 1965; Shanthaveerappa and Bourne 1966).
- The epineurium, lax connective tissue containing some fatty cells, surrounds fascicles and holds them together. Its blood vessels form a vascular network (an extrinsic system) that responds to adrenergic stimulation and anastomose with intrinsic vessels (Myers and Heckman 1989). Its dense, thick outermost layer, the external epineurium, does not interfere with local anesthetic diffusion. The epineurium accounts for 30–75% of the total cross‐section of peripheral nerves (Sunderland 1965).
Table 4.1 Classification of peripheral nerve fibers.
Source: Data from Jastak et al. (1995).
Type of fiber | Myelin | Diameter (μm) | Speed (m/s) | Location | Function |
---|---|---|---|---|---|
Aα | +++ | ||||
Motor | 12–20 | 65–120 | Muscle | Motor | |
Ia sensorial | 13–22 | 70–130 | Muscle spindle | Proprioceptive | |
Ib sensorial | 12–120 | 65–120 | Tendon | Proprioceptive | |
Aβ | ++ | ||||
Motor | 7–14 | 40–80 | Muscle | Motor | |
II sensoriala | 5–15 | 20–80 | Tendon | Proprioceptive | |
Aγ | ++ | ||||
Motor | 2–10 | 10–50 | Muscle | Motor | |
II sensoriala | 5–15 | 20–80 | Tendon | Proprioceptive | |
Aδ | + | ||||
III sensorial | 1–7 | 5–40 | Various | First pain Temperature Pressure |
|
B | + | ||||
Preganglionic autonomic (sympathetic) | 1–5 | 4–25 | Heart Smooth muscle Gland |
Motor Motor Secretion |
|
C | − | ||||
Postganglionic autonomic (sympathetic) | 0.2–2 | 0.2–2 | Heart Smooth muscle Gland |
Motor Motor Secretion |
|
IV sensorial | 0.2–2 | 0.2–2 | Various | Second pain Temperature Pressure Visceral |
a Not distinguishable from one another.
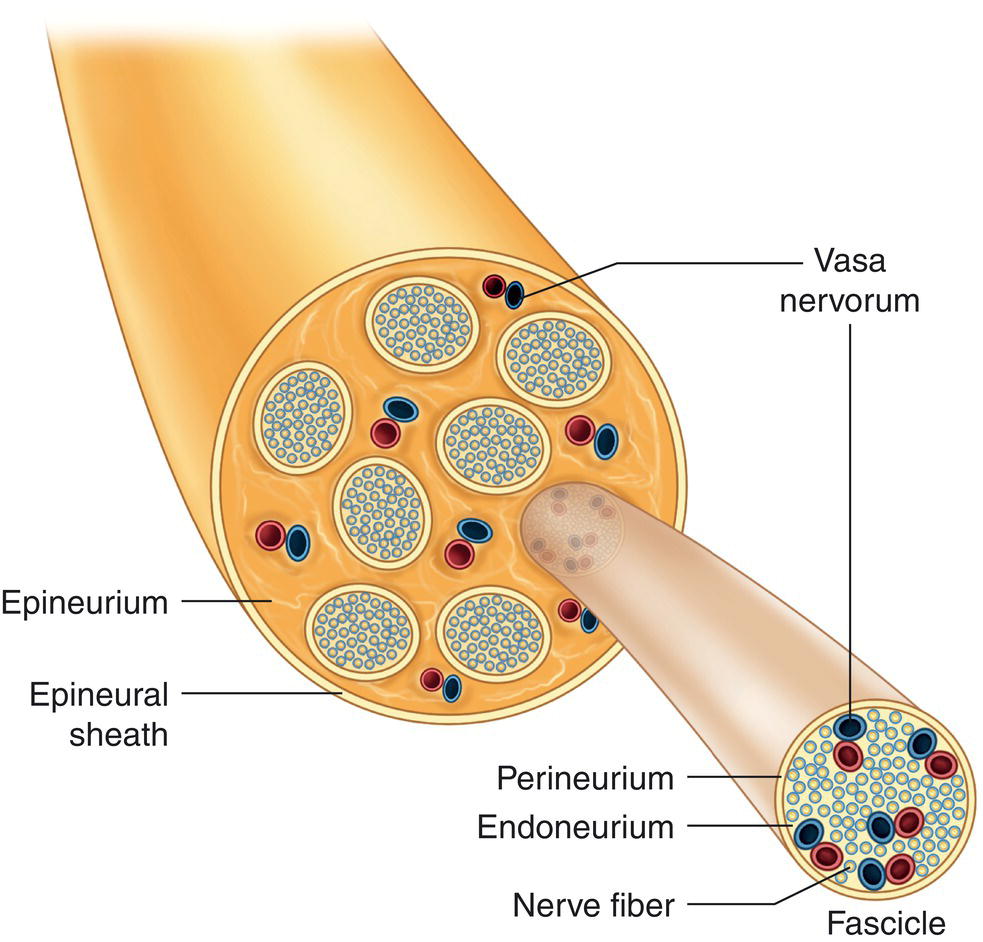
Figure 4.4 Peripheral nerve structure.
All these vasa nervorum absorb local anesthetic molecules, removing them from the nerve trunk. Lymphatic vessels also participate in this process, albeit minimally (<1%) (Asher 1892; Schou 1961).
Basic Membrane Proteins
Three membrane proteins are essential to conducting electrochemical impulses, the sodium–potassium pump, and the sodium and potassium channels. All three are integral membrane proteins (spanning the entire cell membrane) which, as their names infer, transport sodium (Na+) and potassium (K+) ions.
Sodium–Potassium Pump
The sodium–potassium pump (Na+/K+ pump) was discovered in 1957 by Skou, although there were precedents. Initially called the sodium pump (Skou 1957), it is an enzyme (sodium–potassium ATPase, Na+/K+ ATPase) essential to all animal cell membranes (Scheiner‐Bobis 2002). This bulbous integral protein transports Na+ and K+ ions and has three subunits (Scheiner‐Bobis 2002):
- The alfa (α) subunit, with a molecular mass of 100–113 kiloDaltons (kDa) and four isoforms (α1, α2, α3, and α4), is where adenosine triphosphate (ATP) bonds to the Na+ and K+ ions.
- The beta (β) subunit, with a molecular mass of 60 kDa and three isoforms (β1, β2, and β3), apparently stabilizes α.
- The gamma (γ) subunit, with a molecular mass of 7–11 kDa, is believed to modulate Na+/K+ pump activity, although its exact function is not known.
Na+/K+ATPase pumps three Na+ ions out and two K+ ions into the cell per ATP molecule hydrolyzed (Guyton 1987; Butterworth and Strichartz 1990; Scheiner‐Bobis 2002). As the enzyme pumps both ions against their respective electrochemical gradients, the process is active, i.e. it consumes energy. Interestingly, the Na+/K+ pump can increase its activity as circumstances require (Guyton and Hall 2007).
The function of the Na+/K+ pump is essential to the osmotic regulation of cell volume (Scheiner‐Bobis 2002), for the cell contains sizeable quantities of negatively charged organic proteins and other molecules too large to flow out of the cell. They consequently attract positively charged ions such as Na+, although as three Na+ ions are pumped out per every two K+ ions pumped in, inducing a net ionic loss, there is less water in the cell (Guyton and Hall 2007).
Another essential Na+/K+ pump function is its role in nerve impulse conduction, which is of particular interest in the present context, as discussed later.
Sodium Channels
Sodium channels (Na+ channels) are glycoproteins (Catterall 1984, 1988) with three subunits, α, β1, and β2 (Table 4.2). Their glycosylated groups are located on the outer surface of the cell membrane (Butterworth and Strichartz 1990). The structure–function relationship is still poorly understood (Ulbricht 2005). As these proteins are 11.8 nm in diameter (Catterall 1984), they span and project beyond the cell membrane, which is just 7–10 nm thick (Catterall 1984; Butterworth and Strichartz 1990).
The α subunit has four domains (D1–D4) with six membrane‐spanning helicoid α segments (S1–S6) (Ulbricht 2005). These segments are connected by intra‐ and extracellular loops (Catterall 2000; Ulbricht 2005) (Figure 4.5). The local anesthetic receptor is located in domain D4, segment 6, concurring with amino acids phenylalanine and tyrosine, the sites of which vary with sodium channel isoform or subtype. Segment S4 is positively charged and the pore lies between S5 and S6 (Ulbricht 2005). The Na+ ions, with a 0.095‐nm radius (Scheiner‐Bobis 2002; Lockless et al. 2007), or 0.19 nm when hydrated (Lipscombe 2005), flow through the pore, which on the outer end has a diameter of 0.3–0.5 nm (Catterall 1984, 1988; Fozzard et al. 2005).
Subunits β1 and β2 are long, glycosylated extracellular domains with folds reminiscent of those in immunoglobulins or myelin (Catterall 2000; Ulbricht 2005), a single transmembrane segment, and a small intracellular domain (Catterall 2000).
To date nine Na+ channel subtypes or isoforms have been identified, which differ in structure, expression pattern, biophysical properties, and location (Lai et al. 2004; Ulbricht 2005). Local anesthetics, which are scantly specific in this regard, block all nine (Lai et al. 2004; Fozzard et al. 2005). The subtypes of greatest relevance here are sited on the sensory neurons that propagate nociceptor (pain) signals, such as Nav 1.8, Nav 1.9 (Lai et al. 2004; Wells et al. 2007), and in the dental pulp, especially Nav 1.7 (Luo et al. 2008). Molecules may be developed in the future to act specifically on certain types of sodium channels, thereby reducing risks such as cardiotoxicity.
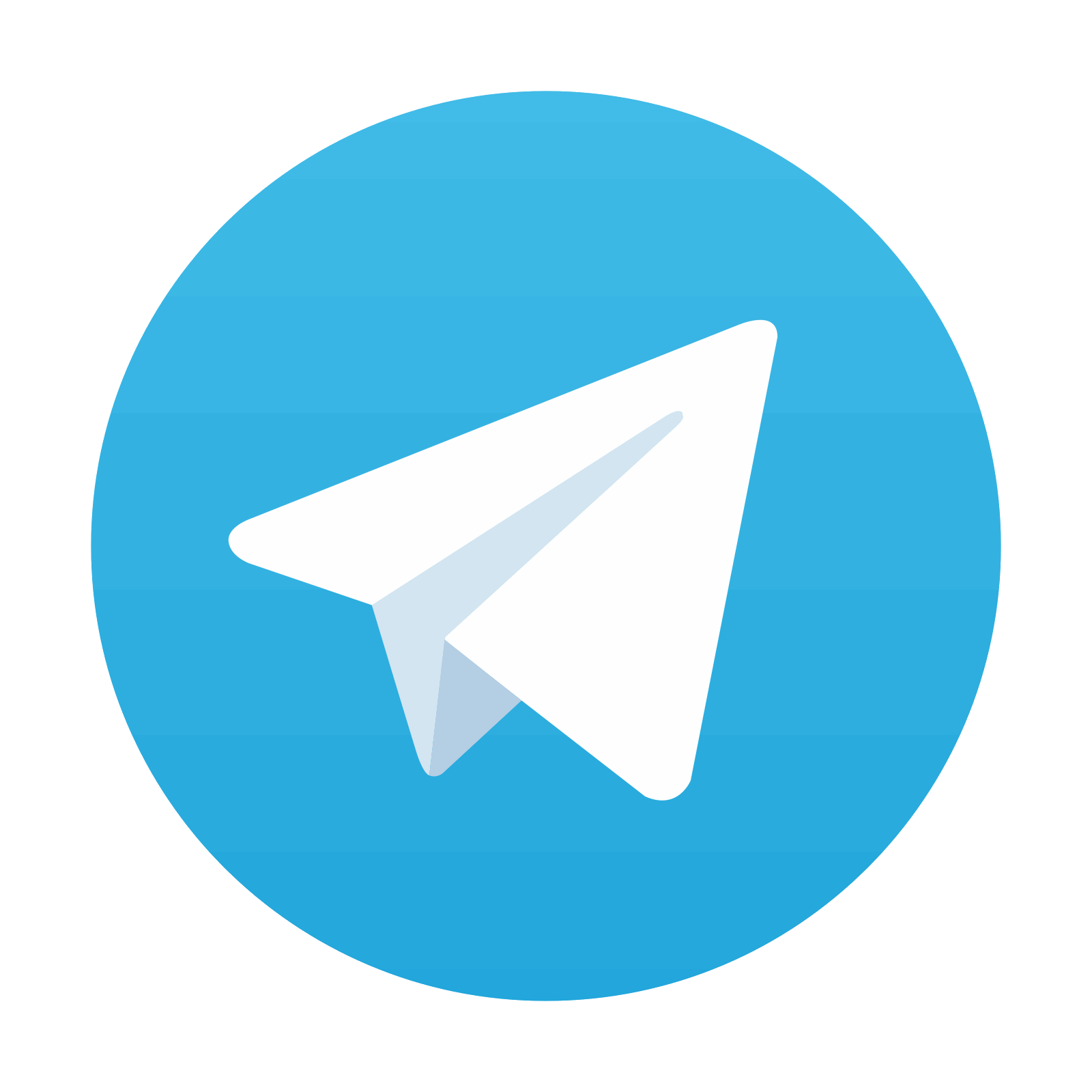
Stay updated, free dental videos. Join our Telegram channel

VIDEdental - Online dental courses
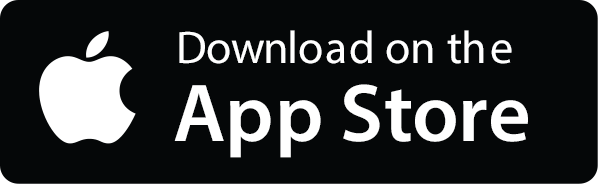
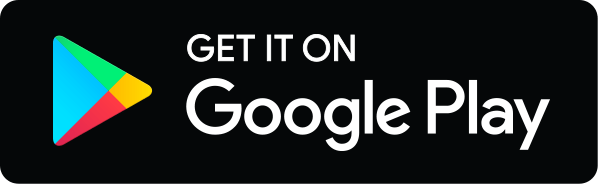