Abstract
Nicotine, one of the constituents of tobacco, is known to have an adverse effect on human health. We sought to clarify the interaction between nicotine and recombinant human bone morphogenetic protein 2 (rhBMP-2) in terms of osteogenesis in vitro and osteoinduction in vivo. Nicotine did not inhibit or stimulate alkaline phosphatase (ALP) activity or the amount of osteocalcin in C2C12 cells in the presence of rhBMP-2 in vitro. Ectopic bone formation using a collagen sponge containing rhBMP-2 was evaluated with and without nicotine after 21 days using radiographic, histological, biochemical, and immunohistochemical analyses. ALP activity in the medium-dose group (2.2 ± 0.9 IU/mg protein; P = 0.047) and the high-dose group (2.0 ± 0.1 IU/mg protein; P = 0.03) was significantly lower than in the control group. The calcium content in the medium-dose group (35.4 ± 12.9 μg/mg tissue; P = 0.0099) and high-dose group (34.8 ± 10.5 μg/mg tissue; P = 0.006) was significantly lower than in the control group. The number of vascular endothelial growth factor-positive cells in the high-dose group (671.9 ± 57.3 cells/mm 2 ; P = 0.03) was significantly lower than in the control group. Results showed that nicotine did not inhibit the stimulatory effect of rhBMP-2 in vitro, but a high dose of nicotine inhibited bone formation in vivo by adversely affecting vascularization.
Tobacco smoking is one of the major dangers to human health. It is associated with a large number of pathologies, such as cancer, cardiovascular diseases including atherosclerosis, myocardial infarct, and peripheral vascular disease, an increased risk of sudden death, and stroke. Moreover, tobacco smoking has been proposed as a risk factor for osteoporosis and delayed fracture repair. Besides nicotine, there are approximately 4000 other compounds in the particulate phase of tobacco smoke. The compounds affecting bone healing have not yet been accurately determined, but it is well known that nicotine is the most toxic constituent of cigarette smoke. A number of negative effects of nicotine on bone healing have been reported. Thus, nicotine has been claimed to negatively affect different stages of the bone-healing process, such as the inflammatory phase and blood flow, as well as the proliferative and mineralization phases. It has furthermore been suggested that nicotine in tobacco products causes peripheral vasoconstriction, decreased blood flow and oxygen tension, and tissue ischemia and a reduction in vascular ingrowth.
Recombinant human bone morphogenetic protein 2 (rhBMP-2) is one of the proteins that can trigger ectopic bone formation at non-skeletal sites in vivo and converts the differentiation pathway of the clonal myoblastic cell line C2C12 into that of osteoblast lineage cells in vitro. It has been indicated that the type I collagen atelocollagen is highly effective as a carrier for rhBMP-2. Recently, BMP studies have been reported from a translational point of view. Although some studies have indicated the adverse effect of nicotine on bone healing, others have reported no significant impact on bone healing. Zheng et al. showed that rhBMP-2 expression was usually maintained at a high level for two weeks after completion of distraction in the presence of nicotine, while Theiss et al. reported that nicotine exposure inhibited gene expression of BMPs in autogenous bone grafts in a rabbit posterolateral spine fusion model, a feature which contributes to disc degeneration. In this study, we sought to clarify the interaction between nicotine and rhBMP-2 in terms of osteogenesis in vitro and osteoinduction in vivo.
Materials and methods
In vitro experiments
Cytotoxicity evaluation assay (MTS test)
Cytotoxicity was evaluated using the Cell Titer 96 One Solution Cell Proliferation Assay Kit (Promega, Madison, WI, USA). The reaction is based on the bioreduction of the substrate 3-(4,5-dimethylthiazol-2-yl)-5-(3-carboxymethoxyphenyl)-2-(4-sulfophenyl)-2H-tetrazolium (MTS) into a brown formazan product by mitochondrial dehydrogenases of viable cells. C2C12 cells (American Type Culture Collection) were seeded at 5 × 10 2 cells per well in a 96-well plate containing Dulbecco’s modified Eagle’s medium (DMEM), 10% fetal bovine serum (FBS), and 1% penicillin. After 24 h, the cells were exposed to serial concentrations of nicotine ((−)-nicotine (1-methyl-2-(3-pyridyl)pyrrolidine); Wako Pure Chemical Industries, Ltd., Osaka, Japan) at medium concentrations of 0, 0.01, 0.1, 1, and 10 μM. Thirty-six hours after addition, 20 μl of the reagent was pipetted into each well of the 96-well assay plate containing the samples, in 100 μl of culture medium. The plate was incubated for 1 h at 37 °C in a humidified, 5% CO 2 atmosphere and the absorbance was recorded at 490 nm using a 96-well plate reader.
Alkaline phosphatase (ALP) activity and osteocalcin
C2C12 cells were seeded at 1 × 10 3 cells per well in six-well plates. Medium consisting of DMEM, 10% FBS, and 1% penicillin was added. After 24 h, the cells were exposed to serial concentrations of nicotine at medium concentrations of 0, 0.01, 0.1, 1, and 10 μM in addition to 200 ng/well rhBMP-2. The medium was changed every 2 days. On day 5, the medium was removed and the cells were washed with phosphate-buffered saline (PBS) and lysed in homogenizing buffer (0.15 M NaCl, 0.003 M NaHCO 3 , 0.1% Triton X, pH 7.4). The ALP activity and total protein were determined by 4-nitrophenylphosphate method and using the BCA protein assay kit (Micro BCA Protein Assay Reagent Kit; Pierce Biotechnology, Rockford, IL, USA), respectively, while the amount of osteocalcin was determined using an osteocalcin assay kit (Biomedical Technologies Inc., Stoughton, MA, USA).
von Kossa staining
On day 5, the medium was removed and the cells were washed with PBS and fixed in 4% paraformaldehyde (PFA) for 10 min, and then rinsed with distilled water. Five percent silver nitrate solution was added and the plate was exposed to UV light for 3 min, after which it was washed well with distilled water. Five percent sodium thiosulfate was added for 5 min and the plates were then rinsed in running tap water for 5 min.
Animal experiment
Thirty-two rats (Jc1-Wistar male, 10 weeks old, weight 250–280 g; obtained from CLEA JAPAN Inc., Tokyo, Japan) were randomly divided into four groups (eight rats per group). Saline was administered to the control group, while nicotine was administered thorough intraperitoneal injection at a rate of 0.1 mg/kg/day, 1.0 mg/kg/day, or 10.0 mg/kg/day for 21 days in the other three groups. rhBMP-2 (5 μg) was mixed with 3 mg of atelopeptide type I collagen (pH 3.0) (Cellmatrix LA; Nitta Gelatin Inc., Osaka, Japan), then lyophilized (EYELA FDU-2200; Tokyo Rikakikai Co., Ltd., Tokyo, Japan) and compressed in a syringe to form discs (4 mm in diameter and 1.5 mm thick). Rats were anesthetized by intraperitoneal injection of sodium pentobarbital (5.0 mg per 100 g of body weight). An osmotic mini-pump (200 μl) (Alzet Model 2004; Alza Scientific Products, Palo Alto, CA, USA) was implanted subcutaneously in the abdominal region, while the collagen discs with rhBMP-2 were inserted into the right calf muscle of each rat. On day 21, the rats were sacrificed with an overdose of sodium pentobarbital. All procedures were approved by the university animal care committee.
Body weight measurement
Body weights were measured on a daily basis until sacrifice.
Radiographic evaluation
In order to determine the radiopacity in the target muscle induced by the collagen implant with rhBMP-2, the collagen implant with the surrounding tissue was excised and examined with a soft X-ray radiograph for each implant (Sofron SRO-M50; Sofron, Tokyo, Japan).
Histological examination
The collagen implant was fixed in 10% neutral buffered formalin (pH 7.4), demineralized in ethylenediaminetetraacetic acid, and embedded in paraffin. Sections of 4 μm in thickness were cut and stained with hematoxylin and eosin.
Immunolocalization of vascular endothelial growth factor (VEGF)
The sections were stained using the labeled streptavidin biotinylated antibody method. Before the primary antibody was added, antigenic sites were irradiated with microwaves (20 min, 500 W, 90 °C). The slides were immersed in 3% hydrogen peroxide solution for 15 min to inhibit endogenous peroxidase before incubating for 5 min in Tris buffered saline T (TBST; 50 mM Tris–HCl, pH 7.6, 0.15 M NaCl + 0.05% Tween). To detect VEGF immunoexpression, slides were then exposed to rabbit polyclonal anti-VEGF antibody (NeoMakers, Fremont, CA, USA) and diluted 1:100 in PBS at 4 °C overnight.
Secondary biotinylated goat anti-rabbit immunoglobulin G was diluted 1:300 in PBS for 30 min at room temperature. After washing in TBST, the slides were exposed to peroxidase-conjugated streptavidin (Dako North America, Inc., Carpinteria, CA, USA) for 30 min at room temperature. After developing in 0.05% 3-amino-9-ethylcarbazole (AEC Substrate–Chromogen System; Dako North America Inc.), which gave a distinctive red color, the sections were counterstained with Mayer hematoxylin, cleared, and mounted with a cover slip.
Detection of apoptosis
Apoptosis was detected by the terminal deoxynucleotidyl transferase-mediated dUTP nick end-labeling (TUNEL) method using the Apoptag Plus Peroxidase In situ Apoptosis Detection Kit (Intergen Company, Purchase, NY, USA), in accordance with the manufacturer’s specifications.
Biochemical examination
Samples were weighed and homogenized in 0.25 M sucrose in a Polytron homogenizer (Bio-Mixer, type ABM; Nissei Inc., Osaka, Japan). The sediment was demineralized in 0.5 N HCl and the calcium content was determined by orthocresol–phthalein complex method. ALP activity was determined using the 4-nitrophenylphosphate method and the total protein was determined by BCA assay. The calcium content and the ALP activity (IU/mg protein) were used as indices of bone formation.
Statistical analysis
Data are expressed as the mean and standard deviation (SD). The statistical analysis was performed by analysis of variance (ANOVA) with Fisher’s least significant difference method when appropriate. The median and range of the values were given for each group. The results were verified using the two-tailed t -test (with 95% confidence intervals). Differences were considered significant if the probability was <0.05 or <0.01.
Results
In vitro results
Cytotoxicity of nicotine
The addition of nicotine did not inhibit cellular proliferation at any concentration. There was no difference among groups, including the control ( Fig. 1 A ).

ALP and osteocalcin activity
There was also no significant difference in ALP activity or the amount of osteocalcin among groups ( Fig. 1 B and C). These results indicate that nicotine had no effect on the osteogenic differentiation of C2C12 cells by rhBMP-2.
von Kossa staining
To evaluate the osteogenic differentiation of C2C12 cells by rhBMP-2, the calcium deposits were stained by the von Kossa method in cultured cells after 5 days ( Fig. 1 D–I). There were no significant differences in the amounts of calcium deposits.
In vivo results
Rat body weight
As shown in Fig. 2 , body weight measured on day 21 was significantly greater than that measured on day 1 in all groups, including those treated with a high dose of nicotine. There was no significant difference in body weight among groups.
Radiographic findings
After 21 days, radiographs revealed the presence of opaque regions in the calf muscle of every rat. The regions exhibiting radio-opacity were larger in the control group and the 0.1 mg/kg/day group (low-dose group), with no difference between them. For the 1.0 mg/kg/day group (medium-dose) and 10.0 mg/kg/day group (high-dose), the areas exhibiting opacities were smaller in comparison to those of the control and low-dose groups ( Fig. 3 A–D ).
ALP and calcium content
The ALP activity and calcium content of each group were measured on day 21 after implantation. ALP activity in the medium-dose group (2.2 ± 0.9 IU/mg protein; P = 0.047) and high-dose group (2.0 ± 0.1 IU/mg protein; P = 0.03) was significantly lower than in the control group (3.5 ± 1.3 IU/mg protein), whereas the difference was not significant for the low-dose group (2.78 ± 1.26 IU/mg protein; P = 0.19) ( Fig. 3 E). The calcium content in the medium-dose group (35.4 ± 12.9 μg/mg tissue; P = 0.0099) and high-dose group (34.8 ± 10.5 μg/mg tissue; P = 0.006) was significantly lower than in the control group (58.0 ± 12.1 μg/mg tissue), whereas the difference was not significant for the low-dose group (37.2 ± 13.6 μg/mg tissue; P = 0.11) ( Fig. 3 F). These results indicate that nicotine at high and medium doses inhibited bone formation in comparison with that in the control group.
Histological findings
The muscle bundles near the implant were edematous with the presence of large and rounded nuclei cells for the groups with medium- and high-dose nicotine (1.0 mg/kg/day, 10.0 mg/kg/day), indicating ischemia and decreased oxygen tension in the implant. In the control group, much new bone tissue was noted in the entire circumference around the implant margin, and bone formation was also noted in a region near the center of the implant ( Fig. 4 A, C, E, G ). Furthermore, trabecular bone was found in parts of the marginal area of implanted materials for the groups with medium- and high-dose nicotine in von Kossa sections, whereas bone formation of the entire area was observed in the control and low-dose groups ( Fig. 5 A–H ).
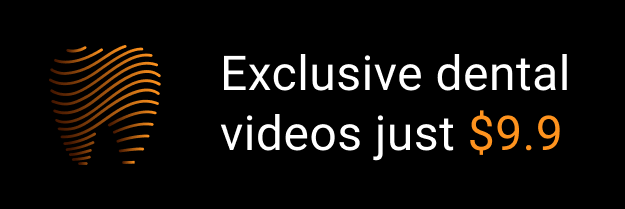