Abstract
The use of a laser in surgical procedures involving the soft tissues is advantageous due to its sterile and hemostatic nature. Several different lasers are in use for intraoral soft tissue surgery; however, small, efficient, and fibre-coupled lasers are favoured due to the tightly confined nature of the intraoral environment. This study proposes the use of a 1940-nm thulium fibre laser (Tm:fibre laser) for intraoral soft tissue procedures. Its thermal effects when used to make incisions were investigated. This laser was chosen due to its output wavelength, which is absorbed well by water in biological tissues. Lamb tongues were used in the experiments. The laser was coupled to a 600-μm silica fibre and incisions were made in contact mode with a continuous wave. The extent of ablation and coagulation produced were measured at three different speeds, powers, and numbers of passes. The thermal effects of laser power, movement speed, and number of passes on incision depth and ablation efficiency were determined. The Tm:fibre laser is a promising tool for intraoral surgery, with excellent absorption by tissue, good coagulative qualities, and easy to manipulate fibre output. Its use as an incisional tool with very little to no carbonization is shown in this study.
Intraoral soft tissue procedures such as frenectomy, vestibuloplasty, excisional and incisional biopsy, and leukoplakia removal are conventionally carried out using scalpels and scissors, with hemostats and sutures required to provide hemostasis and wound healing. Electrosurgery units allow bloodless surgery due to their ability to coagulate while cutting and may be preferred for such operations. However, the safety risks involved (possible electrical shocks, burns, and interference with pacemakers), the unpleasant odour and taste produced, and the possible artefacts at margins of a biopsy specimen must be taken into consideration.
The disadvantages of electrosurgery techniques have been overcome with the introduction of laser technology to intraoral soft tissue surgery. Lasers can be used to produce immediate and precise tissue destruction in a bloodless manner, forming an incision or ablation with minimal thermal damage to the surrounding healthy tissue. This property allows lasers to be used with ease in the surgical treatment of vascular lesions, while rendering pharmacological therapy unnecessary for patients with coagulation alterations. The incisions are self-sterilizing and are formed with minimal tissue distortion. The wounds formed afterwards heal by secondary intention, requiring no dressing or suturing. The sealing ability of the laser provides a bloodless dissection. Small blood vessels (up to 0.5 mm in diameter), lymphatic vessels, and nerve endings are sealed by the beam, which provides hemostasis, reduces the spread of tumour cells via lymphatics, and contributes to the lack of intraoperative and postoperative pain. Furthermore, although thermal damage is present at the margins of the excision, the histological alterations do not interfere with the diagnosis provided that the biopsy incision is larger than a traditional scalpel incision.
A photothermal interaction occurs when laser energy is absorbed by tissue and converted to heat, and this is the source of the cutting and coagulating ability of the laser. The extent of absorption is governed by the chromophores present in the tissue (such as water, haemoglobin, and melanin), is strongly related to the laser wavelength, and is one of the parameters that determine the heat generated in tissue. Vaporization of tissue (ablation) can be achieved when the heat generated inside reaches 100 °C, at which point the water molecules begin to vaporize.
Surrounding the ablation crater, an area of coagulation forms due to heat transport, which is responsible for sealing the blood vessels. This area of thermal damage is mostly unavoidable, but can be restricted by choosing appropriate laser parameters.
One means of minimizing the collateral thermal damage is choosing an appropriate laser type for the target tissue. Greater absorption of the laser energy will result in quicker ablation and less heat transport, which, in turn, will constrict the coagulation zone. The most abundant chromophores in soft tissues are water and haemoglobin; therefore wavelengths that are absorbed the most by these, will result in more precise cuts with less thermal damage. Haemoglobin has absorption peaks that mostly fall into the visible portion of the spectrum, whereas the absorption peaks of water are higher in the infrared region. In particular, above 1400 nm, light scattering in tissue becomes insignificant compared to absorption, and water molecules become the dominant absorbers due to the absorption coefficient increase of several orders of magnitude. Intraoral soft tissues are rich in water content and therefore can easily be cut using a laser that has a wavelength close to an absorption peak.
Of the many different laser types that are currently used for intraoral surgery, CO 2 (10,600 nm), Er:YAG (erbium-doped yttrium aluminium garnet laser; 2940 nm), and Er,Cr:YSGG (erbium, chromium-doped yttrium, scandium, gallium, and garnet laser; 2780 nm) are of great interest due to their emission wavelengths being close to water absorption peaks. Light is rapidly converted to heat at these wavelengths, creating a very steep temperature gradient, which gives these lasers the ability to ablate precisely in exchange for good coagulation properties. However, these wavelengths cannot be transmitted through silica fibres. CO 2 lasers utilize articulated arms or special hollow waveguides, whereas Er:YAG requires fluoride glass or sapphire fibres, which have higher loss and larger refractive indices compared to silica fibers.
The thulium fibre laser (Tm:fibre laser), with a 1940-nm emission wavelength that overlaps with one of the water absorption peaks, is a promising tool for intraoral surgery. The high absorption coefficient provides shallow penetration depth, enabling precise ablation with less thermal damage. Furthermore, the output can be coupled to silica fibres to provide easier manipulation and access to hard-to-reach zones in the mouth. The laser used in the present study is shown in Fig. 1 .

The aim of this study was to investigate the efficiency of the Tm:fibre laser as an incisional tool for intraoral soft tissue surgery. For this purpose, three variables were taken into consideration during the forming of the incisions – power, speed, and number of passes – and the ablation efficiencies for each set of variables were calculated.
Materials and methods
Preparation of samples
A total of 54 lamb tongues were used as the target tissue and assumed as test subjects simulating the soft tissue. The tongues obtained were cooled to 4 °C after sacrifice of the animal and were used within 24 h. Samples were washed with and immersed in saline to prevent dehydration of the tissue and to simulate the damp nature of the intraoral environment. Four laser applications were performed per tongue, side by side, allowing enough space (8–10 mm) between incisions to eliminate the effects of one incision on another. Two hundred sixteen incisions were performed in total in order to study 27 different parameter combinations, with each parameter set comprising eight different incisions.
Laser system
The thulium fibre laser (TLR-5-1940; IPG Laser GmbH, Germany) used has an emission wavelength of 1940 nm and a maximum power of 5 W. The output power and exposure time of the laser were controlled by a custom-built controller unit (Teknofil Inc., Istanbul, Turkey), which could be accessed via a LabVIEW interface to set the parameters. The output was provided through a fibre optic cable, which was coupled to a 600 μm fibre via focusing lenses and xyz alignment apparatus. The output of the fibre was checked with an optical power metre (1918-C; Newport Co., CA, USA) before every application.
Laser application procedure
The fibre was applied in contact with the dorsal surface of the tongue at an angle of 30° to form the incisions, starting at the middle and moving towards the apex. The tip of the fibre was checked for irregularities and cleaved if needed before every application. The variables studied were power (2.5 W, 3 W, and 3.5 W), speed of the fibre tip (0.5, 0.75, and 1 mm/s), and number of passes (single, three, and five). The fibre tip was moved manually to simulate use in the clinic, at a constant speed through a predetermined length to adjust the speed. The lengths of incisions were set to 1, 1.5, or 2 cm while the duration was set to 20 s.
Slide preparation and measurements
The incisions were excised in rectangular blocks that included some of the unaltered surrounding tissue and these were fixed in 10% buffered formalin for 4 days. The samples were embedded in paraffin blocks at the end of the fixation phase, and 10-μm sections were obtained using a microtome (RM 2255; Leica Biosystems Nussloch GmbH, Germany). The sections obtained were then stained with haematoxylin and eosin (H&E) to enable visualization of the ablation and coagulation zones via light microscope (Eclipse 80i; Nikon Co., Tokyo, Japan). Measurements of the ablation depth and coagulation width at the surface (total thermally altered area), as well as the ablated and total thermally altered areas, were performed using the imaging software NIS Elements-D (Nikon Co, Tokyo, Japan). The ablation efficiency, an effective concept that has been defined previously by the authors and is the ratio of the ablation area to the total thermally altered area, was calculated using the measurements.
Data analysis
Measurements were obtained from eight samples for each set of parameters. Three-way analysis of variance (ANOVA) was used to determine whether or not each variable had an effect on ablation efficiency. The Tukey test was then used in order to determine the statistical difference between ablation efficiencies; the significance level was set to p < 0.05.
Results
The three-way ANOVA results revealed that the effect of each variable on the ablation efficiency was significant and therefore the efficiency depended on all three variables.
The ablation efficiency, calculated as the ratio of the area of the ablation crater to the area of total thermal damage, reached a maximum of 20.97% observed at 0.75 mm/s, 3.5 W power, and with five passes. However, on the whole, higher ablation efficiencies were achieved at the lowest speed (0.5 mm/s).
The mean ablation depths and coagulation widths measured for 0.5 mm/s speed are shown in Fig. 2 , along with the ablation efficiency which is plotted on the same graph. The significant differences in ablation depth, coagulation width, and ablation efficiency at constant power with increasing numbers of passes are shown in Table 1 . When the ablation depths were examined in relation to the number of passes, only the three passes group showed a significant increase in depth with increasing power, whereas in the single pass and five passes groups, only 3.5 W resulted in a significantly deeper ablation. On the other hand, coagulation depth in relation to the number of passes variable revealed that there was no significant difference in width with increasing power in the single pass group. In the three passes group, 3.5 W power resulted in a significantly larger coagulation zone, while 3 W yielded a narrower coagulation zone in the five passes group.
Power | Ablation depth | Coagulation width | Ablation efficiency | ||||||
---|---|---|---|---|---|---|---|---|---|
1p–3p | 1p–5p | 3p–5p | 1p–3p | 1p–5p | 3p–5p | 1p–3p | 1p–5p | 3p–5p | |
2.5 W | * | * | * | * | * | ||||
3 W | * | * | * | ||||||
3.5 W | * | * | * | * | * | * |
* Significantly different groups are denoted with an asterisk.
Ablation efficiency for each incision was calculated as the ratio of the ablated area to the total thermally altered area. The efficiencies calculated for 0.5 mm/s did not show a significant difference with increasing power in the single and five passes groups when this was organized with respect to the number of passes. However, in the three passes group, 3.5 W power yielded higher ablation efficiency.
Figure 3 shows the ablation depth and coagulation width data measured for 0.75 mm/s as well as the calculated ablation efficiency for that speed. The significant differences in ablation depth, coagulation width, and ablation efficiency at constant power with increasing numbers of passes are shown in Table 2 . It was observed that ablation depth increased significantly with increasing power in the three and five passes groups, whereas in the single pass group, 3 W did not yield a significantly different ablation depth to either 2.5 W or 3.5 W, although the mean ablation depths increased with increasing power.
Power | Ablation depth | Coagulation width | Ablation efficiency | ||||||
---|---|---|---|---|---|---|---|---|---|
1p–3p | 1p–5p | 3p–5p | 1p–3p | 1p–5p | 3p–5p | 1p–3p | 1p–5p | 3p–5p | |
2.5 W | * | * | * | * | * | ||||
3 W | * | * | * | * | * | * | |||
3.5 W | * | * | * | * | * |
* Significantly different groups are denoted with an asterisk.
Coagulation width data, when considered in groups of the same number of passes, showed the only significant difference at 3.5 W power (wider than 2.5 W and 3 W) in the single pass group.
For the three passes group, no significant difference was observed among the different power settings used. The coagulation width obtained with five passes at 3 W was significantly larger than that obtained at both 2.5 W and 3.5 W, whereas there was no significant difference between 2.5 W and 3.5 W at that setting.
Ablation efficiency in relation to the number of passes showed no significant difference between power settings 2.5 W and 3 W, while 3.5 W yielded a significantly higher ablation efficiency compared to the other power settings.
Ablation depth and coagulation width data for the last utilized velocity, 1 mm/s, are shown in Fig. 4 , in which ablation efficiency is also plotted. The significant differences in ablation depth, coagulation width, and ablation efficiency at constant power with increasing numbers of passes are summarized in Table 3 . Sorting the ablation depths according to the number of passes, depth produced with 2.5 W was found to be significantly shallower than that at 3 W and 3.5 W in the three and five passes groups. In the single pass group, although the depth increased with increasing power setting used, the difference between 2.5 W and 3 W was not significant.
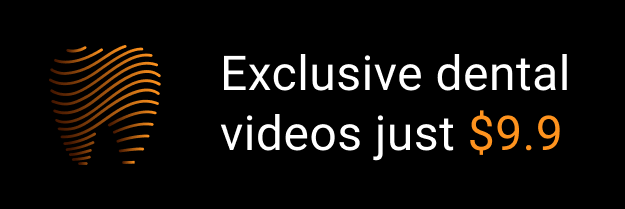