Abstract
Objective
This study evaluated the effects of different NaOCl concentrations and contact times on removal of the organic phase from mineralized dentin with and without the adjunctive use of EDTA, and the effect of NaOCl concentrations on canal wall erosion after the use of EDTA as the final active irrigant.
Methods
Dentin powders were immersed in 5.25% or 1.3% NaOCl for different contact periods and then rinsed with 17% EDTA for 2 min. Before and after the use of 17% EDTA as the final rinse, the NaOCl-treated dentin powders were examined using ATR–FT-IR spectroscopy to analyze the relative loss of organic and inorganic components. Scanning (SEM) and transmission electron microscopy (TEM) were used to examine the erosion of instrumented canal walls irrigated with 5.25% NaOCl/EDTA or 1.3% NaOCl/EDTA.
Results
Compared with 1.3% NaOCl, less intact collagen remained within the subsurface of the mineralized dentin powder after the use of 5.25% NaOCl, irrespective of subsequent rinsing with 17% EDTA. Canal wall erosion was apparent only under SEM when root canals were irrigated 5.25% NaOCl followed by 17% EDTA. Under TEM examination, subsurface erosion extended 10–15 μm beneath the sealer-bonded dentin surface after the use of 5.25% NaOCl for 20 min.
Conclusion
The superficial destructive effect of NaOCl on mineralized dentin is irreversible and is present irrespective of whether EDTA is subsequently employed as the final active irrigant. The EDTA removes the collagen-depleted apatite phase to expose the underlying cause of destruction that is morphologically perceived as canal wall erosion.
1
Introduction
Canal wall smear layers produced by instrumentation of the root canal system with hand and rotary instruments contain remnant bacteria, pulpal debris, and toxins . The sequential application of sodium hypochlorite (NaOCl) and ethylenediamine tetraacetic acid (EDTA) has been conceived as a predictable means of canal irrigation for those clinicians who encompass smear layer removal as part of their root canal cleaning and shaping objectives . Previous studies have demonstrated that the use of this dual irrigation regime resulted in sporadic erosion of the canal walls that is characterized by surface dissolution of intertubular and peritubular dentin and coalescence of widened dentinal tubules , with consequential degradation of the mechanical properties of the irrigants-treated dentin . This has resulted in the introduction of alternative experimental and commercially available irrigants for removing canal wall smear layers without causing inadvertent erosion of the canal walls .
As EDTA demineralizes radicular dentin , it is logical to assume that canal wall erosion is caused by the prolonged use of EDTA or its sustained release from dentinal tubules. Thus, attempts have been made to dilute the continuous chelating effect of EDTA with the use of water as a passive final irrigant , to use a lower EDTA concentration for a longer time period , or to inactivate the chelating action of EDTA with NaOCl . For short exposure times that are clinically relevant for dentin bonding (i.e. less than one min), the demineralization action of 17% EDTA is mild when compared to the more aggressive acid conditioners employed by etch-and-rinse adhesive systems in adhesive dentistry . Erosion of crown dentin that is conditioned with EDTA for bonding purpose has never been reported in the adhesive dentistry literature. Even for longer contact times (1–2 min) that are clinically relevant for root canal irrigation, the calcium chelation capacity of EDTA is self-limiting due to the excellent buffering capacity of dentin . Thus, it is unlikely that canal wall erosion is caused solely by EDTA irrigation. As the mechanical properties of mineralized dentin are adversely affected by its prolonged contact with 3–5% NaOCl , it is possible that that NaOCl removes the organic phase from mineralized dentin, creating diffusion channels for more rapid penetration of the EDTA into the subsurface intertubular and peritubular dentin and expedites their disintegration.
Other related issues that have not been thoroughly investigated are the effects of NaOCl concentrations and exposure times on canal wall erosion after the sequential application of NaOCl and EDTA. For example, the use of 1.3% NaOCl as the initial irrigant and BioPure MTAD (Denstply Tulsa Dental Specialties, Tulsa, OK, USA) as the final irrigant had minimal effect on canal wall erosion , whereas the use of 5.25% NaOCl as the initial irrigant and 17% EDTA as the final irrigant resulted in substantial canal erosion . However, 1.3% NaOCl had not been employed in those studies to examine whether the use of 17% EDTA as the final irrigant would have resulted in a similar lack of canal wall erosion that was reported with the use of BioPure MTAD.
Thus the objectives of the present study were to examine the effects of two NaOCl concentrations (1.3% and 5.25%) and different exposure times on subsurface collagen degradation within mineralized dentin, in the absence or presence of 17% EDTA as the final irrigant, and to examine the effects of these two NaOCl concentrations on canal wall erosion following the use of 17% EDTA as the final irrigant. Understandably, the second objective is only meaningful when the experiment is conducted within the clinically relevant time period in which radicular dentin is exposed to these irrigants. The null hypothesis stated that the different concentrations of NaOCl (5.25% and 1.3%) employed as the initial irrigant had no effect on subsurface collagen degradation within mineralized dentin.
2
Materials and methods
2.1
Fourier transform-infrared spectroscopy (FT-IR)
Sixty extracted human third molars were obtained from anonymous subjects following their signed consent under a protocol approved by the Human Assurance Committee of the Medical College of Georgia. The teeth were stored in 0.9% NaCl containing 0.02% sodium azide at 4 °C to prevent bacterial growth and used within 2 months after extraction. Radicular dentin devoid of enamel, cementum and pulpal tissues were prepared from those teeth using tungsten carbide burs, barbed broaches and stainless steel files. The dentin specimens were dehydrated in acetone, placed in a stainless steel capsule containing 5 mm diameter stainless steel balls and immersed in liquid nitrogen for 15 min. The frozen assembly was triturated in a mixer mill (Model MM301, Retsch, Newtown, PA, USA) for 6 min at 30 Hz . The radicular dentin chips were sieved with a multiple-sieve sonic sieving device (Sonic Sifter, Sepor Inc., Wilmington, CA, USA) to obtain a fine powder containing aggregates that were smaller than 38 μm in diameter. Dentin chips larger than 38 μm in diameter were re-triturated and re-sieved to obtain enough dentin powder for the experiment. The mineralized dentin powder was kept frozen at −70 °C until use to prevent degradation of the collagen component.
Sixteen groups of 50 mg dentin powder (in triplicates) were immersed respectively in scintillation vials containing 50 mL of 5.25% or 1.3% NaOCl (Clorox, The Clorox Company, Oakland, CA, USA) for 10, 20, 30, 60, 120, 180 or 240 min, respectively. During the immersion period, the scintillation vials were gently agitated at 25 rpm using a platform shaker (Belly Button, Stovall Life Science Inc., Greensboro, NC, USA). After NaOCl treatment, the dentin powder aliquots were filtered, washed three times with deionized water and lyophilized using a freeze dryer (Labconco Corp, Kansas City, MI, USA). The dried dentin power aliquots were designated as Group I (5.25% NaOCl) and Group II (1.3% NaOCl only). Three additional 50 mg aliquots of freeze-dried untreated mineralized dentin powder served as the control for Groups I and II.
A Nicolet 6700 FT-IR spectrophotometer (Thermo Scientific Inc., Waltham, MA, USA) with a single-reflection attenuated total reflection (ATR) setup (Smart OMNI-Sampler, Thermo Scientific Inc.) was used for the FT-IR part of the experiment. The ATR/FT-IR spectrophotometer was used to collect infrared spectra from the dentin “superficial subsurface” as the evanescent wave reflected through the crystal and penetrated the specimen. The ATR accessory (Smart OMNI-Sampler) was equipped with a diamond crystal that has a spectral range of 30,000–250 cm −1 . The diamond crystal provides a shallow depth of penetration of the evanescent wave, which decreases exponentially with the depth of penetration into the specimen. For a germanium crystal with a refractive index of 2.4 and the angle of incidence of the infrared beam between 30° and 60°, the depth of penetration is typically between 1.1 and 2 μm, depending upon the refractive indices of the specimens . Infrared spectra of the dentin powder were collected in triplicates for each NaOCl concentration and time period. To ensure optimal “point-to-point” contact of the hard, unyielding specimen particles and to achieve consistent pressure on the particles throughout the entire experiment, a compressible 2-mm diameter “O” ring was placed over the 2-mm diameter sampling window to prevent the dentin powder from slipping and spreading during compression . The anvil of the back pressure tower of the Smart OMNI-Sampler was then pressed against the “O” ring-confined dentin powder and the slip clutch of the back pressure tower was turned clockwise until an audible click was heard. This ensured that correct pressure was applied to each powder aliquot, independent of the size or shape of the powder particles. Infrared spectra were collected between the range of 4000–400 cm −1 at 4 cm −1 resolution using 32 scans. Background spectrum was automatically subtracted from the acquired spectrum using algorithm incorporated in the OMNIC FT-IR software (Thermo Scientific Inc.). The spectral data were expressed as absorbance. The germanium crystal surface was cleaned with 70% ethanol and then acetone between sampling. After spectra collection, each aliquot of NaOCl-treated dentin powder was immersed in 17% EDTA (Sigma–Aldrich, St. Louis, MO, USA) for 2 min under gentle swiveling at 25 rpm. The powder was filtered, washed three times with deionized water and freeze-dried. Infrared spectra of the NaOCl/EDTA-treated specimens were obtained again and designated as Groups III: 5.25% NaOCl followed by 17% EDTA, and Group IV: 1.3% NaOCl followed by 17% EDTA. An additional 50 mg aliquot of dentin powder was rinsed only with 17% EDTA for 2 min, freeze-dried, and served as the control for Groups III and IV. Spectra were collected in triplicates for the control group and each experimental group, using the three respective aliquots of untreated and treated dentin powder. The mean of the “apatite/collagen ratio” derived from the spectra obtained from each group was used for statistical evaluation.
For each group, linear regression was employed to examine if a linear relationship existed between NaOCl immersion time and the mean “apatite/collagen ratio” (see Fig. 2 ) derived from each time period. For Groups I and II, the apatite–collagen ratios in each group were compared with the mineralized dentin control. For Groups III and IV, the apatite–collagen ratios in each group were compared with both the mineralized dentin control and the 17% EDTA control. As the normality and homoscedasticity assumptions appeared to be violated, the data were analyzed using Kruskal–Wallis ANOVA on ranks. Post hoc comparisons were performed with the Dunn’s multiple comparison tests against the untreated mineralized dentin control, and for Groups III and IV only, against the 17% EDTA control. For all analyses, statistical significance were set at α = 0.05.
2.2
Scanning electron microscopy (SEM)
Sixteen intact, caries-free human anterior single-rooted teeth were collected after the patients’ informed consents were obtained under a protocol reviewed and approved by the Human Assurance Committee of the Medical College of Georgia. The teeth were selected based on the presence of only one canal and similar canal width as determined by buccolingual and mesiodistal digital radiographs. They were stored in 0.9% NaCl containing 0.02% sodium azide at 4 °C. Each tooth was decoronated to obtain a standardized root length of 17 mm.
Each decoronated root was seated in a 40 cm long, 20 cm diameter Plexiglas tube containing unset, clear polyvinylsiloxane impression material (Memosil, Heraeus Kulzer Inc., South Bend, IN, USA) with the root apex located at approximately 5 mm from the base of the Plexiglas tube. Approximately 3 mm of the coronal portion of the root protruded on top of the polyvinylsiloxane to permit root canal cleaning and shaping. This setup prevented irrigant from extruding from the apical foramen during root canal preparation . The working length of each canal was determined by deducting 1 mm from the length recorded when the tip of a size 10 K-file (Dentsply Tulsa) was just visible from the apical foramen. The canals were prepared to working length with the apical seat established at size 50, 0.06 taper using stainless steel hand files and Profile nickel–titanium rotary instruments (Dentsply Tulsa). As the use of NaOCl only did not result in dissolution of the smear layer , the 16 teeth were randomly divided only into two groups (i.e. Group III: 5.25% NaOCl followed by 17% EDTA and Group IV: 1.3% NaOCl followed by 17% EDTA). During canal shaping, 1 mL of either 5.25% or 1.3% NaOCl was used to irrigate the root canal between each hand and rotary instrument. A total of 10 mL of NaOCl was used as the initial irrigant for each root canal. The irrigants were delivered to within 1 mm of the working length using 30-gauge side-vented ProRinse irrigation needles (Dentsply Tulsa). Each canal was filled with irrigant during instrumentation. The instrumentation time for each root canal was approximately 20 min. After shaping, all canals were irrigated with 5 mL of 17% EDTA as the final active irrigant for 2 min, followed by the use of 5 mL of deionized water as the final passive irrigant for another 2 min.
Four of the eight roots in each group were not obturated. The orifice of each root was temporized with 2 mm of a provisional restorative material (Tempit, Centrix Inc., Shelton, CT, USA) prior to its retrieval from the polyvinylsiloxane impression material for SEM preparation. Two longitudinal grooves were prepared in each root without perforating the canal to facilitate splitting of each root into two longitudinal halves. The root-halves were fixed in 2% glutaraldehyde, dehydrated in ascending ethanol and hexamethyldisilazane to preserve the collagen matrix created along the instrumented canal walls by EDTA irrigation and to prevent the latter from collapsing during examination by a high vacuum SEM . The dried root-halves were sputter-coated with gold/palladium, and examined using a field emission-SEM (Model XL-30 FEG; Philips, Eindhoven, The Netherlands) operated at 5 keV. Images of the middle third (i.e. 5–10 mm from the anatomic apex) of the instrumented canal walls were taken at 2000× to determine the overall extent of canal wall erosion and at 7500× to record details of dentin erosion.
2.3
Transmission electron microscopy (TEM)
The remaining four roots in each group were similarly temporized with the provisional restorative material and retrieved from the polyvinylsiloxane impression material. Each root was split into two root-halves as previously described. As it is difficult to perform ultramicrotomy of a thermoplastic root filling material without resorting to cryo-TEM, the more intact root-half from each root was rehydrated in deionized water, dried with paper points and filled entirely with a self-adhesive methacrylate resin-based root canal sealer (RealSeal SE, Sybron-Endo, Orange, CA, USA) that has limited self-etching potential . The latter was placed over the canal space without the adjunctive use of a thermoplastic root filling material. The use of a split root-half model instead of obturating an intact canal also helped to minimize the effect of polymerization shrinkage stresses on sealer adhesion along the cavity walls by reducing the cavity configuration factor of the root canal . The sealer was lighted-cured using a quartz–tungsten–halogen light-curing unit with an output intensity of 600 mW/cm 2 . After light-curing, the surface oxygen inhibition layer was removed using a piece of lint-free tissue prior to further TEM processing.
A 2-mm thick transverse section containing the sealer-radicular dentin interface was prepared from each root-half using a diamond-coated Isomet Saw (Buehler Ltd., Lake Bluff, IL, USA) under water cooling. Sectioning was performed at 7–9 mm from the apical foramen, corresponding to the middle third of the canal. The specimens were fixed initially in Karnovsky’s fixative for 8 h, post-fixed with 1% osmium tetroxide for 1 h and progressively dehydrated by passing them through an ascending ethanol series (50–100%) for 1 h each. Following three changes of absolute ethanol, the latter was replaced with propylene oxide as a transitional medium to enable specimen infiltration by the ethanol-insoluble epoxy resin embedding medium. Specimens were subsequently embedded in pure epoxy resin. Non-demineralized, 90–120 nm thick sections were examined without further staining using a JEM-1230 TEM (JEOL, Tokyo, Japan) operated at 110 keV.
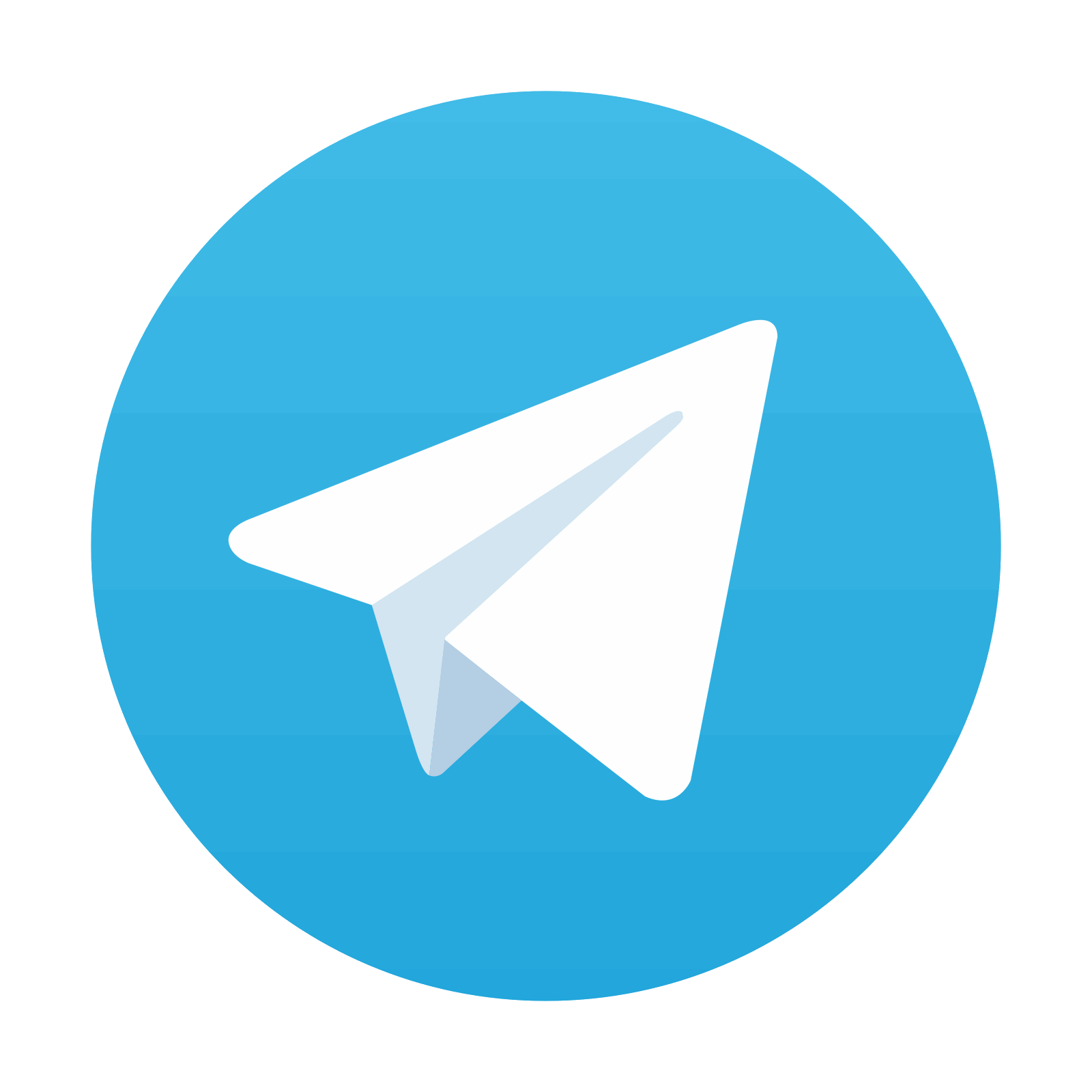
Stay updated, free dental videos. Join our Telegram channel

VIDEdental - Online dental courses
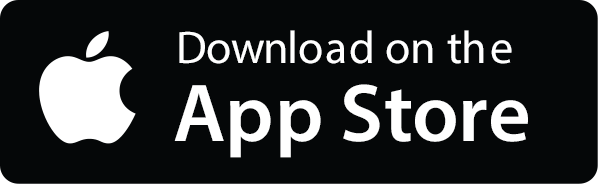
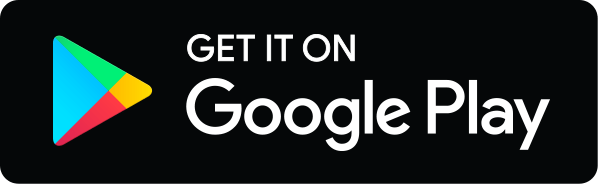