Abstract
Objective
The aim of this investigation was to determine reaction enthalpy, ion viscosity and curing light transmission changes of unfilled methacrylate-based systems in order to compare methods that monitor photoactive resin polymerization.
Methods
Photoinitiator (0.2%, w/v, camphoroquinone), accelerator (0.3%, w/v, amine) and inhibitor (ranging from 0 to 1%, w/v, butylated hydroxytoluene, BHT) were incorporated in an experimental BisGMA/TEGDMA co-monomer mixture (50/50, w/v). The concentration of BHT was varied from 0.00, 0.01, 0.05, 0.10, 0.50 to 1.00% (w/v). Light transmission (LT), reaction enthalpy (UV-differential scanning calorimetry, DSC), and ion viscosity (dielectrical analysis, DEA) were determined during irradiation of the resins (40 s; halogen light curing-unit). Statistical analysis was performed using two-way ANOVA followed by post hoc tests ( α = 0.05). Curve fitting and regression calculation were done.
Results
There was no significant change in the time to reach the maximum rate of polymerization (reaction time) in the individual systems up to a BHT concentration of 0.05% ( P > 0.05). Starting at a concentration of 0.10% BHT an increase in time of reaction could be found from 4.0 s (LT), 4.07 s (DEA) and 4.9 s (DSC) to a maximum of 7.4 s (DSC), 9.43 s (DEA) and 9.67 s (LT). Linear increase ( y = 5.588 × x ) in time to the maximum speed of reaction could be found with a correlation of R 2 = 0.992.
Conclusions
The speed of polymerization reaction is strongly influenced by BHT concentration. The linear relationship should allow for the prediction of the speed of reaction during blending of a methacrylate-based resin. The three test systems allow for monitoring the complex polymerization kinetics of unfilled methacrylate-based systems.
1
Introduction
The majority of dental composites consist of dimethacrylate resins reinforced with inorganic filler particles. The properties of the composite can be optimized by the manufacturer to suit a particular application (e.g. Class IV anterior or Class II posterior cavities, filled adhesives) by varying the filler (volume percentage, filler size and particle distribution) and polymer matrix composition. Smaller, more flexible monomer chains, such as those in triethylene glycol dimethacrylate (TEGDMA) and hydroxyethyl methacrylate (HEMA) in larger volumes are used to create less viscous but highly cross-linked systems. Complex, bulky monomers with phenolic rings (bisphenol glycol dimethacrylate, BisGMA) or urethane components (urethane dimethacrylate, UDMA) with higher molecular weight allow for the fabrication of higher strength, more viscous resins. Other components may be modified to alter reactivity of the system and influence network formation (by varying initiator, co-initiator types and concentrations, dye and pigment inclusions) and improve shelf-life (UV-stabilizers and inhibitors). Polymerization retarders or inhibitors are added to improve shelf-life and operatory light stability during in vivo placement and contouring but may also influence polymerization kinetics and degree of cure of the polymer .
For monitoring curing characteristics and efficiency of polymerization, differential scanning calorimetry (DSC) , Fourier transform infrared spectroscopy (FTIRS) , rheology, temperature measurements, simple micro-hardness tests or dielectric analysis (DEA) are used. For characterization of photopolymerization reactions in real-time a fast testing frequency and a high data acquisition rate of measurement is essential. In DEA, the specimen is exposed to an alternating sinusoidal voltage. The dielectric specimen affects the amplitude and phase of the input signal depending on ionic mobility and the alignment of the dipoles in the alternating current field . It is considered that ion mobility is reduced during the polymerization reaction and finally stopped, so the change of ion viscosity is regarded as an indicator for the reaction and degree of cure. This allows for monitoring the chemical process of the resin from monomer with different viscosities over gelation to an insoluble polymer .
The first studies of dynamic measurements of light transmission (LT) during polymerization of light-activated composites identified that variations in light unit irradiance over time and changes induced by the polymerization reaction process itself influenced transmission and also proposed LT monitoring as a simple technique for determining appropriate radiation time for any LCU/composite combination . Since then additional work has led to an improved understanding of the effects of photoinitiator absorption and filler/resin refractive index mismatch changes on the cure behavior of unfilled resins and resin composites .
DSC investigations on the reaction of resin polymers have been described and measurements are based on enthalpy changes during the reaction of the system. It is assumed that following repeated irradiation of a photoreactive system the resin is converted to a maximum degree. The calculation of the enthalpy (area below the heat flow over time of the first polymerization peak) gives the energy of the thermal and chemical reaction (including absorption/reflection). The curve provides information about the speed and enthalpy of reaction.
The hypothesis of this investigation was that the measurement of reaction enthalpy, ion viscosity and curing light transmission changes of unfilled methacrylate-based systems allow for a combined interpretation of polymerization characteristics.
2
Materials and methods
The investigated resin composed of a BisGMA/TEGDMA co-monomer mixture (50/50, w/v). The photoinitiator (0.2%, w/v, camphoroquinone), accelerator (0.3%, w/v, amine) and inhibitor (ranging from 0 to 1%, w/v, butylated hydroxytoluene, BHT) were incorporated. The concentration of BHT varied from 0.00, 0.01, 0.05, 0.10, 0.50 to 1.00% (w/v).
All specimens ( n = 3) for each monomer formulation were irradiated for 40 s with a quartz tungsten halogen, QTH light curing-unit, LCU (Elipar Trilight, 3M EPSE, Seefeld, Germany;) exit window operating at 900 ± 50 mW/cm 2 throughout the present work. The QTH LCU was fitted with a 12 mm diameter light guide exit window.
2.1
Light transmission
Experimental resins were transferred from a lightproof dropper bottle into the cavity (4 mm diameter) of a black nylon disc mould (24 mm diameter, 2 mm thickness) taking care not to incorporate air bubbles. Opposing surfaces were covered with mylar matrix strips. Changes in light transmission (LT) throughout polymerization of specimens were recorded with an established photodiode technique , where the light curing-unit was held concentrically in an alignment ring for reproducible curing of the resin specimens. Light passes through the specimen above a diffuser to homogenize irradiation and eliminate effects of light dispersion. Light is detected through a 4 mm diameter aperture by a calibrated photodiode at the base of the lightproof box and the voltage output processed by a computer ( Fig. 1 a) . LT traces of the polymerizing monomers were normalized against light output since the operation efficiency decreased as the unit warmed up and irradiance fell by approximately 5% over the 40 s radiation time.

2.2
UV-differential scanning calorimetry (DSC)
Special modified UV-DSC (DSC 204 F1, Netzsch, Selb, Germany) was used in combination with the light curing-unit, which was positioned directly above the specimen pan. The reference pan was kept empty. All measurements were performed isothermally at 37 °C. N 2 -atmosphere (20 ml/min) was used to avoid an oxygen inhibition layer. Each specimen (15 ± 0.04 mg) was exposed to five 40 s curing cycles, with a 2 min pause in between each cycle ( Fig. 1 b). Data acquisition rate was 10 s −1 . Control measurements without resin and with resin but without light activation were performed. The speed of reaction (height of the peak) and enthalpy of reaction (peak area) curves ( f = conversion) were evaluated, as well as time to maximum speed and maximum peak (Netzsch complex evaluation program). The heat of reaction (Δ H R ) curve was calculated according to Eq. (1) :
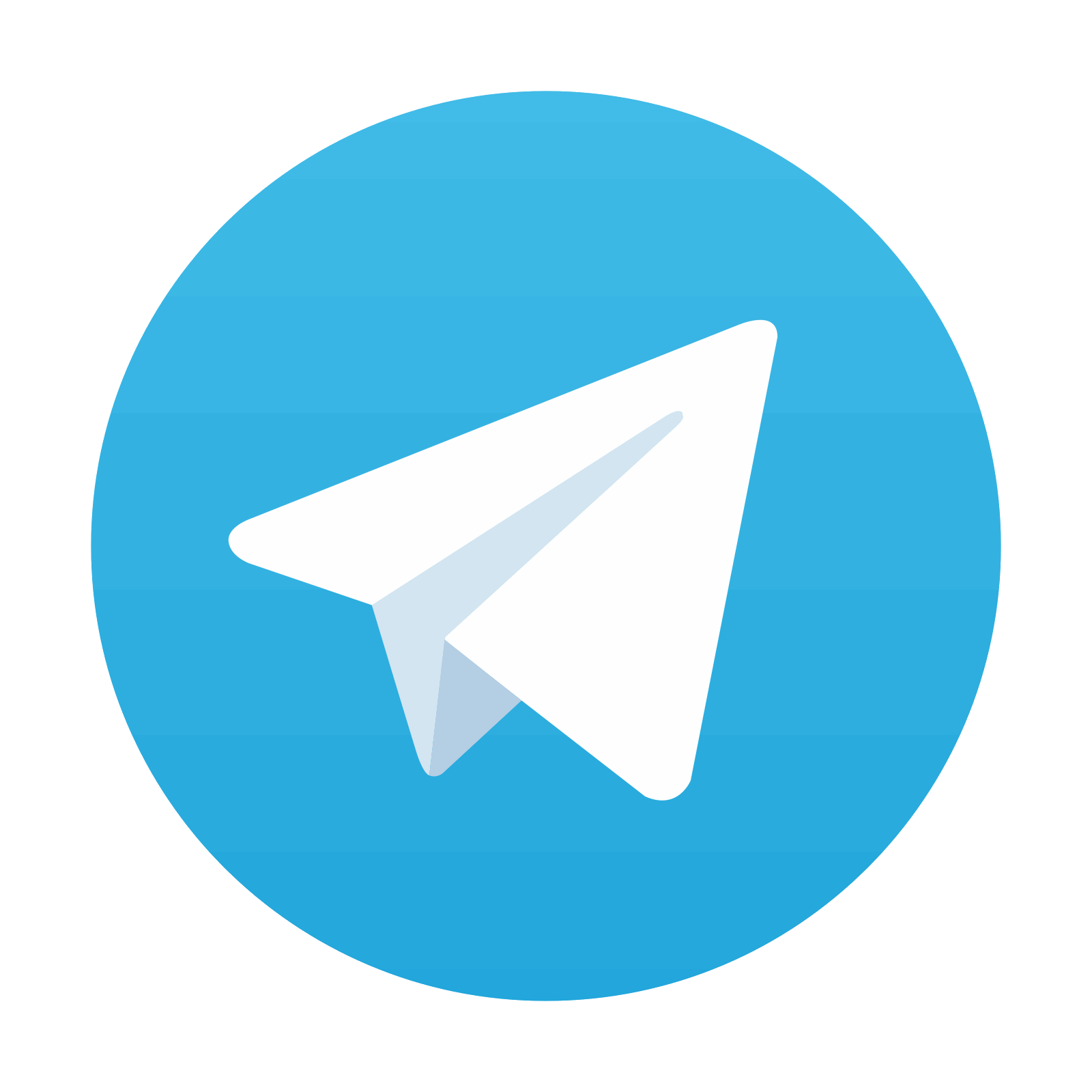
Stay updated, free dental videos. Join our Telegram channel

VIDEdental - Online dental courses
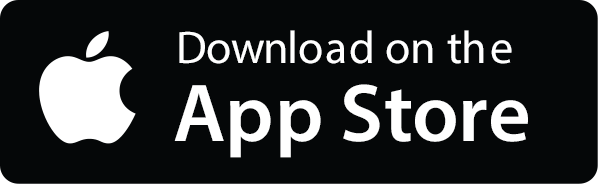
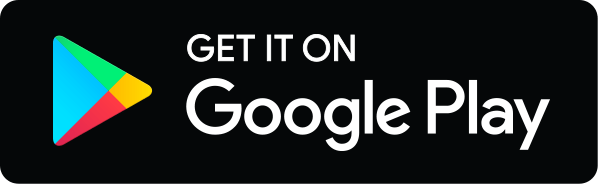