Highlights
- •
The non-uniform nature of the beam can affect the curing profile of composites.
- •
TPO can reduce the yellowness without affecting cure efficiency of CQ-composites.
- •
Resin-based composites containing only TPO resulted in reduced depth of cure.
Abstract
Objective
To evaluate the effect of combining camphorquinone (CQ) and diphenyl(2,4,6-trimethylbenzoyl)phosphine oxide (TPO) on the color and curing profile of resin-based composites.
Methods
Experimental composites were produced with different CQ and TPO molar concentrations: CQ-only, 3CQ:1TPO, 1CQ:1TPO, 1CQ:3TPO, and TPO-only. Polywave LED was characterized using a beam profiler. Block-shaped samples (5 mm × 5 mm × 3 mm depth) were cured in a custom-designed mold with a polywave LED positioned to compare the regions exposed to the 420–495 nm and 380–420 nm LED emittances. To map the cure profile, degree of conversion (DC) of longitudinal cross-sections from each block were evaluated by FT-NIR. Color, light-transmittance and light-absorption during curing were evaluated on specimens 1–3 mm thick. Data were analyzed using ANOVA/Tukey’s test ( α = 0.05; β = 0.2).
Results
Though the polywave LED beam profile was non-uniform, up to a depth of 2 mm no differences in DC were found among the composites containing CQ with TPO added up to 50%, regardless of the position under the curing tip. Composites with higher TPO concentration showed a decrease in DC beginning with a depth of 1 mm, while composites with higher or similar CQ concentrations did not show decreased DC until a depth of 3 mm. Higher TPO concentration reduced initial yellowness and color change after curing; and lower CQ concentration decreased light-absorption at greater depths.
Significance
The combination of CQ with TPO added up to 50% reduced the yellowness and color change of composites after curing without affecting cure efficiency up to a depth of 2 mm.
1
Introduction
It is suggested that the main requirement to ensure clinical success of resin-based composites (RBCs) is the appropriate light curing of the entire restoration . Although this concept is logical, light curing is a complex process that to be most effective depends on the photoinitiators within the entire restoration receiving sufficient radiant exposure at the correct wavelengths . The radiant exposure is defined as the total irradiance (mW/cm 2 ) that the material receives from the curing light over a certain time of light application (s) . Thus, ideal curing of a composite restoration depends on a calibrated and sufficiently powerful curing light being used during a minimum specified time of exposure, and with a correct light-delivery technique .
Most RBCs contains camphorquinone (CQ) as the conventional photoinitiator system, which absorbs most efficiently at approximately 460–470 nm; but some RBCs may contain alternative photoinitiators, such as diphenyl(2,4,6-trimethylbenzoyl) phosphine oxide (TPO), that absorb light most effectively within a lower wavelength range. These photoinitiators are often included as a substitute for CQ in commercial resin-based materials for esthetic restorations to reduce the yellow coloration . However, as the efficiency of these molecules depends on their absorption of light in a different wavelength range than CQ, newer polywave LED curing lights that emit additional light at lower wavelengths near the UV range (i.e. 380–420 nm) may be more optimal for light curing than the original monowave units that emit predominantly in the blue wavelength range (i.e. 420–495 nm) . However, because the precise composition of commercial materials is not always fully divulged and the instructions for use are not always described in sufficient detail , it is sometimes challenging to match materials with curing lights. Further, the physical geometry of the individual LEDs within the curing lights typically produce a non-uniform distribution of emitted wavelengths across the surface of the light guide of polywave LED units , and this may also affect the curing efficiency of resin-based materials .
A correlation between the non-uniformity of emitted light and the microhardness of RBCs up to 1.2 mm in thickness has been demonstrated ; however, it is not clear that this result will also be reflected throughout the entire depth of the typical restoration . Other factors, such as differences in the refractive index between the resin matrix and the filler, can influence the light distribution through RBCs , making it difficult to predict the overall effect of the beam profile on the curing profile of commercial RBCs containing different photoinitiators. The effect of the non-uniform nature of the beam profile of polywave LEDs on the curing profile of RBCs having different photoinitiator systems has not been fully explored.
Thus, the aim of this study was to evaluate the influence of two different photoinitiators having different wavelength absorption ranges: CQ (with absorption range in the visible wavelengths at 420–495 nm, i.e. blue spectral region), TPO (with absorption range into the violet wavelengths at 380–420 nm) and their combination (CQ and TPO), on light-transmittance, light-absorption and curing profile of RBCs exposed to a polywave unit. As the initial purpose of substituting the CQ with an alternative photoinitiator is to reduce yellowing, this study also evaluated the color before and after curing of the composites with the different photoinitiator systems. The tested hypotheses were that: (1) the non-uniform light emitted from the polywave LED would affect the curing profile of the RBCs and (2) the combination of CQ and TPO in the formulation would increase depth of cure compared to the TPO-only system and reduce yellowness and color change compared to the CQ-only system.
2
Materials and methods
2.1
Experimental resin-based composites
Table 1 lists the monomers and filler particles and their concentrations used in the experimental dental composites . The monomers were blended using a centrifugal mixing device (SpeedMixer, DAC 150.1 FVZ-K, Hauschild Engineering, Hamm, North Rhine-Westphalia, Germany). To this resin blend, different molar concentrations of CQ-amine (1:1) and TPO were added ( Table 2 ). Subsequently, the filler particles were added, first by pre-mixing the fumed silica filler with the monomer blend for 30 s at 3000 rpm, followed by the barium borosilicate glass filler for 1 min at 3500 rpm. Then, each resin-based composite was mixed one final time for 1 min at 3500 rpm under vacuum to eliminate porosities.
Material | Chemical | Refractive index b | Concentration (wt%) | Manufacturer |
---|---|---|---|---|
Monomers | Bis-GMA a | 1.552 | 10.15 | Sigma–Aldrich, St. Louis, MO, USA |
Bis-EMA a | 1.535 | 11.375 | ||
UDMA a | 1.461 | 11.375 | ||
TEGDMA a | 1.483 | 2.1 | ||
Fillers | 0.05 μm fumed silica | 1.535 | 13 | Nippon Aerosil Co. Ltd., Tokyo, Japan |
0.7 μm BaBSiO 2 a | 1.541 | 52 | Esstech Inc., Essington, PA, USA |
a Bisphenol A diglycidylmethacrylate (Bis-GMA); ethoxylated bisphenol A diglycidyldimethacrylate (Bis-EMA); triethylene glycol dimethacrylate (TEGDMA); urethane dimethacrylate (UDMA), barium borosilicate glass (BaBSiO 2 ).
Photoinitiator systems a | Molar ratio CQ:TPO | Concentration (wt%) | ||
---|---|---|---|---|
CQ | EDMAB | TPO | ||
CQ-only | 1:0 | 0.2 | 0.2 | 0 |
3CQ:1TPO | 3:1 | 0.15 | 0.15 | 0.1 |
1CQ:1TPO | 1:1 | 0.1 | 0.1 | 0.2 |
1CQ:3TPO | 1:3 | 0.05 | 0.05 | 0.3 |
TPO-only | 0:1 | 0 | 0 | 0.4 |
a Camphorquinone (CQ); ethyl 4(dimethylamino) benzoate (EDMAB); diphenyl(2,4,6-trimethylbenzoyl) phosphine oxide (TPO).
2.2
Curing light characterization
The mean radiant exposure of the polywave LED (Valo Cordless, Ultradent, South Jordan, UT, USA) was evaluated during 10 s of application in the standard mode using a portable spectrometer-based instrument (CheckMARC, BlueLight Analytics, Nova Scotia, Canada). The radiant exposure at 380–420 nm, 420–495 nm and over the whole range of wavelengths was calculated by integrating the irradiance versus wavelength curves obtained from a spectrometer (MARC Resin Calibrator, BlueLight Analytics, Nova Scotia, Canada).
The beam profile of the polywave curing light was characterized by BlueLight Analytics. The irradiance and wavelengths of the emitted light distributed across the light tip was measured at the emitting surface using a laser beam analyzer (Model SP503U, Ophir-Spiricon, Logan, UT) attached to a x – y – z positioning device mounted on an optical bench in order to standardize the positioning of the light beam in contact with the diffusive surface of the frosted quartz target (DG2X21500, Thor Laboratories, Newton, NJ) while the resulting image was recorded with the optical analysis software. The software was calibrated according to the pixel scale of the camera and the pixel dimensions to enable precise linear measurement of the light intensities. Lastly, narrow bandpass filters with full width at half maximum of 10 nm (FWHM = 10) (Thor Laboratories, Newton, NJ, USA) were used to differentiate the spectral output in the 400–410 nm and 455–465 nm wavelength peak regions.
2.3
Absorption spectrophotometric analysis
Spectrophotometric analysis was performed in order to evaluate the absorption of the photoinitiators in the 360–540 nm wavelength range using a UV–vis spectrophotometer (U-2450, Hitachi High-Technologies, Chiyoda, Tokyo, Japan). Each photoinitiator was diluted in 1 mL of >99.5% ethanol (Sigma–Aldrich, St. Louis, MO, USA) at the same concentration as presented in Table 2 for CQ- and TPO-only. The spectra were collected using a quartz cell with a path length of 1 cm.
2.4
Curing profile
Block-shaped specimens (5 mm × 5 mm, 3 mm depth) of the experimental composites were produced by curing the material with a radiant exposure of 10,000 mJ/cm 2 (1000 mW/cm 2 for 10 s) in a custom-designed polymethylmethacrylate (PMMA) mold using a polywave LED (Valo Cordless, Ultradent, South Jordan, UT, USA) with the light tip placed directly on the specimen surface with a Mylar strip covering the top ( n = 5). A jig was made to reproducibly position the LED in order to establish the regions of the block directly exposed to the 380–420 nm and 420–495 nm LED emittances from the polywave unit ( Fig. 1 ). To map the degree of conversion (DC) from top to bottom and across the breadth of the block, the block was sectioned twice with a diamond saw to produce a 5 mm × 3 mm longitudinal cross-section (0.5 mm thick) of the center of the block which was evaluated with a FT-NIR microscope (Nicolet Continuum, Thermo Scientific) coupled to a FT-NIR spectrometer (Nicolet Nexus 6700, Thermo Scientific). Spectra were obtained by the co-addition of 64 scans at a resolution of 4 cm −1 . Vinyl conversion was determined based on the aliphatic absorption band at 6165 cm −1 , using the aromatic band at 4623 cm −1 as an internal reference . Spectra were collected for the polymerized longitudinal cross-section specimens of each restoration across the entire length and depth of the cross-section and for a non-polymerized sample of each experimental composite with the same thickness (0.5 mm) sandwiched between two glass plates. The degree of conversion (DC, in %) was calculated as follows: (1 − ( R pol/ R non-pol) × 100), where R is the ratio of peak areas at 6165 cm −1 and 4623 cm −1 in polymerized (pol) and non-polymerized (non-pol) specimens.
The results were exported to create color-coded maps to describe the DC as a function of position under the light beam using the Origin Pro software (OriginLab Co., Northampton, MA, USA). The map scales were set to indicate the maximum DC achieved and a reduction of 10% of the maximum DC in each subsequent color-coded area.
2.5
Color measurements
Samples of 1–3 mm thickness of each composite were made in Delrin rings (Ø = 6 mm) with Mylar strips covering the top and bottom. The color parameters of each specimen ( n = 3) were obtained with a D65 illuminant over a white background (CIE L * = 91.1, a * = 1.2 and b * = −3.4, Y = 78.8) using a calibrated chromameter (CR-221, Konica Minolta, Tokyo, Japan) with a sensor-opening diameter of 4 mm. The sensor opening of the chromameter was placed in the middle of each specimen and three measurements were taken before and after light curing.
The CIELab coordinates ( L *, a *, b *) from the pre- and immediately post-cured specimens were used to evaluate color change (Δ E 00 ) according to the CIEDE2000 formula (CIE, 2004)
Δ E 00 = Δ L K L ⋅ S L 2 + Δ C K C ⋅ S C 2 + Δ H K H ⋅ S H 2 + R T ⋅ Δ C K C ⋅ S C ⋅ Δ H K H ⋅ S H 0.5 ,
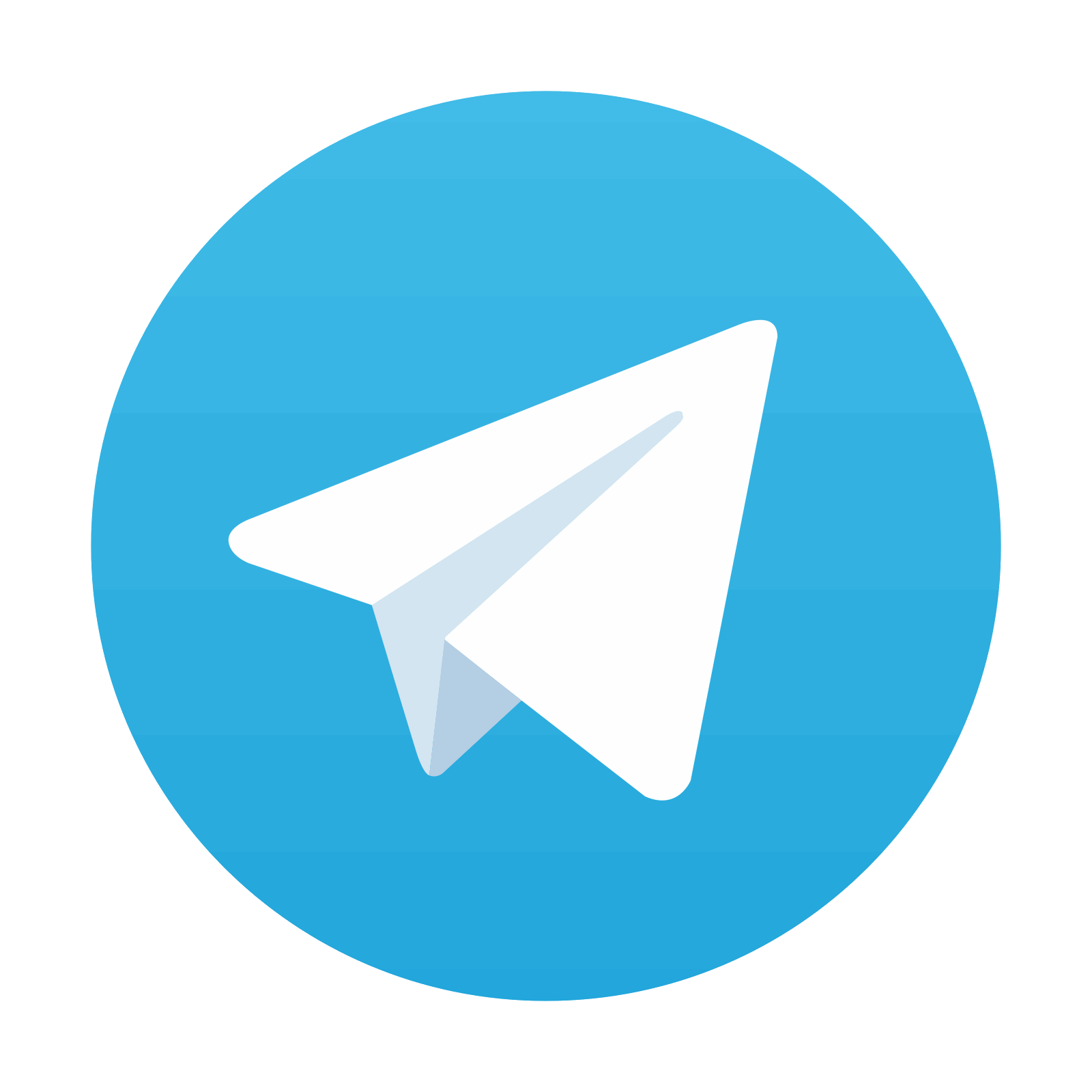
Stay updated, free dental videos. Join our Telegram channel

VIDEdental - Online dental courses
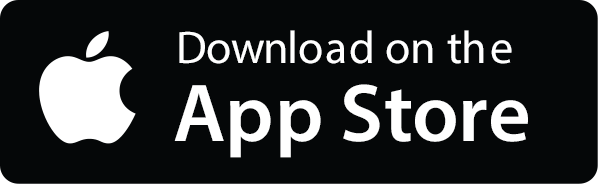
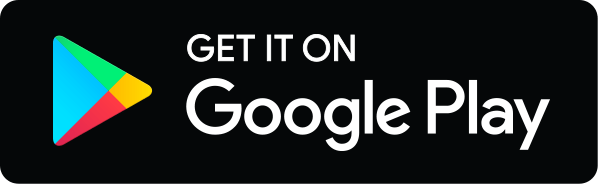