Dental caries is a site-specific disease that undergoes many cycles of demineralization and remineralization during lesion development. Because of its developmental characteristics dynamics, the caries lesion can be arrested and even repaired at its early stages without operative intervention by increasing the net mineral gain during the demineralization and remineralization cycles. This result can be accomplished by reducing the effect of etiological factors such as cariogenic biofilms and diet, and increasing the efficacy of remineralizing agents such as saliva and fluoride.
This article discusses the main changes in tooth structure that occur during the formation, progression, and regression of caries lesions, with a particular focus on chemical and histologic events with direct relevance to the clinician.
Dental caries lesions arguably start to develop when demineralization of the tooth surface goes beyond the mineral exchanges that occur regularly between crystals at the surface of the tooth and the surrounding environment. Although, from the mechanistic point of view, these early events are important, from the clinical point of view early demineralization does not become relevant until it becomes clinically visible or detectable by other means (eg, radiography; Fig. 1 ). This clinical threshold is certain to change when new, more sensitive detection instruments become available. When conditions favorable for progression of the lesion continue for a significant time, these incipient noncavitated lesions continue to progress until the surface of the lesion collapses and a cavity is formed ( Fig. 2 ). As the cavitated lesion continues to progress, the tooth can lose its vitality with a high risk of developing an infection beyond the tooth boundaries invading periapical tissues ( Fig. 3 ).
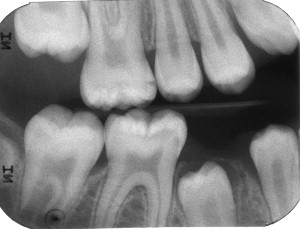
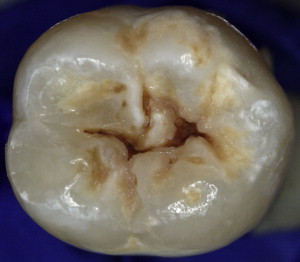
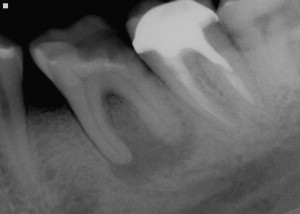
The dynamic tooth surface
Not all mineral loss from tooth structure is part of a pathologic process such as dental caries disease. Crystals at the tooth surface regularly go through natural periods of mineral loss (demineralization) and mineral gain (remineralization), particularly in surfaces covered by undisturbed (stagnant) biofilms (ie, dental plaque). Immediately after eruption into the oral cavity, teeth are colonized by oral bacteria that create conditions that, in combination with saliva, modify the composition of teeth surfaces, making them more resistant to dental caries. This process has been called posteruptive enamel maturation and it is critically important for the caries process because it occurs in the tooth area with direct contact with the oral environment, where lesions start to develop.
Enamel surface crystal composition at eruption time has large amounts of carbonate, water, and magnesium, among other elements, and it is porous. After undergoing many periods of demineralization and remineralization, the chemical composition and structure of surface enamel becomes more amorphous; less porous; contains less water, carbonates, and magnesium; and has increased amounts of fluoride and organic material. These substitutions in the crystal surface are beneficial to the tooth surface because the new, matured tooth surface is less soluble and more resistant to caries challenges.
A similar posteruptive maturation process is expected to occur on exposed root surfaces when the cementum or dentin is exposed to the oral environment after gingival recession. Cementum is typically lost quickly as a result of wear forces such as toothbrushing and scaling/root planning, and the highly porous and soluble newly exposed dentin undergoes similar demineralization and remineralization cycles, thus creating a much less porous dentinal surface containing larger amounts of minerals, particularly calcium, phosphate, and fluoride. This increase in crystal size and concentration significantly reduces the permeability and caries susceptibility of the root surface, as happens in enamel.
Crystals at the enamel and dentin/cementum surfaces covered by undisturbed biofilms are likely to continue having mineral exchanges for as long as the covering biofilms are able to create conditions of undersaturation and supersaturation with respect to the crystals. Undersaturation occurs mainly under acidic conditions by the loss of phosphate and hydroxyl ions that react with the hydrogen ions being produced by the metabolic activity of the covering biofilms in the presence of fermentable carbohydrates, particularly when they are frequently present; conditions commonly present in caries-active individuals. In reaction to undersaturation conditions, minerals are released from multiple sources: saliva, bacteria, calculus, calcium-fluoride formations, and the tooth surface itself. After a period of time, saturation at the plaque fluid stabilizes and mineral loss stops. When enough minerals (mainly calcium, phosphate, and hydroxyl ions) are available in the solution surrounding the crystal increasing its saturation level, balance returns. At some point the pH can increase to a point at which supersaturation conditions can occur. Under those conditions, the tendency of the solution is to precipitate minerals to return to saturated conditions. During this period of supersaturation, minerals can remineralize partially demineralized enamel crystals and, when supersaturation occurs for long periods of time, dental calculus can form, although protein inhibitors tend to prevent this from happening. Therefore, although pH is the strongest determinant for saturation level leading to demineralization or remineralization under clinical conditions, it is not the only important factor because saturation is significantly affected by other factors such as the concentration of calcium and phosphate ions and the total ionic strength of plaque fluid. This is clinically relevant because patients with significant decrease in salivary flow would have difficulty supplying enough minerals and buffering the fluid to maintain adequate saturation levels to maintain tooth surface integrity. There are other clinically relevant factors, such as the presence of calculus nearby, that also affect this process. Calculus crystals are less stable than those in tooth structure and therefore demineralize at higher saturation levels, releasing minerals into the plaque fluid protecting the tooth structure. Several clinical studies have shown an inverse relationship between caries and calculus.
Caries lesion formation; enamel
Under cariogenic conditions, the naturally increased tooth surface resistance is not enough to prevent the formation of a caries lesion. When, for a significant amount of time, the amount of minerals lost during those exchanges is larger than the amount of minerals gained, a caries lesion starts to develop in the enamel surface. The roughness and porosity of the tooth surface increases, presenting an eroded appearance at high magnification. Increased porosity allows the development of a subsurface lesion, which is characteristic of caries lesions, distinguishing it from other types of demineralization such as dental erosion caused by stronger acids that promotes the loss of enamel structure layer by layer.
Caries lesions develop in the subsurface because of a series of unique phenomena that occur mainly around the lesion surface. Crystals at the tooth surface become more resistant to demineralization through the posteruptive maturation process and the formation of the lesion itself, leaving the subsurface crystals more susceptible to undersaturation conditions being created by the diffusion of hydrogen ions from plaque fluid. Moreover, the surface layer has better saturation conditions because, in addition to having access to ions coming from the body of the lesion and plaque fluid/saliva, it is also covered by the salivary pellicle, which acts as a diffusion barrier slowing down the outward diffusion of ions. These conditions increase saturation levels of calcium, phosphate, and fluoride at the surface, enhancing the probability of remineralization and reducing those of demineralization, with the added consequence of the solution penetrating to the subsurface being much less saturated and with a reduced remineralization potential. Consequently, fluoride has a much larger impact in the surface layer, forming many crystal areas of less soluble fluoridated hydroxyapatite, which is more acid resistant and requires lower saturation levels before it starts to dissolve. These conditions allow the caries lesion (demineralization secondary to acid penetration) to develop well into dentin without breakage of the surface, and it is probably bacteria free in most cases because bacteria are physically too large to fit into the diffusion spaces of a seemingly intact surface layer.
Mineral surfaces are covered with organic material that restricts ion exchange, limiting the rate of demineralization and remineralization by acting as a diffusion barrier for mineral exchanges. Some laboratory evidence suggests that the net result of this restriction is enhanced protection by a reduction in the amount of hydrogen ions going in and the amount of calcium and phosphate going out, increasing the saturation level at those specific locations. Furthermore, fluoride ions are likely to concentrate at those locations and only sub–parts per million (ppm) levels of fluoride near the crystal are needed to significantly affect saturation levels. Therefore, lesion progression is not only determined by the level of undersaturation but also by the ability of the ions to diffuse in and out of the lesion, which has been shown to be slow.
Large portions of the noncavitated lesion can be remineralized if the saturation condition cycle shifts to conditions in which more mineral is gained than lost. Under these favorable conditions, remineralization of the lesion surface is more likely to occur first, leading to the blockage of the communication channels that allowed the demineralization of the subsurface of the lesion. These particular dynamics lead to the formation of a remineralized surface that is highly resistance to demineralization (probably more resistant than sound enamel ), leaving a sealed, partially demineralized subsurface lesion. Although this situation might concern some clinicians, many noncavitated caries lesions develop and become arrested naturally, with no detrimental clinical consequences. As long the saturation conditions in the biofilms covering those lesions do not return to cariogenicity, those lesions do not progress and teeth are fully functional. Many early noncavitated (white and brown spot) lesions will continue to be visible clinically and radiographically after remineralization because most of the detection signal comes from the body of the lesion, where changes in its light scattering and radiolucency are unlikely because of its limited remineralization potential ( Fig. 4 ).
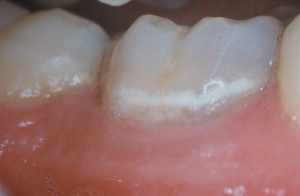
Caries lesions develop following the shape of the undisturbed biofilms covering the tooth surface where the lesion is developing. For example, early caries lesions in the proximal surfaces typically are curved following the cervical margin of the contact. In occlusal surfaces, where most of the caries lesions develop, most lesions develop at the entrance of fissures and fossae and the bottom of wider ones. These are areas of higher risk because of their limited salivary access and propensity to accumulate undisturbed biofilms. Therefore, conditions leading to the growth of undisturbed biofilms create site-specific high-risk situations that might lead to the development of caries lesions. Good examples are occlusal surfaces of erupting teeth that are not yet fully functional in mastication and are easily covered by significant amounts of undisturbed biofilm, greatly increasing the risk for lesion development or large gaps between dental restorations and tooth structure in approximal surfaces, also promoting the accumulation of undisturbed biofilm and consequently increasing the risk of developing secondary caries lesions.
Caries lesion formation; enamel
Under cariogenic conditions, the naturally increased tooth surface resistance is not enough to prevent the formation of a caries lesion. When, for a significant amount of time, the amount of minerals lost during those exchanges is larger than the amount of minerals gained, a caries lesion starts to develop in the enamel surface. The roughness and porosity of the tooth surface increases, presenting an eroded appearance at high magnification. Increased porosity allows the development of a subsurface lesion, which is characteristic of caries lesions, distinguishing it from other types of demineralization such as dental erosion caused by stronger acids that promotes the loss of enamel structure layer by layer.
Caries lesions develop in the subsurface because of a series of unique phenomena that occur mainly around the lesion surface. Crystals at the tooth surface become more resistant to demineralization through the posteruptive maturation process and the formation of the lesion itself, leaving the subsurface crystals more susceptible to undersaturation conditions being created by the diffusion of hydrogen ions from plaque fluid. Moreover, the surface layer has better saturation conditions because, in addition to having access to ions coming from the body of the lesion and plaque fluid/saliva, it is also covered by the salivary pellicle, which acts as a diffusion barrier slowing down the outward diffusion of ions. These conditions increase saturation levels of calcium, phosphate, and fluoride at the surface, enhancing the probability of remineralization and reducing those of demineralization, with the added consequence of the solution penetrating to the subsurface being much less saturated and with a reduced remineralization potential. Consequently, fluoride has a much larger impact in the surface layer, forming many crystal areas of less soluble fluoridated hydroxyapatite, which is more acid resistant and requires lower saturation levels before it starts to dissolve. These conditions allow the caries lesion (demineralization secondary to acid penetration) to develop well into dentin without breakage of the surface, and it is probably bacteria free in most cases because bacteria are physically too large to fit into the diffusion spaces of a seemingly intact surface layer.
Mineral surfaces are covered with organic material that restricts ion exchange, limiting the rate of demineralization and remineralization by acting as a diffusion barrier for mineral exchanges. Some laboratory evidence suggests that the net result of this restriction is enhanced protection by a reduction in the amount of hydrogen ions going in and the amount of calcium and phosphate going out, increasing the saturation level at those specific locations. Furthermore, fluoride ions are likely to concentrate at those locations and only sub–parts per million (ppm) levels of fluoride near the crystal are needed to significantly affect saturation levels. Therefore, lesion progression is not only determined by the level of undersaturation but also by the ability of the ions to diffuse in and out of the lesion, which has been shown to be slow.
Large portions of the noncavitated lesion can be remineralized if the saturation condition cycle shifts to conditions in which more mineral is gained than lost. Under these favorable conditions, remineralization of the lesion surface is more likely to occur first, leading to the blockage of the communication channels that allowed the demineralization of the subsurface of the lesion. These particular dynamics lead to the formation of a remineralized surface that is highly resistance to demineralization (probably more resistant than sound enamel ), leaving a sealed, partially demineralized subsurface lesion. Although this situation might concern some clinicians, many noncavitated caries lesions develop and become arrested naturally, with no detrimental clinical consequences. As long the saturation conditions in the biofilms covering those lesions do not return to cariogenicity, those lesions do not progress and teeth are fully functional. Many early noncavitated (white and brown spot) lesions will continue to be visible clinically and radiographically after remineralization because most of the detection signal comes from the body of the lesion, where changes in its light scattering and radiolucency are unlikely because of its limited remineralization potential ( Fig. 4 ).
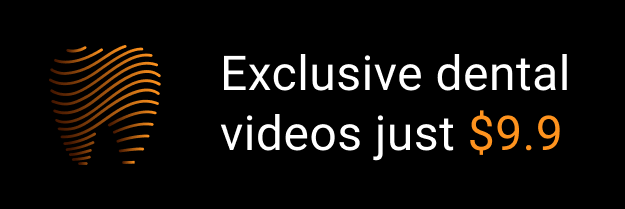