The pathogenicity of the dental biofilm is modified by salivary and dietary factors, as well as by the characteristics of the tooth structure. The composition of the acquired pellicle can modify the mineral homeostasis of the tooth surfaces and the attachment of bacteria for the development of the biofilm. The substitution of sucrose from the diet by other less cariogenic sugars and/or sugar substitutes can contribute to reducing the pathogenicity of the biofilm. Saliva clears, dilutes, neutralizes, and buffers acids produced by the biofilm. In addition, saliva provides the biofilm/tooth structure with Ca 2+ PO 4 3– and F – ions, which can positively affect the equilibrium between demineralization-remineralization toward the remineralization and modify the susceptibility of the tooth structure to caries progression.
An important starting place when discussing the caries environment is to accept that it is only the biofilm-covered tooth surfaces that have the potential to develop caries lesions. The multifaceted nature of the caries process makes it somewhat difficult to discuss any individual component in isolation of the other major etiologic factor, namely the dental biofilm. Thus, this article relates how saliva, the acquired pellicle, the diet, and the tooth structure itself interact and modify the pathogenicity of the dental biofilm, which is not necessarily pathogenic (see the article by Philip D. Marsh elsewhere in this issue for further exploration of this topic).
Saliva
It is the saliva surrounding the tooth that creates the milieu that bathes the tooth surface and serves as the main vehicle for solubilizing and transporting potential harmful substances as well as protective factors to the biofilm-covered tooth surface. The saliva flow rate and composition are well recognized as important host factors that modify the caries process. Saliva’s protective role is mediated by its ability to clear cariogenic food substances from the mouth; dilute, neutralize, and buffer organic acids formed by biofilm microorganisms; and reduce the demineralization rate and enhance remineralization by providing calcium, phosphate, and fluoride in the fluid phase of the biofilm in close association with the tooth surface. In that sense, aspects increasing the salivary flow rate, such as mechanical and chemical stimuli, are relevant modulating factors potentially increasing protection against caries lesion development. Mastication can stimulate the saliva output. It has been suggested that stimulation of the mechanoreceptive neurons in the gingival tissues may result in a reflex secretion of saliva. Chewing gum has proved to be an effective way to mechanically increase salivary flow. Clinical studies have been able to correlate sugarless gum use with lower levels of caries lesions. Although no clear association has been observed with the presence of specific ingredients of the gum (ie, sugar substitutes such as sorbitol or xylitol), a rather evident benefit seems to be related to the mechanical stimulation of saliva by the chewing process. Chemical stimulation from acidic food has been shown to significantly increase salivary flow. Depending on the stimuli, different salivary glands may be affected leading to the variation in salivary flow and composition, thus influencing the level of salivary protection.
Higher salivary flow allows for increased availability of organic and inorganic constituents of saliva. The inorganic constituents of interest are calcium (Ca 2+ ), phosphate (PO 4 – ), fluoride (F – ), carbonic acid (H 2 CO 3 )/hydrogen carbonate (HCO 3 – ), and di-hydrogenphosphate (H 2 PO 4 – )/hydrogenphosphate (HPO 4 2– ). These ions are associated with the maintenance of the integrity of teeth by regulating the demineralization/remineralization processes and the buffer capacity of saliva. Although the benefits of calcium phosphate and fluoride have been well established, the influence of salivary buffer capacity in the prevention of caries is not well defined and has sometimes been questioned. However, it has been reported that the concentration of hydrogen carbonate, the main buffer component of saliva, increases about 12 times from unstimulated to stimulated whole saliva. Therefore, the salivary buffer capacity can be considered as a potential contributing factor in the reduction of enamel demineralization, although its effect may, sometimes, be overshadowed by several other caries risk factors such as oral hygiene, presence of cariogenic bacterial strains, sugar consumption, and fluoride. Thicker biofilms that form in caries-prone tooth surfaces may limit the ability of saliva to exert its protective effect in combination with the interactions that occur between cariogenic bacteria and diet.
Increase of salivary flow has a direct impact on the clearance of sugar and acids from the tooth surface, which implicates the onset and progression of dental caries lesions. Once secreted into the mouth, saliva will form a thin film that interacts with substances present on the teeth and/or mucosa surfaces that will be ultimately removed from the oral cavity by swallowing. The rate of oral clearance was shown to vary markedly at different locations in the mouth. Oral clearance is slower for upper teeth compared with lower and for the buccal surfaces compared with lingual. The slowest clearance rate is on the buccal surface of the upper anterior teeth, whereas the fastest is on the lingual surfaces of lower anterior teeth. This can be explained by the proximity of those surfaces in relation to the submandibular and sublingual glands. More recently, the importance of the location of the parotid duct orifice was also shown to be important for the clearance rate of buccal surfaces of upper molars.
If the increase of salivary flow benefits caries prevention, the loss of salivary function has shown to be detrimental and can be associated with the development of rampant caries lesions. Salivary flow impairment can be related to aging even though some other studies have not found this correlation. It is well established that patients taking medication can also present decreased saliva output, as well as those who have received radiation therapy for neck and head cancer. Tests of the stimulated and unstimulated flow rate as well as of the buffer capacity of saliva may provide useful information about the susceptibility of an individual to dental caries. It is suggested that sialometric evaluations be carried at a fixed time point or in a limited time interval in the morning, avoiding intraindividual variations owing to the circadian cycle.
The organic phase of saliva contains antimicrobial agents, such as secretory immunoglobulin A (IgA), lysozyme, lactoferrin, and peroxidases that may be related to caries. Lysozyme can lead to bacteriolysis by disrupting bacterial cell walls. Lactoferrin binds iron, interfering with bacterial growth by both iron-dependent and -independent mechanisms. Lactoperoxidase can oxidize bacterial sulfhydryl groups, thus inhibiting glucose metabolism. This peroxidase protects salivary glycoproteins from degradation by bacteria. Salivary IgA can react with Streptococcus mutans; consequently, it may have implications on the prevention of dental caries. Studies have suggested that saliva of caries-free patients contains higher levels of IgA antibody against many S mutans epitopes than the saliva of caries-susceptible patients ; however, the strength of these relationships may not be adequate to have clinically useful predictive value.
The organic phase of saliva is also constituted by proteins that may regulate the demineralization and remineralization processes involved in caries development and arrest. Statherins, proline-rich proteins (PRPs), histatins, and cystatins have shown high affinity to enamel surfaces and are potentially involved with the maintenance of tooth integrity by supporting a suitable calcium phosphate environment. Thus, it is suggested that those salivary proteins can provide protection against demineralization. Up to this date, the literature is scarce showing how this interaction occurs in clinically relevant conditions and what would be the net result in terms of demineralization and remineralization of the dental structures. Mandel and colleagues and Mandel and Bennick could not observe differences between caries-active and caries-resistant individuals when analyzing parotid saliva proteins and acidic PRPs, respectively. Conversely, Balekjian and colleagues found that rampant caries was associated with the reduction in the proportion of basic proteins and increase in amylase. Vitorino and colleagues, using mass spectrometry approaches, showed a strong correlation between presence of phosphoproteins (PRP1, PRP3, histatin 1, and statherin) and absence of caries, suggesting that large amounts of phosphoproteins could contribute to more effective remineralization processes. More recently, Rudney and colleagues similarly identified not only statherin but also cystatin s-(AA1-8) variant as predictors of occlusal caries. Higher levels of these proteins seemed to be associated with higher rates of remineralization. Although the above-mentioned studies may suggest some relevance of the salivary proteins in the maintenance of tooth surface integrity, results are still inconclusive. Dodds and colleagues have suggested that not only the proteins but also their biologic activity are relevant for the development of caries lesions. In addition, limitations on the experimental design may interfere with the results. More research is needed to verify how those, and potentially other salivary proteins, can modify the caries process.
Considering the importance of saliva in the caries process, attempts have been made to evaluate its properties and composition and use them to predict the development of caries. For instance, clinical tests and tool kits have been developed to evaluate the salivary flow (unstimulated and stimulated) and the inorganic phase of saliva, including its pH, buffer capacity, and Ca 2+ , PO 4 3– , and F – ion concentrations. Similarly, it has been suggested that salivary proteins could be used as key biomarkers for several systemic diseases, including dental caries. The collection of information on the salivary factors may be of great help in determining the individual risk for caries development, but additional validation is required.
Acquired pellicle
The acquired pellicle is an acellular, bacteria-free organic film that is deposited on teeth, occupying a critical position between the enamel surface and dental biofilm. The pellicle is formed mainly by the salivary glycoproteins and proteins from different sources, including saliva, bacterial components or products, gingival crevicular fluid, blood, food, and enamel fluid. These select organic components have a high affinity for the enamel surface and rapidly adsorb to a clean (after tooth brushing with dentifrice, chemical dissolution, or prophylaxis) enamel surface. The pellicle formation process appears to involve a 2-step process. Initially, there is adsorption of discrete proteins to the hydrophobic regions of the tooth by electrostatic interactions, leaving hydrophobic parts of the protein molecules exposed at the surface. Following, protein aggregates or micellelike structures may adsorb to uncovered sites on the tooth surface and also interact with the initially formed hydrophobic protein layer. This specific adsorption pattern seems to be responsible for the globular morphology of the acquired pellicle.
This organic layer becomes detectable on dental surfaces after a few minutes of exposure to the oral environment. Biologic, ie, enzymatic, activity is also detectable on early stages of pellicle formation. It is suggested that it grows until reaching equilibrium between protein adsorption and de-sorption within 2 hours. Even if the pellicle may reach its full thickness in 2 hours, there is a continuing maturation process, which modifies the pellicle characteristics as a diffusion barrier to ionic conductivity on the enamel surface. In in vitro conditions, this maturation process was suggested to take at least 4 days, whereas about 18 hours was needed in in vivo conditions. It seems that enzymes, possibly transglutaminase, may account for this difference. Transglutaminase is continuously resupplied in the mouth but, because of its short half-life, rapidly loses its activity in vitro. Therefore, enzyme activity promotes a structural remodeling of the acquired pellicle during the maturation process, which seems to be important for stabilizing and creating a more acid-resistant pellicle. Extraoral factors may also modify pellicle formation. The regular use of abrasive toothpastes, whitening products, intake of acidic foods and beverages, and the frequent abrasion of teeth during professional cleaning inhibit pellicle formation and maturation. This may increase the susceptibility of enamel to dental erosion and potentially modify the caries susceptibility of a tooth surface.
Similar to saliva, the acquired enamel pellicle is constituted of proteins such as albumin, mucin, acidic PRPs, and cystatins that have shown to be important contributors to the protection of enamel tissues from acid-induced demineralization. The acquired pellicle can act not only as a physical barrier preventing acid diffusion, but also as a reservoir of remineralizing electrolytes (Ca 2+ , PO 4 3– , and F – ). Recent developments of sensitive proteomic methodologies have opened new avenues for the characterization of very low abundance biologic samples. Using this proteomic technology, more than 130 different proteins have been identified in the dental pellicle ; however, there is no clear understanding of their roles in dental caries development. It is known that those proteins can modulate the mineral homeostasis of tooth surfaces and the attachment of the bacteria that constitute the oral biofilm.
The thickness of the acquired enamel pellicle is reported to be between 0.1 and 1.0 μm. Besides acid demineralization protection, the pellicle serves as a lubricant between teeth and soft tissues and other structures, allowing free movement. More importantly for the current topic, the pellicle also provides a base for the subsequent development of dental biofilm. Early colonizing bacteria derived from the saliva passively adhere to this pellicle. In this process, statherin and PRPs seem to have active roles, as they have anchoring receptors that allow microorganisms to attach firmly to their surfaces by electrostatic, hydrophobic ionic, and van der Waals forces. The maturation of the dental biofilm as well as its pathogenicity is further described in the article by Philip D. Marsh elsewhere in this issue.
Acquired pellicle
The acquired pellicle is an acellular, bacteria-free organic film that is deposited on teeth, occupying a critical position between the enamel surface and dental biofilm. The pellicle is formed mainly by the salivary glycoproteins and proteins from different sources, including saliva, bacterial components or products, gingival crevicular fluid, blood, food, and enamel fluid. These select organic components have a high affinity for the enamel surface and rapidly adsorb to a clean (after tooth brushing with dentifrice, chemical dissolution, or prophylaxis) enamel surface. The pellicle formation process appears to involve a 2-step process. Initially, there is adsorption of discrete proteins to the hydrophobic regions of the tooth by electrostatic interactions, leaving hydrophobic parts of the protein molecules exposed at the surface. Following, protein aggregates or micellelike structures may adsorb to uncovered sites on the tooth surface and also interact with the initially formed hydrophobic protein layer. This specific adsorption pattern seems to be responsible for the globular morphology of the acquired pellicle.
This organic layer becomes detectable on dental surfaces after a few minutes of exposure to the oral environment. Biologic, ie, enzymatic, activity is also detectable on early stages of pellicle formation. It is suggested that it grows until reaching equilibrium between protein adsorption and de-sorption within 2 hours. Even if the pellicle may reach its full thickness in 2 hours, there is a continuing maturation process, which modifies the pellicle characteristics as a diffusion barrier to ionic conductivity on the enamel surface. In in vitro conditions, this maturation process was suggested to take at least 4 days, whereas about 18 hours was needed in in vivo conditions. It seems that enzymes, possibly transglutaminase, may account for this difference. Transglutaminase is continuously resupplied in the mouth but, because of its short half-life, rapidly loses its activity in vitro. Therefore, enzyme activity promotes a structural remodeling of the acquired pellicle during the maturation process, which seems to be important for stabilizing and creating a more acid-resistant pellicle. Extraoral factors may also modify pellicle formation. The regular use of abrasive toothpastes, whitening products, intake of acidic foods and beverages, and the frequent abrasion of teeth during professional cleaning inhibit pellicle formation and maturation. This may increase the susceptibility of enamel to dental erosion and potentially modify the caries susceptibility of a tooth surface.
Similar to saliva, the acquired enamel pellicle is constituted of proteins such as albumin, mucin, acidic PRPs, and cystatins that have shown to be important contributors to the protection of enamel tissues from acid-induced demineralization. The acquired pellicle can act not only as a physical barrier preventing acid diffusion, but also as a reservoir of remineralizing electrolytes (Ca 2+ , PO 4 3– , and F – ). Recent developments of sensitive proteomic methodologies have opened new avenues for the characterization of very low abundance biologic samples. Using this proteomic technology, more than 130 different proteins have been identified in the dental pellicle ; however, there is no clear understanding of their roles in dental caries development. It is known that those proteins can modulate the mineral homeostasis of tooth surfaces and the attachment of the bacteria that constitute the oral biofilm.
The thickness of the acquired enamel pellicle is reported to be between 0.1 and 1.0 μm. Besides acid demineralization protection, the pellicle serves as a lubricant between teeth and soft tissues and other structures, allowing free movement. More importantly for the current topic, the pellicle also provides a base for the subsequent development of dental biofilm. Early colonizing bacteria derived from the saliva passively adhere to this pellicle. In this process, statherin and PRPs seem to have active roles, as they have anchoring receptors that allow microorganisms to attach firmly to their surfaces by electrostatic, hydrophobic ionic, and van der Waals forces. The maturation of the dental biofilm as well as its pathogenicity is further described in the article by Philip D. Marsh elsewhere in this issue.
Diet
Dental caries has long been associated with the frequency of fermentable carbohydrate intake. Changes in dietary patterns and the increase in the use of fluoride has modified this relationship, as discussed later; however, from an etiology basis, diet still remains the main driver of the caries process. In that aspect, considerations on the retentiveness of the food, the presence of protective factors in foods (calcium, phosphate, and fluoride), and the type of carbohydrate are still important. Relevant carbohydrates can be divided into complex and simple. Complex carbohydrates (starches) are considered less cariogenic because starches are not readily soluble in oral fluids and have a low diffusion rate in biofilm. They also must be broken down to maltose by salivary amylase before biofilm bacteria can metabolize them; however, most starch is cleared from the mouth before it can be broken down. Simple sugars (sucrose, glucose, fructose) are more cariogenic, with sucrose being possibly the most; therefore, it has been implicated as an important determinant of dental caries disease.
Sucrose represents the main source of sugar in the diet. In studies in rats, using an experimental caries model, sucrose was shown to be more cariogenic than other dietary sugars such as glucose, fructose, and lactose. This cariogenic effect appears to be strain-specific, and is also influenced by the type of animal model and class of caries. The cariogenic properties of sucrose may be explained by 2 main processes. First, sucrose is freely diffusible in dental plaque and readily metabolized by oral bacteria, leading to the production of organic acids in sufficient concentration to lower the pH of dental plaque enough to allow enamel demineralization to occur. Second, sucrose is involved in the synthesis of soluble and insoluble extracellular glucan. The enzymes responsible for the synthesis of extracellular glucans and fructans (glucosyltransferases and fructosyltransferases, respectively) have a high affinity for sucrose. Although the first property is shared among simple sugars, the second is specifically related to sucrose only.
The synthesis of extracellular polysaccharides can favor the accumulation of S mutans and other cariogenic bacteria in dental biofilm. This unique property of sucrose has been shown in previous studies using an intraoral caries model, where markedly enhanced demineralization was associated with S mutans test plaques prepared from sucrose-containing cultures compared with glucose-grown plaques. This was attributed to an alteration of the diffusion properties of plaque owing to the presence of water-insoluble extracellular matrix material (glucan) synthesized from sucrose. The extracellular matrix permits greater penetration of dietary carbohydrates into the deeper layer of dental biofilm adjacent to the tooth surface, which from a caries lesion point of view is the only layer that counts.
It is important to keep in mind that the relationship of diet with caries disease has changed. All historical and epidemiologic evidence before 1970 clearly associated the availability of sugar and refined carbohydrates with increased caries disease prevalence. Caries disease increased in severity as the standard of living and nutrition improved. From the early 1970s until the most recent (1999–2004) US National Health and Nutrition Examination Survey, a change has been observed in that there has been a reduction in the caries prevalence in adolescents (age 12 to 19), adults (age 20 to 64), and seniors (age 65 and older). There was, however, a small but significant increase in caries in the primary teeth of children (age 2–11). Although no clear change has been observed in total sugar consumption over the past 30 years, there has been a change in the form of sugar use, with reduction of sucrose and increase of fructose use, mainly in the form of high-fructose corn syrup. Although the reduction in caries prevalence has been generally attributed to topical effects of fluoride, the reduction in sucrose consumption and replacement with other simple sugars may also be a contributing factor.
Individual guidance on dietary changes should be provided by dental care practitioners. This includes the assessment of the patient’s dietary habits with analyses of the sugar exposure patterns and type of foods. This information can be obtained through the use of dietary diaries and also by recall interviews. Diet counseling should focus not only on reducing the exposures to sugar but also on providing recommendations of healthier alternatives.
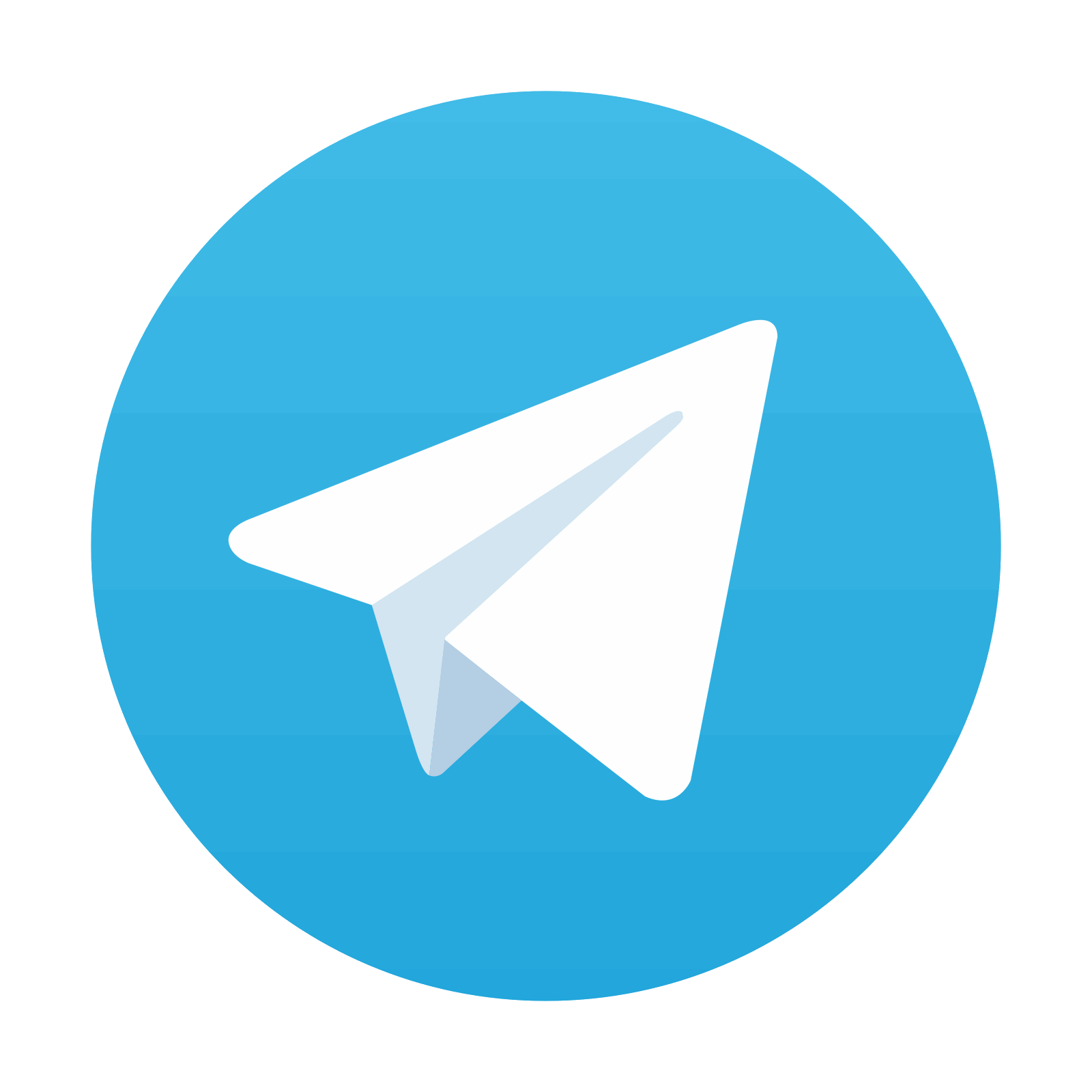
Stay updated, free dental videos. Join our Telegram channel

VIDEdental - Online dental courses
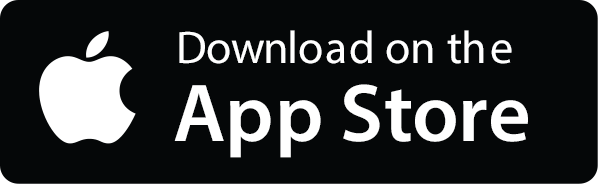
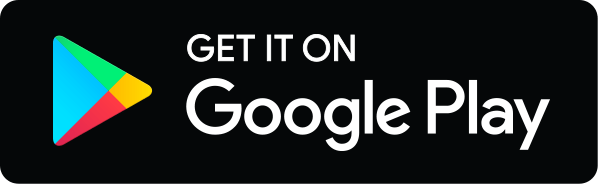