Abstract
Objectives
Interaction between osteogenesis and angiogenesis plays an important role in implant osseointegration. In the present study we investigated the influence of titanium surface properties on the angiogenic behaviors of endothelial cells grown in direct contact co-culture with osteoblasts.
Methods
Human umbilical vein endothelial cells (HUVECs) and osteoblast-like cells (MG-63 cells) were grown in direct co-culture on the following titanium surfaces: acid-etched (A), hydrophilic A (modA), coarse-gritblasted and acid-etched (SLA) and hydrophilic SLA (SLActive). Cell proliferation was evaluated by cell counting combined with flow cytometry. The expression of von Willebrand Factor (vWF), thrombomodulin (TM), endothelial cell protein C receptor (EPCR), E-Selectin, as well as vascular endothelial growth factor (VEGF) receptors Flt-1 and KDR in HUVECs and VEGF in MG-63 were measured by qPCR. The dynamic behavior of endothelial cells was recorded by time-lapse microscopy.
Results
Proliferation of HUVECs was highest on A, followed by SLA, modA and SLActive surfaces. The expression of vWF, TM, EPCR, E-Selectin and Flt-1 in HUVECs was significantly higher on A than on all other surfaces. The expression of KDR in HUVECs grown on A surface was below detection limit. VEGF expression in MG-63 cells was significantly higher on SLActive vs SLA and modA vs A surfaces. Time-lapse microscopy revealed that HUVECs moved quickest and formed cell clusters earlier on A surface, followed by SLA, modA and SLActive surface.
Conclusions
In co-culture conditions, proliferation and expression of angiogenesis associated genes in HUVECs are promoted by smooth hydrophobic Ti surface, which is in contrast to previous mono-culture studies.
1
Introduction
Wound healing following implantation is a complicated process which comprises a series of biological events, in which different cell types and regulation factors are involved . Titanium (Ti) implant surface characteristics, such as chemical composition, surface topography, roughness as well as surface energy are considered to have a substantial impact on the osseointegration process during initial wound healing after implantation . Angiogenesis, the process of new blood vessels formation, supports new bone formation by delivering prerequisite substances of bone reconstruction, such as nutrients, regulatory factors, oxygen, and osteoprogenitor cells. The dynamic balance and regulation between angiogenesis and osteogenesis is crucial for bone modeling and remodeling in wound healing after trauma, . Alteration of angiogenesis might also lead to a pathological situation of the bone tissue . Since angiogenesis plays an important role in osteogenesis through wound healing, studies of the last years focus on investigating the role of angiogenesis and the interaction between angiogenesis and osteogenesis in wound healing after implantation .
The investigation of cell growth and functional behavior on different Ti surfaces in in vitro models is important to understand the mechanisms underlying wound healing after implantation . Several previous studies used mono-cell culture to investigate the influence of Ti surface characteristics on osteoblasts and endothelial cells, as models for osteogenesis and angiogenesis, respectively. Osteoblasts grown on rougher and hydrophilic Ti surfaces exhibit increased differentiation and expression of osteogenesis markers . In contrast, studies of endothelial cells show that smooth surfaces are optimal for the proliferation of endothelial cells, whereas surfaces with high hydrophilicity promote the differentiation of endothelial cells . However, mono-culture studies have certain limitations, because they do not consider the interaction between different cell types. Particularly, the interaction between osteogenesis and angiogenesis, which plays a pivotal role in wound healing, cannot be assessed appropriately in studies with mono-culture cell models. These shortcomings could be overtaken by co-culture studies, which consider both intercellular paracrine signaling and direct intercellular communication, and therefore more exactly reflect complex in vivo processes . In our previous studies on co-culture of osteoblasts and endothelial cells, we found that differentiation and the expression of osteogenic phenotype are enhanced by hydrophilic and rough SLActive surface . Studies with mono-culture model also show that rough hydrophilic SLActive surface stimulates the release of the vascular endothelial growth factor (VEGF) by osteoblasts, which in turn might promote endothelial cells differentiation and angiogenesis . However, the behavior of endothelial cells under condition of simultaneous growth with osteoblasts on Ti surfaces has not been investigated to date. Therefore, in the present study we investigated proliferation, migration, and expression of angiogenesis-related factors in endothelial cells grown in direct contact co-culture with osteoblasts on different Ti surfaces.
2
Materials and methods
2.1
Preparation of Ti surfaces
Cylindrical shaped Ti disks with a diameter of 15 mm and a thickness 1 mm were prepared from grade 2 unalloyed commercially pure Ti and supplied by Institut Straumann AG (Basel, Switzerland). Four kinds of Ti surfaces with different roughness and hydrophilicity were treated as described in our previous study : acid-etched surfaces (A), hydrophilic A surfaces (modA), coarse-grit blasted and acid-etched surfaces (SLA) and hydrophilic SLA surfaces (SLActive, in former publications called modSLA). Shortly, A surface was prepared by acid-etching in hydrochloric acid/sulfuric acid according to a proprietary process of Institut Straumann AG. SLA surface was prepared by sandblasting with 0.25–0.5 mm corundum and subsequent acid etching in hydrochloric acid/sulfuric acid according to a proprietary process of Institut Straumann AG. The modA and SLActive surfaces were prepared by rinsing the A and SLA surfaces under nitrogen protection and storing the samples in isotonic saline solution, respectively. The surfaces SLA and SLActive correspond to the commercially available SLA ® and SLActive ® (both Institut Straumann AG) implant surfaces. The methods of roughness measurements as well as parameters describing roughness of investigated Ti surfaces are given in our previous study . In this study no characteristic differences in the micro roughness values between A vs modA as well as SLA vs SLActive were observed. Different roughness parameters of some surfaces used in the present study are mentioned in the recent review paper . Here, higher values of the developed surface area S dr in SLActive compared to those of SLA are reported, which could be attributed to the presence of nanostructures on SLActive surface.
2.2
Cell culture
Commercially available human umbilical vein endothelial cells (HUVECs, Technoclone, Vienna, Austria) were cultured in endothelial cell growth medium (ECM, Technoclone, Vienna, Austria) supplemented with 100 U/ml penicillin, 100 μg/ml streptomycin, 0.25 μg/ml fungizone, 2 mM l -glutamine, 5 U/ml heparin, 30–50 μg/ml endothelial cell growth supplement, and 20% fetal calf serum (FCS). Osteoblast-like cells MG-63 (American Type Culture Collection, Rockville, USA) were cultured in modified Eagle’s minimum essential medium (Gibco ® , Invitrogen, Carlsbad, USA) supplemented with 10% fetal bovine serum, streptomycin (50 μg/ml), and penicillin (100 U/ml). Both HUVECs and MG63 were grown in a humidified atmosphere containing 5% CO 2 at 37 °C. Cells from the passage 4–7 in culture were used in this study.
2.3
Cell co-culture of HUVECs with MG63s
HUVECs were seeded on different Ti surfaces in 24 well plates at an initial density of 0.67 × 10 5 cells per well in 300 μl of ECM. After 24 h, 200 μl of ECM containing 0.33 × 10 5 MG-63 cells was added into each well. Cells seeded with the same protocol on tissue culture plastic surface served as a control. Each experimental group included six different wells. The choice of the experimental conditions was made based on the following criteria: first, both cell types must proliferate during the experiments; second, no extreme prevalence of one cell type should be observed. Approximately similar amount of both cell types during the experiments are required to assure the interaction between different cell types. We performed co-culture experiments in ECM, because the growth of endothelial cells was strikingly inhibited by culturing in MEM medium, which is preferable for MG-63 cells (data not shown). The choice of initial ratio 2:1 between HUVECs and MG-63 cells was determined by the fact, that the proliferation rate of MG-63 is higher than that of HUVECs (data not shown). At these initial conditions, we did not have extreme prevalence of MG-63 over HUVECs during the experiment.
2.4
Cell proliferation
Proliferation of HUVECs and MG-63 cells in co-culture was evaluated by manual cell counting combined with flow cytometry similarly to the method described in our previous studies . Shortly, after 24 or 48 h of co-culture the cells were collected and the total cell number in the suspension was determined by manual cell counting. Afterwards, cells were stained with fluorescein isothiocyanate (FITC)-conjugated anti-CD31 antibody and the proportion of different cell types was measured by flow cytometry (FACSCalibur, Becton Dickinson, CA, USA). Each sample was acquired three times; each acquisition was limited to 20,000 events. Data were analyzed using Cell Quest 3.3 software. CD31-positive cells were considered as HUVECs, CD31-negative cells were considered as MG-63 cells. The number of each individual cell type was calculated by multiplying the total cell number and the proportion of this cell type in the suspension.
2.5
Real-time PCR
The expression of various angiogenesis-related genes in HUVECS was measured by real time polymerase chain reaction (PCR) after 48 h of co-culture. Before PCR, different cells types were separated by fluorescence-activated cell sorting as described in our previous studies . Shortly, cells were collected and stained with FITC-conjugated anti-CD31 antibody. Mixed cell samples were sorted using flow cytometry in the exclusion mode: HUVECs and MG63 were gated in the CD31-positive and CD31 negative cell quadrants, respectively. Cells were collected into sorting tubes coated with 4% BSA. The number of sorting events was 50,000 for each sample. Cells from control groups were also passed through similar staining and sorting procedures. After collection, the total cellular mRNA was isolated and transcribed into complementary DNA (cDNA) using TaqMan ® Gene Expression Cells-to-CT™ kit (Ambion/Applied Biosystems, CA, USA) according to the manufacturer’s instruction. Real time PCR was performed on an Applied Biosystems Step One Plus real time PCR system (Applied Biosystems, CA, USA) using the Taqman ® gene expression assays with the following primers (all from Applied Biosystems, CA, USA): Von Willebrand Factor (vWF), Hs00169795_m1; thrombomodulin (TM), Hs00264920_s1; endothelial cell protein C receptor (EPCR), Hs00941182_m1; E-Selectin (E-selectin), Hs00950401_m1; VEGF receptor 1 (Flt-1), Hs01052961_m1; VEGF receptor 2 (KDR), Hs00911700_m1; VEGF, Hs00900055_m1; β-actin (ACTB), Hs99999903_m1; glyceraldehyde-3-phosphate dehydrogenase (GAPDH), Hs99999905_m1. GAPDH and β-actin were used as house-keeping genes in HUVECs and MG-63, respectively. Triplicate real-time PCR reactions were performed at the following thermocycling conditions: 95 °C for 10 min, 50 cycles, each 95 °C for 15 s and 60 °C for 1 min. The point at which the PCR product was first detected above a fixed threshold (termed cycle threshold, C t ) was determined for each sample. Changes in the expression of target genes were calculated using <SPAN role=presentation tabIndex=0 id=MathJax-Element-1-Frame class=MathJax style="POSITION: relative" data-mathml='2−ΔΔCt’>2−ΔΔCt2−ΔΔCt
2 − Δ Δ C t
, where ΔΔ C t = ( C t target − C t GAPDH/β-actin ) sample − ( C t target − C t GAPDH/β-actin ) control .
2.6
Time-lapse microscopy
HUVECs dynamic behaviors were evaluated by time-lapse photography similar to the method described in our previous studies . HUVECs were stained with CellTracker™ Orange CMRA (Molecular Probes™, Invitrogen, UK) and seeded together with MG-63 cells on different Ti surfaces at a density of 1 × 10 5 cells and a ratio of 5:1 (HUVECs:MG-63) in 800 μl ECM medium. The HUVECs were observed dynamically under an upright fluorescence microscope (Nikon Eclipse E 800M microscope; Nikon Instruments Europe B.V., Badhoevedorp, The Netherlands). Fluorescently labeled HUVECs were photographed with a digital imaging system (Photometrics1 Cascade 512F, Germany) every 30 min for over 120 h and cell behaviors were recorded by making films using NIS-Elements software (Laboratory imaging, Prague, Czech Republic). Experiments with each kind of Ti surfaces were repeated at least three times.
2.7
Statistical analysis
The data are presented as mean ± SD of three independent experiments. ANOVA with Tukey’s HSD analysis was used to assess the difference between individual groups. P < 0.05 was considered to be statistically significant. All statistical analyses were performed using SPSS 17.0 (SPSS, Chicago, IL, USA).
2
Materials and methods
2.1
Preparation of Ti surfaces
Cylindrical shaped Ti disks with a diameter of 15 mm and a thickness 1 mm were prepared from grade 2 unalloyed commercially pure Ti and supplied by Institut Straumann AG (Basel, Switzerland). Four kinds of Ti surfaces with different roughness and hydrophilicity were treated as described in our previous study : acid-etched surfaces (A), hydrophilic A surfaces (modA), coarse-grit blasted and acid-etched surfaces (SLA) and hydrophilic SLA surfaces (SLActive, in former publications called modSLA). Shortly, A surface was prepared by acid-etching in hydrochloric acid/sulfuric acid according to a proprietary process of Institut Straumann AG. SLA surface was prepared by sandblasting with 0.25–0.5 mm corundum and subsequent acid etching in hydrochloric acid/sulfuric acid according to a proprietary process of Institut Straumann AG. The modA and SLActive surfaces were prepared by rinsing the A and SLA surfaces under nitrogen protection and storing the samples in isotonic saline solution, respectively. The surfaces SLA and SLActive correspond to the commercially available SLA ® and SLActive ® (both Institut Straumann AG) implant surfaces. The methods of roughness measurements as well as parameters describing roughness of investigated Ti surfaces are given in our previous study . In this study no characteristic differences in the micro roughness values between A vs modA as well as SLA vs SLActive were observed. Different roughness parameters of some surfaces used in the present study are mentioned in the recent review paper . Here, higher values of the developed surface area S dr in SLActive compared to those of SLA are reported, which could be attributed to the presence of nanostructures on SLActive surface.
2.2
Cell culture
Commercially available human umbilical vein endothelial cells (HUVECs, Technoclone, Vienna, Austria) were cultured in endothelial cell growth medium (ECM, Technoclone, Vienna, Austria) supplemented with 100 U/ml penicillin, 100 μg/ml streptomycin, 0.25 μg/ml fungizone, 2 mM l -glutamine, 5 U/ml heparin, 30–50 μg/ml endothelial cell growth supplement, and 20% fetal calf serum (FCS). Osteoblast-like cells MG-63 (American Type Culture Collection, Rockville, USA) were cultured in modified Eagle’s minimum essential medium (Gibco ® , Invitrogen, Carlsbad, USA) supplemented with 10% fetal bovine serum, streptomycin (50 μg/ml), and penicillin (100 U/ml). Both HUVECs and MG63 were grown in a humidified atmosphere containing 5% CO 2 at 37 °C. Cells from the passage 4–7 in culture were used in this study.
2.3
Cell co-culture of HUVECs with MG63s
HUVECs were seeded on different Ti surfaces in 24 well plates at an initial density of 0.67 × 10 5 cells per well in 300 μl of ECM. After 24 h, 200 μl of ECM containing 0.33 × 10 5 MG-63 cells was added into each well. Cells seeded with the same protocol on tissue culture plastic surface served as a control. Each experimental group included six different wells. The choice of the experimental conditions was made based on the following criteria: first, both cell types must proliferate during the experiments; second, no extreme prevalence of one cell type should be observed. Approximately similar amount of both cell types during the experiments are required to assure the interaction between different cell types. We performed co-culture experiments in ECM, because the growth of endothelial cells was strikingly inhibited by culturing in MEM medium, which is preferable for MG-63 cells (data not shown). The choice of initial ratio 2:1 between HUVECs and MG-63 cells was determined by the fact, that the proliferation rate of MG-63 is higher than that of HUVECs (data not shown). At these initial conditions, we did not have extreme prevalence of MG-63 over HUVECs during the experiment.
2.4
Cell proliferation
Proliferation of HUVECs and MG-63 cells in co-culture was evaluated by manual cell counting combined with flow cytometry similarly to the method described in our previous studies . Shortly, after 24 or 48 h of co-culture the cells were collected and the total cell number in the suspension was determined by manual cell counting. Afterwards, cells were stained with fluorescein isothiocyanate (FITC)-conjugated anti-CD31 antibody and the proportion of different cell types was measured by flow cytometry (FACSCalibur, Becton Dickinson, CA, USA). Each sample was acquired three times; each acquisition was limited to 20,000 events. Data were analyzed using Cell Quest 3.3 software. CD31-positive cells were considered as HUVECs, CD31-negative cells were considered as MG-63 cells. The number of each individual cell type was calculated by multiplying the total cell number and the proportion of this cell type in the suspension.
2.5
Real-time PCR
The expression of various angiogenesis-related genes in HUVECS was measured by real time polymerase chain reaction (PCR) after 48 h of co-culture. Before PCR, different cells types were separated by fluorescence-activated cell sorting as described in our previous studies . Shortly, cells were collected and stained with FITC-conjugated anti-CD31 antibody. Mixed cell samples were sorted using flow cytometry in the exclusion mode: HUVECs and MG63 were gated in the CD31-positive and CD31 negative cell quadrants, respectively. Cells were collected into sorting tubes coated with 4% BSA. The number of sorting events was 50,000 for each sample. Cells from control groups were also passed through similar staining and sorting procedures. After collection, the total cellular mRNA was isolated and transcribed into complementary DNA (cDNA) using TaqMan ® Gene Expression Cells-to-CT™ kit (Ambion/Applied Biosystems, CA, USA) according to the manufacturer’s instruction. Real time PCR was performed on an Applied Biosystems Step One Plus real time PCR system (Applied Biosystems, CA, USA) using the Taqman ® gene expression assays with the following primers (all from Applied Biosystems, CA, USA): Von Willebrand Factor (vWF), Hs00169795_m1; thrombomodulin (TM), Hs00264920_s1; endothelial cell protein C receptor (EPCR), Hs00941182_m1; E-Selectin (E-selectin), Hs00950401_m1; VEGF receptor 1 (Flt-1), Hs01052961_m1; VEGF receptor 2 (KDR), Hs00911700_m1; VEGF, Hs00900055_m1; β-actin (ACTB), Hs99999903_m1; glyceraldehyde-3-phosphate dehydrogenase (GAPDH), Hs99999905_m1. GAPDH and β-actin were used as house-keeping genes in HUVECs and MG-63, respectively. Triplicate real-time PCR reactions were performed at the following thermocycling conditions: 95 °C for 10 min, 50 cycles, each 95 °C for 15 s and 60 °C for 1 min. The point at which the PCR product was first detected above a fixed threshold (termed cycle threshold, C t ) was determined for each sample. Changes in the expression of target genes were calculated using <SPAN role=presentation tabIndex=0 id=MathJax-Element-2-Frame class=MathJax style="POSITION: relative" data-mathml='2−ΔΔCt’>2−ΔΔCt2−ΔΔCt
2 − Δ Δ C t
, where ΔΔ C t = ( C t target − C t GAPDH/β-actin ) sample − ( C t target − C t GAPDH/β-actin ) control .
2.6
Time-lapse microscopy
HUVECs dynamic behaviors were evaluated by time-lapse photography similar to the method described in our previous studies . HUVECs were stained with CellTracker™ Orange CMRA (Molecular Probes™, Invitrogen, UK) and seeded together with MG-63 cells on different Ti surfaces at a density of 1 × 10 5 cells and a ratio of 5:1 (HUVECs:MG-63) in 800 μl ECM medium. The HUVECs were observed dynamically under an upright fluorescence microscope (Nikon Eclipse E 800M microscope; Nikon Instruments Europe B.V., Badhoevedorp, The Netherlands). Fluorescently labeled HUVECs were photographed with a digital imaging system (Photometrics1 Cascade 512F, Germany) every 30 min for over 120 h and cell behaviors were recorded by making films using NIS-Elements software (Laboratory imaging, Prague, Czech Republic). Experiments with each kind of Ti surfaces were repeated at least three times.
2.7
Statistical analysis
The data are presented as mean ± SD of three independent experiments. ANOVA with Tukey’s HSD analysis was used to assess the difference between individual groups. P < 0.05 was considered to be statistically significant. All statistical analyses were performed using SPSS 17.0 (SPSS, Chicago, IL, USA).
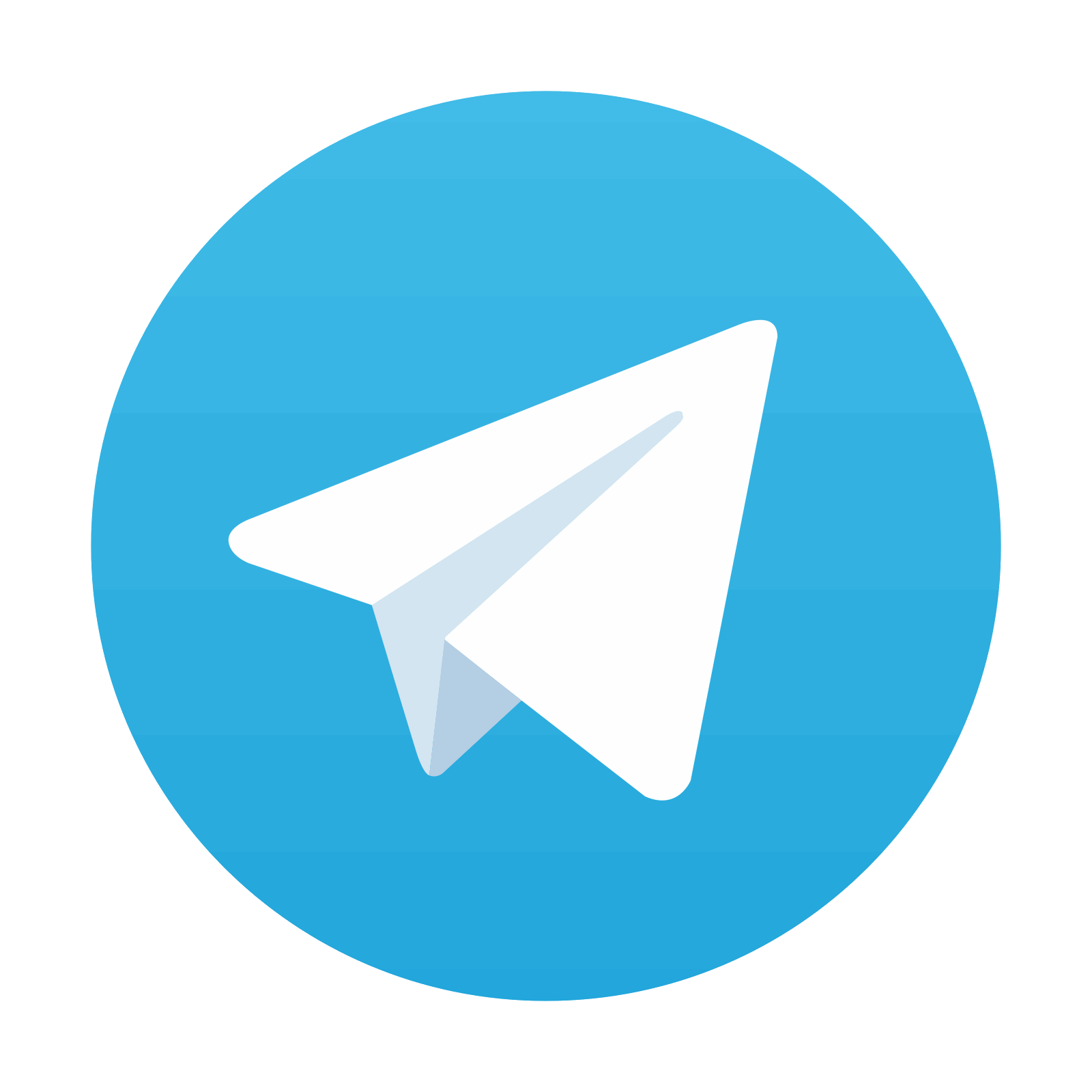
Stay updated, free dental videos. Join our Telegram channel

VIDEdental - Online dental courses
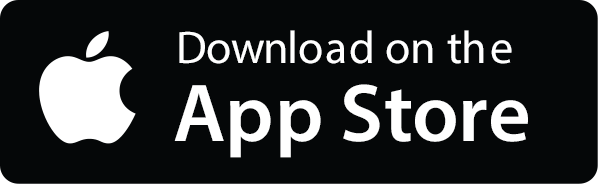
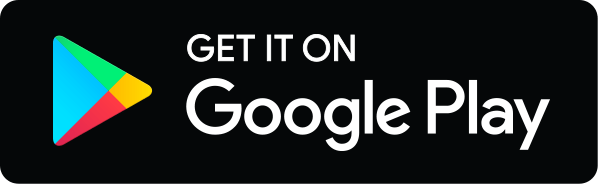