Abstract
Using polymethylmethacrylate (PMMA) cement in cemented endoprostheses may cause heat necrosis and prosthesis failure due to the highly exothermic reaction. This study determined the magnitude and duration of temperature change during the cementation of a mandibular endoprosthesis in an in vitro and in vivo Macaca fasicularis model. In the in vivo study the median maximum temperature ( T max ) around the mandible-prosthesis unit (MPU) was 31.0 °C with the peak T max at hole 1 (1 mm from stem). The in vitro study recorded a lower T max and indicated a trend that increased spacing (groove) around the prosthesis results in a higher T max . All the T max MPU measurements were lower than normal body temperature (38 °C). In the in vivo study the median maximum temperature change ( T maxΔ ) was 1.8 °C; in the vitro study, the T maxΔ of the 4 mm groove width was significantly higher than all others. Temperature increases were transient, with the temperature returning to baseline a median of 6.0 min after T max . Histological analysis showed surrounding tissue at the cement–bone interface with mild inflammation. Within the parameters tested, there was minimal risk of thermal damage. The temperature changes were influenced by the quantity of cement used and the distance from the prosthesis stem.
Polymethylmethacrylate (PMMA) was introduced in the medical field about 50 years ago for femoral head replication , cranioplasties , spine fixation and intracranial aneurysm . Sir J ohn C harnley of the University of Manchester is credited for leading the adaptation of PMMA for the cementation of an orthopaedic prosthesis in the long bones . There has been some reluctance in the medical community to accept PMMA cement. One of the reservations was the possibility of thermal necrosis of the surrounding bone as a result of the heat generated during the polymerization reaction of the cement, which can lead to loosening of the prosthesis and eventual failure of the implant .
PMMA cement is created through the combination of two primary components, a powder consisting of copolymers based on PMMA and a liquid monomer, methylmethacrylate (MMA). When the powder is mixed with the liquid, two processes occur. The combined absorption of the liquid by the powder and dissolution of the powder by the liquid creates a dough-like consistency. A chemical reaction called ‘addition polymerization’ takes place, resulting in hardening of the cement. The initiator in the powder, benzoyl peroxide, and the activator in the liquid, dimethyl-para-toluidine, interact in the initiation reaction to produce numerous free radicals, which interact with the vinyl double bonds of the MMA monomer to create polymerizable molecules. These form multiple active growing polymer chains by combining with more MMA molecules in the propagation phase. This rapid process continues until almost all the monomer has been converted to polymers. The reactions are terminated by the direct coupling of two active polymer chains or the transfer of a hydrogen atom from one active molecule to another. The polymerization ends when all the active molecules are eliminated.
The radical polymerization reaction is very exothermic, producing 57 kJ per 100 g of MMA . The maximum in vitro temperature during the polymerization reaction is 60–80 °C . The centre of the cement mantle reaches 67–124 °C , which is significantly higher than the denaturation temperature of collagen (56 °C). This increase in temperature may cause heat necrosis and subsequently aseptic loosening of the cemented prosthesis . The threshold for thermal tissue damage is also influenced by exposure time . L undskog indicated that bone that has been heated to 47 °C for 1 min will resorb and be replaced by fat . There is permanent disruption of blood flow and tissue necrosis of the bone at 60 °C . The in vivo polymerization temperature has been observed to be lower, probably due to a smaller quantity of cement, the cement being applied in thin layers, absorption and elimination by the blood circulation as well as the dissipation of the heat by the prosthesis and the surrounding tissue . R eckling and D illon showed that the mean maximum temperature was 48 °C with an increase of 3–17 °C , while H arving et al. recorded temperatures above 56 °C for 2–3 min . Animal studies indicated that the temperature increase did not induce any significant adverse effect . A study using finite element analysis predicted no thermal damage if the cement mantle was 3–5 mm thick . All these studies are based on orthopaedic joint replacement and may not be relevant for a mandibular endoprosthesis.
The aim of this study was to assess the magnitude and duration of the temperature changes around a mandibular endoprosthesis in an in vivo and in vitro study. The in vivo study focused on the effects of the polymerization reaction under natural conditions, while the in vitro study focused on the additional effect of the cement mantle thickness on the final temperature change. The in vitro study was also used to assess the temperature changes occurring in the stem of the prosthesis during the polymerization of the cement mantle.
The hypothesis was that the temperature changes around a PMMA cemented mandibular endoprosthesis are minor and unlikely to cause significant thermal damage to the surrounding tissues.
Materials and methods
In vivo study
Six pathogen-free young adult monkeys ( Macaca fascicularis ), about 4 years old, weighing approximately 3.5 kg, were used in this study. The monkeys had full dentition with partially erupted third molars. The study was accepted by the Institutional Animal Care and Use Committee of SingHealth, Singapore and the animal laboratory has been certified by the International Association for Assessment of Laboratory Animal Care.
The mandibular endoprostheses were made with help of skull models as explained in a previous study . They were made from titanium alloy (Ti–6A1–4V) with a dual etched outer surface (Biomet Microfixation, Jacksonville, USA) ( Fig. 1 ). The endoprosthesis is a 3-piece modular system . The height of the body of the prosthesis was 70% of the original height of the mandible, while the contour on the buccal, lingual and caudal aspects followed the original contour of the mandible. The stems were made to fit closely in the marrow space of the mandible. They were rounded off at the ends to reduce the shearing forces at the stem–cement interface. The implant body was 15 mm long and 12 mm high and the stems were 10 mm long, 7 mm high and 1 mm thick, with two holes to counteract rotational movements.

Before surgery, the animals were fasted overnight. They were given 0.05 mg/kg atropine s.c. preoperatively and were sedated with 10 mg/kg ketamine s.c.. All animals were weighed prior to surgery. Induction of anesthesia was performed by a veterinarian using 3% halothane. Endotracheal intubation was instituted with oral-tracheal tubes of gauge 3.5 mm. Anesthesia was maintained with 1–2% isoflurane. The animals were given 2 mg/kg carprofen (Rimadyl ® , Pfizer Inc., New York, USA) for analgesia and 5 mg/kg enrofloxacion (Baytril ® , Bayer Health Care, Leverkusen, Germany) and 15 mg/kg amoxycillin s.c. (Betamox ® , Norbrook Pharmaceuticals World Wide, Ireland) for antibiotic cover.
Preoperative lateral radiographs were taken of all animals, positioned with their right mandible facing the plate. The radiographs were taken using a Siemens POLYMOBIL Plus ® machine (Siemens Medical Solutions, Erlangen, Germany) set at 40 kV for 2 ms at a distance of 70 cm.
Surgery was carried out via an intra-oral approach beginning with two vertical incisions between the second bicuspid and first molar as well as behind the second molar. A horizontal incision, 2–3 mm below the attached gingiva, connected the two vertical incisions. The periosteum was reflected to expose the area of the planned resection. The resected segment contained the two permanent molars and included the attached gingiva on both sides.
The marrow space of the monkey mandible contains cancellous bone. In order to create space to accommodate the stem, a 9 mm high and 11 mm deep groove was made with a 12 mm long tapered fissure burr on the posterior (distal) and anterior (mesial) ends of the bone. This study assessed only the anterior stem cementation. The position of the stem was marked and three 0.5 mm wide holes were drilled through the buccal cortex ( Fig. 2 ) under copious irrigation. The temperature of the holes was allowed to stabilize and recorded as the baseline temperature. All temperature measurements were taken with a type k thermocouple (CIE TM-305 thermometer, Rapid-Tech Equipment, Knox City Centre, VIC, Australia), which has a sensitivity of 0.1 °C, accuracy of 0.3% and a 0.5 mm measurement tip. The prosthetic stem modules were fitted into the marrow space and cemented using Palacos ® cement (Biomet Inc., Warsaw, USA). The cement was mixed according to the manufacturer’s instructions. The cement was allowed to turn doughy before application into the created groove. The amount of cement used to fix the stem was calculated by using a pre-weighed piece of doughy cement to load the cavity and then subtracting all the excess cement. Separately, approximately 0.5 g of the cement was loaded in a plastic container as a control to register the polymerization reaction at room temperature. This specimen was named ‘cement block’. After seating the stems, temperature recording of the mandible prosthesis unit (MPU) with the thermocouple started. The temperature was measured at 4 MPU locations: mandibular surface, hole 1, hole 2 and hole 3 ( Fig. 3 ). The temperature readings were taken once every minute until all the areas had returned to the baseline temperature or had reached a stable level (3 consecutive unchanged readings). After the temperature recordings, the body of the endoprosthesis was connected to the cemented stem modules ( Fig. 4 ). The wounds were closed and the animals were returned to their housing .



After 3 months of implantation, the animals were killed and the mandibles were harvested for histological preparation. After fixation in 4% formalin, dehydration and embedding in MMA, three sections of each stem were made using a modified inner circular saw technique. The sections were stained with basic fuchsin/methylene blue and examined using a light microscope Olympus Microscope Model CX31 (Tokyo, Japan) and Olympus Soft Imaging Solution Cell A software (Münster, Germany) to determine the thickness of the cement mantle and the histological status of the tissue at the cement–bone interface. The tissue at the cement–bone interface was examined and graded on a scale adapted from J ansen et al. ( Table 1 ).
Reaction zone | Response | Score |
---|---|---|
Interface quality | Direct bone-to-cement contact without soft tissue interlayer | 4 |
Remodelling lacuna with osteoblasts and/or osteoclasts at surface | 3 | |
Localised fibrous tissue not arranged as a capsule | 2 | |
Fibrous tissue capsule | 1 | |
Inflammation | 0 | |
Inflammatory zone quantity | 0–4 cell layers | 4 |
5–9 cell layers | 3 | |
10–30 cell layers | 2 | |
>30 cell layers | 1 | |
Not applicable | 0 | |
Foreign body reaction zone quantity | 0 cell layers | 4 |
1–9 cell layers | 3 | |
10–60 cell layers | 2 | |
>60 cell layers | 1 | |
Not applicable | 0 | |
Foreign body reaction zone quality | Direct bone-to-cement contact without intervening tissue layer | 4 |
Reactive tissue is fibrous, showing fibroblasts and collagen | 3 | |
Reactive tissue is granulous, containing both fibroblasts and many inflammatory cells | 2 | |
Reactive tissue consists of masses of inflammatory cells with little or no signs of connective tissue organization | 1 | |
Cannot be evaluated because of infection or other factors not necessarily related to the material | 0 |
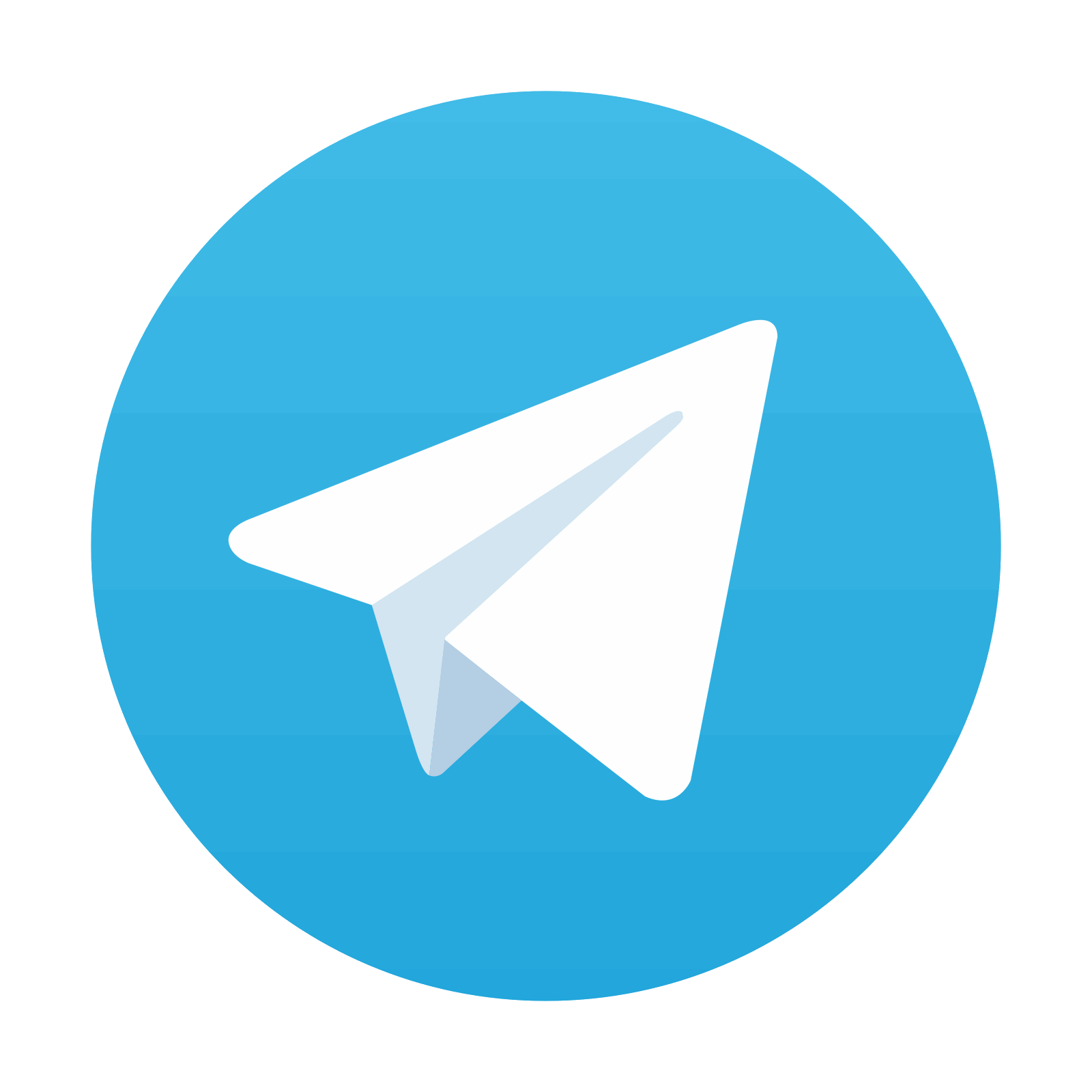
Stay updated, free dental videos. Join our Telegram channel

VIDEdental - Online dental courses
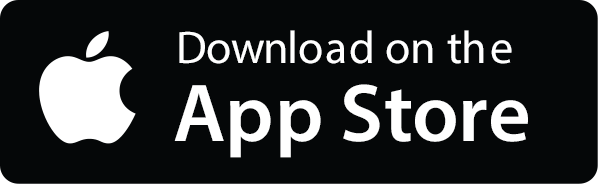
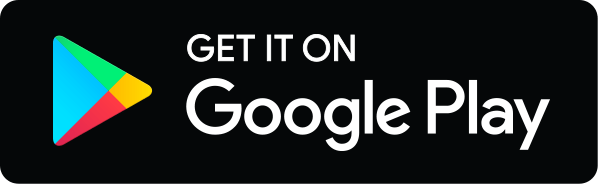
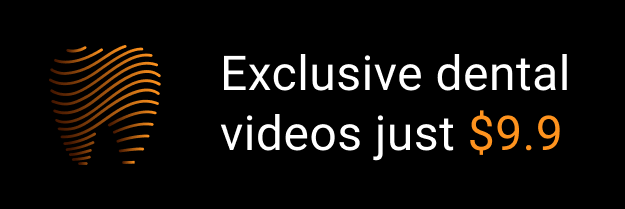