Abstract
A great challenge regarding the ease-of-use of composites involves the development of ‘self-adhesive’ composites that no longer require a separate adhesive to bond to tooth enamel/dentin.
Objectives
To characterize the interfacial ultra-structure of an experimental self-adhesive filling material bonded to enamel and dentin using transmission electron microscopy (TEM).
Methods
The experimental self-adhesive material was bonded to bur-cut human enamel and dentin, and to fractured (smear-free) dentin, strictly according to the manufacturers’ instructions. The specimens were stored for 1 day in distilled water (37 °C) prior to further common specimen processing for TEM.
Results
The experimental self-adhesive filling material revealed a typical micro-hybrid filler distribution. At bur-cut enamel, a tight interface was formed, mostly exhibiting only tiny micro-tags without distinct surface demineralization. At bur-cut dentin, the experimental self-adhesive filling material interacted superficially, with the surface structure being more irregular because of the bur preparation. No clear resin tags were formed due to the obstruction of dentin tubules with smear plugs. At fractured dentin, the formation of a relatively thin hybrid layer of maximum a few hundreds of nanometer was disclosed without clear surface demineralization. Distinct resin tags were formed due to the absence of smear plugs. Silver-nitrate infiltration showed a pattern of spot-like appearance of nano-leakage. Ag deposition was observed more along the dentin-adhesive interface of bur-cut dentin, as compared to that of fractured dentin.
Significance
The obtained tight interface at both enamel and dentin demonstrates the self-adhesive capacity of the experimental self-adhesive filling material.
1
Introduction
Simplification of the clinical application steps needed to bond a composite restoration is highly desirable. This would not only reduce clinical treatment time, but also technique sensitivity. Currently, all resin-based restoratives require a surface pretreatment of enamel and dentin using either an etch-and-rinse or self-etch adhesive. This multi-step application technique is lengthy, rather complex and often very technique sensitive . A new category of composite restoratives was recently introduced that according to the manufacturer’s instructions, do not require any pretreatment of the substrate. Such a ‘self-adhesive filling material’ (coded as ‘Exp. 564’, 3 M ESPE, Seefeld, Germany) was employed in this study, though being still experimental and not commercially available. Its self-adhesiveness is supposedly based upon the use of acidic monomers that demineralise and infiltrate the tooth substrate, resulting in micro-mechanical retention, potentially enhanced by additional chemical interaction. Phosphoric-acid ester methacrylates, as used in Exp. 564, are known functional monomers in dental adhesive formulations for their capacity to demineralise and simultaneously prime tooth surfaces . While the key-feature of such a self-adhering restorative material is the easy, fast and straightforward application procedure, its actual bonding performance is expected to be lower than when an adhesive would be separately used. This seems logical because of the viscous nature of such a material versus the liquid nature of an adhesive that will better wet the surface. Nevertheless, a minimum amount of adhesive capacity could be sufficient to reach clinical success. Therefore, the purpose of this study was to ultra-morphologically characterize the interface of the experimental self-adhesive filling material ‘Exp. 564’ bonded to enamel and dentin using transmission electron microscopy (TEM).
2
Materials and methods
Six non-carious human third molars were stored in 0.5% chloramine solution at 4 °C and used within one month after extraction. The teeth were randomly divided into 3 groups following the three different surface preparation methods applied. All teeth were mounted in gypsum blocks in order to ease manipulation. For the enamel specimens, lingual and buccal enamel was flattened using a medium-grit (100 μm) diamond bur (842, Komet, Lemgo, Germany) in a water-cooled high-speed contra-angle handpiece mounted in the MicroSpecimen Former (The University of Iowa, Iowa, IA, USA). For bur-cut dentin specimens, the occlusal third of the crown was removed at the level of mid-coronal dentin using a slow-speed diamond saw (Isomet 1000, Buehler, Lake Bluff, IL, USA), after which a standard smear layer was produced by the same medium-grit diamond bur, as was also used to prepare the enamel specimens. The last two teeth were used to prepare fractured dentin surfaces, basically excluding any potential smear-layer interference. A shallow 1–2 mm deep groove was cut circumferentially around the tooth at the level of mid-coronal dentin, after which the coronal part was fractured using a forceps. Hence, a fractured dentin surface free of smear debris was produced. All dentin surfaces were carefully verified for absence of enamel and/or pulp tissue using a stereo-microscope (Wild M5A, Heerbrugg, Switzerland). Then, a 1-mm thick layer of ‘Exp. 564’ was placed strictly according to the manufacturer’s instructions ( Table 1 ). Light-curing was performed using an Optilux 500 (Demetron/Kerr, Danbury, CT, USA) device with a light output not less than 600 mW/cm 2 . Specimens were next stored for 1 day in distilled water at 37 °C.
Material | Composition (wt%) | Application |
---|---|---|
Exp. 564 | Component A : 50–70% inorganic fillers; 10–<20% Substituted dimethacrylate; 10–<20% 1,6-hexanediol dimethacrylate; initiators | (1) Mix equal amounts of Components A and B |
Lot. AMIX-0564 | Component B : 15–25% Phosphoric acid-6-methacryloxy-hexylesters; 50–70% inorganic fillers; 5–10% 2-hydroxyethyl methacrylate (HEMA); 1–7% Triethylen glycol dimethacrylate (TEGDMA); <5% 1,6-hexanediol dimethacrylate; initiators | (3) Apply on enamel or dentin (no need to brush) |
3M ESPE, Seefeld, Germany | (4) Light-cure for 20 s |
The specimens were processed for TEM according to the procedure described in detail before by Van Meerbeek et al. . In order to reveal potential porous zones within the interface complex, some of the prepared specimens were immersed in a 50 wt% ammoniacal silver nitrate solution according to a nanoleakage-detection protocol previously described by Tay et al. . Non-demineralized and lab-demineralized ultra-thin sections were cut (Ultracut UCT, Leica, Vienna, Austria) and examined unstained and positively stained (5% uranyl acetate for 12 min/saturated lead citrate for 13 min) using TEM (JEM-1200EX II, JEOL, Tokyo, Japan). After observation of the resin–enamel interface sections, the TEM grids were additionally exposed for 5 s to 0.1 N HCl, and subsequently carefully rinsed with distilled water to remove all dissolved mineral components following a method described before by Hannig et al. . Doing so, the same spots were imaged before and after lab-decalcification.
2
Materials and methods
Six non-carious human third molars were stored in 0.5% chloramine solution at 4 °C and used within one month after extraction. The teeth were randomly divided into 3 groups following the three different surface preparation methods applied. All teeth were mounted in gypsum blocks in order to ease manipulation. For the enamel specimens, lingual and buccal enamel was flattened using a medium-grit (100 μm) diamond bur (842, Komet, Lemgo, Germany) in a water-cooled high-speed contra-angle handpiece mounted in the MicroSpecimen Former (The University of Iowa, Iowa, IA, USA). For bur-cut dentin specimens, the occlusal third of the crown was removed at the level of mid-coronal dentin using a slow-speed diamond saw (Isomet 1000, Buehler, Lake Bluff, IL, USA), after which a standard smear layer was produced by the same medium-grit diamond bur, as was also used to prepare the enamel specimens. The last two teeth were used to prepare fractured dentin surfaces, basically excluding any potential smear-layer interference. A shallow 1–2 mm deep groove was cut circumferentially around the tooth at the level of mid-coronal dentin, after which the coronal part was fractured using a forceps. Hence, a fractured dentin surface free of smear debris was produced. All dentin surfaces were carefully verified for absence of enamel and/or pulp tissue using a stereo-microscope (Wild M5A, Heerbrugg, Switzerland). Then, a 1-mm thick layer of ‘Exp. 564’ was placed strictly according to the manufacturer’s instructions ( Table 1 ). Light-curing was performed using an Optilux 500 (Demetron/Kerr, Danbury, CT, USA) device with a light output not less than 600 mW/cm 2 . Specimens were next stored for 1 day in distilled water at 37 °C.
Material | Composition (wt%) | Application |
---|---|---|
Exp. 564 | Component A : 50–70% inorganic fillers; 10–<20% Substituted dimethacrylate; 10–<20% 1,6-hexanediol dimethacrylate; initiators | (1) Mix equal amounts of Components A and B |
Lot. AMIX-0564 | Component B : 15–25% Phosphoric acid-6-methacryloxy-hexylesters; 50–70% inorganic fillers; 5–10% 2-hydroxyethyl methacrylate (HEMA); 1–7% Triethylen glycol dimethacrylate (TEGDMA); <5% 1,6-hexanediol dimethacrylate; initiators | (3) Apply on enamel or dentin (no need to brush) |
3M ESPE, Seefeld, Germany | (4) Light-cure for 20 s |
The specimens were processed for TEM according to the procedure described in detail before by Van Meerbeek et al. . In order to reveal potential porous zones within the interface complex, some of the prepared specimens were immersed in a 50 wt% ammoniacal silver nitrate solution according to a nanoleakage-detection protocol previously described by Tay et al. . Non-demineralized and lab-demineralized ultra-thin sections were cut (Ultracut UCT, Leica, Vienna, Austria) and examined unstained and positively stained (5% uranyl acetate for 12 min/saturated lead citrate for 13 min) using TEM (JEM-1200EX II, JEOL, Tokyo, Japan). After observation of the resin–enamel interface sections, the TEM grids were additionally exposed for 5 s to 0.1 N HCl, and subsequently carefully rinsed with distilled water to remove all dissolved mineral components following a method described before by Hannig et al. . Doing so, the same spots were imaged before and after lab-decalcification.
3
Results
Ultra-morphologic TEM-characterization of the self-adhesive filling material revealed the presence of a few different kinds of filler, including irregularly polyhedral-shaped particles, irregular particles with rounded edges and a higher electron density as compared to the other filler particles, and nano-sized filler ( Fig. 1 ). The overall filler loading appeared lower than that of conventional composites, and was more in the range of flowable composites and composite cements.
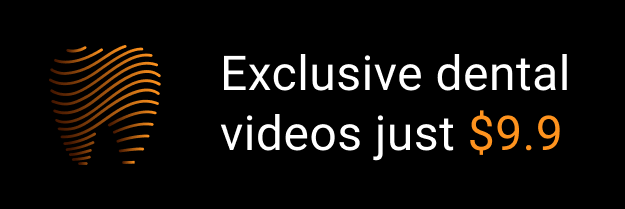