Introduction
This study provides insight into surface and elemental analyses of orthodontic retrieved miniscrew implants (MSIs). The sole purpose was to investigate the behavior of MSIs while they are in contact with bone and soft tissues, fluids, and food in the oral cavity. The information thus gathered may help to understand the underlying process of success or failure of MSIs and can be helpful in improving their material composition and design.
Methods
The study was carried out on 28 titanium-alloy MSIs (all from the same manufacturer) split into 3 groups: 18 MSIs were retrieved after successful orthodontic treatment, 5 were failed MSIs, and 5 were as-received MSIs serving as the controls. All MSIs were subjected to energy dispersive x-ray microanalysis to investigate the changes in surface elemental composition and to scanning electron microscopy to analyze their surface topography. Data thus obtained were subjected to suitable statistical analyses.
Results
Scanning electron microscope analysis showed surface manufacturing imperfections of the as-received MSIs in the form of stripes. Their elemental composition was confirmed to the specifications of the American Society for Testing of Materials for surgical implants. Retrieved MSIs exhibited generalized surface dullness; variable corrosion; craters in the head, neck, body, and tip regions; and blunting on tips and threads. Energy dispersive x-ray analyses showed deposition of additional elements: calcium had greater significance in its proportion in the body region by 0.056 weight percent; iron was seen in greater proportion in the failed retrieved MSIs compared with the successful miniscrews; cerium was seen in greater proportions in the head region by 0.128 weight percent and in the neck region by 0.147 weight percent than in the body and tip regions of retrieved MSIs.
Conclusions
Retrieved MSIs showed considerable surface and structural alterations such as dullness, corrosion, and blunting of threads and tips. Their surfaces showed interactions and adsorption of several elements, such as calcium, at the body region. A high content of iron was found on the failed MSIs, and cerium was seen in the head and neck regions of retrieved MSIs.
Highlights
- •
We analyzed the surfaces and elements of orthodontic miniscrew implants (MSIs) after retrieval.
- •
They had significant alterations: surface dullness, corrosion, and blunting on tips and threads.
- •
Depositions of additional elements were seen on the retrieved MSIs: calcium, iron, and cerium.
- •
The surfaces of the failed MSIs had the highest adsorption values of iron in all 4 zones.
- •
Greater amounts of cerium were observed on the heads and necks of all retrieved MSIs.
The orthodontic miniscrew, a temporary anchorage device, was introduced by Gainsforth and Higley in an animal study. In each of 5 dogs, a Vitallium screw was placed in the anterior border of the ramus of the mandible to apply traction by means of an orthodontic elastic connected to a maxillary appliance for skeletal anchorage. However, Kanomi in 1997 first described the “mini-implant” specifically designed for orthodontic applications. Over the last 2 decades, titanium miniscrews have gained enormous popularity in orthodontics and are often regarded as the source of absolute intraoral anchorage for clinical purposes. The popularity of these devices is due to their low cost, small dimensions, ease of insertion and removal, and the possibility of applying immediate loading, thereby reducing the total orthodontic treatment duration. The introduction of miniscrew implants (MSIs) in the orthodontic armamentarium has widened the scope and envelope of orthodontic treatment to some extent. It is possible to treat patients with moderate to severe skeletal discrepancies and obtain complex tooth movements that were not possible previously. Compared with other forms of compliance-dependent anchorage, MSI-supported anchorage offers a more predictable outcome.
MSIs are manufactured from commercially pure titanium and grade V titanium alloy. Titanium alloy is favored because of its higher strength relative to commercially pure titanium. Contemporary MSIs are designed for ease of insertion and are generally safe to use. However, they have been reported to cause gingival injuries and have occasionally been found to undergo fracture because of mechanical failure in the oral environment. Failed MSIs necessitate their removal or replacement. Several factors influence the success of orthodontic MSIs, including careful patient selection, the characteristics of the implantation site, and the macrostructure and microstructure properties of the implants. Even though titanium alloys are known to be exceptionally corrosion resistant because of the stability of the passive titanium oxide layer on the surface, MSIs have been reported to undergo corrosion after clinical applications. Generally, corrosion is observed when the titanium oxide film breaks down locally, and rapid dissolution of the underlying metal occurs in the form of pits. Crevice corrosion occurs between 2 close surfaces or in constricted places where oxygen exchange is not available. When an implant is milled and placed in bone, the stress on the MSI might lead to stress corrosion or cracking of the alloy. This cracking may propagate in the physiologic or the corrosive environment.
Once the required orthodontic objectives have been achieved, the MSIs are removed from patient’s bone. After removal, the retrieved devices are usually discarded. However, economic factors or environmental conservation might influence clinicians to consider reusing MSIs. Not all temporary implant devices can be reused, but metal implants, such as those made from titanium alloy, may be more amenable to reuse because they can be mechanically and chemically cleaned and resterilized with potentially little or no loss of form or function.
Several studies related to mechanical, chemical, and surface characteristics of prosthetic dental implants are available in the literature. On the other hand, we located 4 studies describing the surface and mechanical natures of retrieved orthodontic MSIs. Eliades et al characterized the morphologic, structural, and compositional alterations, and assessed the changes in the hardness of orthodontic MSIs retrieved after successful service. They reported that used titanium-alloy MSIs have morphologic and surface structural alterations. Their Vickers microhardness testing showed no change in surface hardness of the retrieved specimens compared with the controls. Mattos et al compared the surface morphology and fracture torque resistance of as-received, sterilized, and retrieved mini-implants to evaluate the fracture risks of reusing orthodontic mini-implants after sterilization. They reported that no defects or corrosion could be identified in autoclaved and retrieved mini-implants, but worn surfaces and scratch marks were observed. A statistically significant difference in the fracture torque was observed between the as-received and retrieved groups. Sebbar et al assessed the surface changes in MSIs retrieved after usage and compared them with as-received MSIs under an optical microscope. Used MSIs showed signs of corrosion mainly at the sites of manufacturing defects.
The literature lacks comprehensive information on used miniscrews regarding their surface and elemental composition. Therefore, a study aimed at surface and elemental analyses of successful miniscrews after retrieval compared with failed and as-received miniscrews was undertaken. Our sole purpose was to investigate the behavior of MSIs while in contact with bone and soft tissues, oral fluids, and food. The information thus gathered may help orthodontists to understand the intricacies of success or failure of MSIs related to their design and material composition.
Material and methods
This study was conducted with 28 MSIs, 8 mm long, 1.5 mm in diameter, bracket head type, self-drilling, made from grade V titanium alloy. All MSIs were procured from same manufacturer (Absoanchor; Dentos, Daegu, Korea) to prevent any bias in design and material properties. Of the 28 MSIs, 18 were retrieved from patients after successful service of 12.89 ± 5.33 months. Five MSIs, which had to be retrieved because of loosening failure during treatment (duration, 6.8 ± 2.86 months), constituted the failed retrieved group. All MSIs were retrieved from buccal interradicular bone between the second premolar and the permanent first molar. These retrieved MSIs were used in 8 patients (4 male, 4 female; mean age, 17.75 ± 6.08 years) with Class I bimaxillary protrusion malocclusions. These MSIs were used for en-masse retraction of anterior teeth (11 in the maxillary arch, 12 in the mandibular arch). An orthodontic force of 200 g was applied on each MSI. We used Nitinol closed-coil springs of 9 mm length (GAC International Inc, Central Islip, NY), which applied a consistent retraction force. These retrieved miniscrews were part of the ongoing research in which a standard protocol of insertion and force application was used. Informed consent was obtained from all patients before MSI placement. Five as-received MSIs served as the control group.
Each MSI was retrieved with a long-hand driver supplied by the manufacturer, consisting of body and tip. After removal, each MSI was gently washed and stored completely immersed in fresh deionized water in an autoclaved glass vial duly labeled. Deionized water was considered a suitable medium for storage because it has been reported to be free from any other elements and has the least effect on the dissolution of elements from the cementum of premolars. In addition to its inertness, it also has minimal effects on physical properties such as the hardness and the elastic modulus of teeth.
Analyses by energy dispersive x-ray microanalysis (EDX) and scanning electron microscopy (SEM) were done at 4 zones of each MSI: head, neck, body, and tip ( Fig 1 ). These zones were considered unique because each zone is exposed to a different environment in the body. The head is exposed to oral fluids and food, whereas the neck is in contact with oral mucosa and gingiva, and the body is in contact with bone. Although the tip is immersed in bone, it may show a difference in behavior compared with the body because of its tiny dimensions. Cortical and cancellous bone properties are given in Table I .
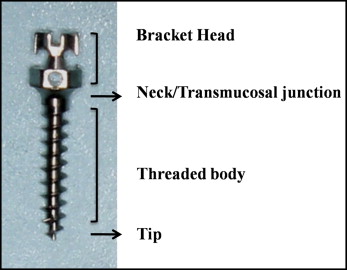
Material | Elastic modulus (GPa) | Density (kg/m 3 ) |
---|---|---|
Cortical bone in maxillary region | 14.7 | 2000 |
Cancellous bone in maxillary region | 1.5 | 1000 |
Artificial bone model outermost layer representing cortical bone (made with short fiber-filled epoxy sheets) | 16.7 | 1660 |
Artificial bone model core layer representing cancellous bone (made with solid polyurethane foam) | 0.759 | 0640 |
MSI | 96 | 4620 |
Each MSI was mounted on a carbon stub and kept in a dessicator for 24 hours. An EDX detector (Oxford Instruments, Abingdon, Oxfordshire, United Kingdom) was used to investigate their elemental composition with an x-ray microanalysis detector. The quantitative analysis of the percentage of weight concentration of the probed elements was performed with an INCA microanalyzer (version 4.06; Oxford Instruments). The carbon quantification was excluded from this study because of the technical limitation of the EDX in an electron microscope. Elements with lower atomic masses such as carbon (atomic number 6 and lower) are difficult to distinguish from each other using EDX. The carbon x-rays have low energy and are easily absorbed by the x-ray detector windows. Furthermore, there can be a significant carbon background signal because of hydrocarbon contamination. Hydrocarbons from the chamber surfaces, vacuum pumps, and sample surface migrate and react with the electron beam to form a black spot that is rich in carbon.
After elemental analysis with the EDX, the retrieved MSIs were subjected to a cleaning cycle of 30 minutes in an ultrasonicator, completely immersed in enzymatic detergent (Cidezyme; ASP: a Johnson and Johnson company, Irvine, Calif) so that organic debris would be removed and the surface topography of the MSIs could be fully observed under the microscope. After cleaning, they were again mounted on carbon stubs and kept in a dessicator for 24 hours, and surface images were taken with SEM (Leo 435VP; SEMTech Solutions, Cambridge, United Kingdom). Alterations of surface changes were looked for: eg, crevice corrosion, corrosion surface damages, dullness, cracks, craters, fractures, and blunting ( Table II ). After initial scanning of each zone, images of the damaged features or areas were captured. Multiple images of each zone of the MSI were taken; the number of images varied for each zone (about 2-4 images per zone of each MSI). Digital images were obtained at various magnifications (20-500 times) in an incremental manner. Experimental conditions were vacuum at 10 −4 mm of mercury; extra high tension voltage level, 20 keV; working distance, 37 mm; and dead time, below 30%.
Zone/Group | Corrosion surface damage | P value | Dullness | P value | Cracks | P value | Craters | P value | Fracture | P value | Blunting | P value | ||||||
---|---|---|---|---|---|---|---|---|---|---|---|---|---|---|---|---|---|---|
S | T | S | T | S | T | S | T | S | T | S | T | |||||||
Head | ||||||||||||||||||
C | 0 | 15 | 0 | 5 | 0 | 5 | 0 | 5 | 0 | 5 | NA | |||||||
FR | 7 | 15 | 0.49 | 5 | 5 | 0 ∗ | 0 | 5 | 0.4 | 1 | 5 | 0.79 | 0 | 5 | 1 | |||
SR | 31 | 54 | 18 | 18 | 5 | 18 | 4 | 18 | 2 | 18 | ||||||||
Neck | ||||||||||||||||||
C | 0 | 15 | 0 | 5 | 0 | 5 | 0 | 5 | 0 | 5 | NA | |||||||
FR | 7 | 15 | 0.72 | 5 | 5 | 0 ∗ | 0 | 5 | NA | 3 | 5 | 0.14 | 0 | 5 | NA | |||
SR | 28 | 54 | 18 | 18 | 0 | 18 | 8 | 18 | 0 | 18 | ||||||||
Body | ||||||||||||||||||
C | 0 | 15 | 0 | 5 | 0 | 5 | 0 | 5 | 0 | 5 | 0 | 5 | ||||||
FR | 12 | 15 | 0.009 ∗ | 5 | 5 | 0 ∗ | 0 | 5 | 1 | 3 | 5 | 0 ∗ | 0 | 5 | 0.41 | 5 | 5 | 0 ∗ |
SR | 54 | 54 | 18 | 18 | 3 | 18 | 18 | 18 | 4 | 18 | 18 | 18 | ||||||
Tip | ||||||||||||||||||
C | 0 | 15 | 0 | 5 | 0 | 5 | 0 | 5 | 0 | 5 | 0 | 5 | ||||||
FR | 7 | 15 | 0.001 ∗ | 5 | 5 | 0 ∗ | 1 | 5 | 1 | 1 | 5 | 0.35 | 0 | 5 | 1 | 5 | 5 | 0 ∗ |
SR | 47 | 54 | 18 | 18 | 3 | 18 | 7 | 18 | 2 | 18 | 18 | 18 |
∗ P <0.05 was statistically significant; Fischer exact test applied.
Statistical analysis
The statistical analysis of the data was performed using SPSS statistical software (version 20; IBM, Armonk, NY). The Kruskal-Wallis and the Wilcoxon rank sum tests were applied to compare the EDX data on elemental composition, and the chi-square test was used for the comparison of the SEM results. We had 3 groups (control, and successful and failed MSIs) and 4 zones (head, neck, body, and tip). To understand the variations, 2 types of analysis were performed: comparisons among the groups and zones, and multiple comparisons between any 2 groups at a time. The Kruskal-Wallis method was used to find the P values among the 3 groups; P values less than 0.05 were considered significant. In the case of multiple comparisons within the 3 groups—analyzing any 2 groups at a time—post hoc analysis of variance was done with the Wilcoxon rank sum method and the Bonferroni correction; P values less than 0.016 were considered significant. The Kruskal-Wallis and Wilcoxon rank sum test results are shown in Tables III and IV .
Element/Group | Head | Neck | Body | Tip | P value for comparison within groups | P value for comparison within zones |
---|---|---|---|---|---|---|
Titanium | ||||||
C | 89.75 ± 0.07 | 89.75 ± 0.07 | 89.75 ± 0.07 | 89.75 ± 0.07 | 0.03 ∗ | 0.67 |
FR | 73.264 ± 14.084 | 75.202 ± 13.848 | 82.018 ± 9.728 | 73.295 ± 16.854 | ||
SR | 84.395 ± 5.489 | 74.222 ± 14.408 | 90.322 ± 1.641 | 70.871 ± 14.16 | ||
Aluminum | ||||||
C | 5.97 ± 0.01 | 5.97 ± 0.01 | 5.97 ± 0.01 | 5.97 ± 0.01 | 0.001 ∗ | 0.656 |
FR | 3.762 ± 1.536 | 2.755 ± 2.055 | 3.659 ± 2.926 | 2.92 ± 1.568 | ||
SR | 4.304 ± 2.739 | 2.769 ± 2.341 | 0.805 ± 0.300 | 2.534 ± 1.421 | ||
Vanadium | ||||||
C | 4.07 ± 0.07 | 4.07 ± 0.07 | 4.07 ± 0.07 | 4.07 ± 0.07 | 0.001 ∗ | 0.639 |
FR | 3.276 ± 2.248 | 1.69 ± 1.477 | 1.786 ± 1.581 | 1.502 ± 1.349 | ||
SR | 0.811 ± 1.404 | 0.865 ± 1.499 | 2.071 ± 3.558 | 1.249 ± 1.093 | ||
Oxygen | ||||||
C | 0.10 ± 0.01 | 0.10 ± 0.01 | 0.10 ± 0.01 | 0.10 ± 0.01 | 0.009 ∗ | 0.384 |
FR | 11.354 ± 18.002 | 19.011 ± 9.385 | 10.186 ± 8.183 | 19.985 ± 12.829 | ||
SR | 8.795 ± 4.008 | 21.302 ± 11.253 | 5.813 ± 5.105 | 23.742 ± 11.344 | ||
Calcium | ||||||
C | 0.00 ± 0.00 | 0.00 ± 0.00 | 0.00 ± 0.00 | 0.00 ± 0.00 | 0.031 ∗ | 0.529 |
FR | 0.215 ± 0.187 | 0.17 ± 0.03 | 0.165 ± 0.181 | 0.189 ± 0.17 | ||
SR | 0.162 ± 0.169 | 0.15 ± 0.026 | 0.221 ± 0.229 | 0.139 ± 0.144 | ||
Iron | ||||||
C | 0.00 ± 0.00 | 0.00 ± 0.00 | 0.00 ± 0.00 | 0.00 ± 0.00 | 0.045 ∗ | 0.218 |
FR | 0.504 ± 0.161 | 0.87 ± 0.116 | 0.387 ± 0.374 | 0.266 ± 0.076 | ||
SR | 0.305 ± 0.329 | 0.59 ± 0.102 | 0.160 ± 0.204 | 0.114 ± 0.129 | ||
Cerium | ||||||
C | 0.00 ± 0.00 | 0.00 ± 0.00 | 0.00 ± 0.00 | 0.00 ± 0.00 | 0.136 | 0.049 ∗ |
FR | 0.126 ± 0.149 | 0.184 ± 0.318 | 0.00 ± 0.00 | 0.00 ± 0.00 | ||
SR | 0.131 ± 0.228 | 0.111 ± 0.097 | 0.00 ± 0.00 | 0.00 ± 0.00 | ||
Nitrogen | ||||||
C | 0.00 ± 0.00 | 0.00 ± 0.00 | 0.00 ± 0.00 | 0.00 ± 0.00 | 0.293 | 0.412 |
FR | 2.58 ± 3.62 | 3.04 ± 4.58 | 1.54 ± 1.45 | 0.00 ± 0.00 | ||
SR | 1.97 ± 3.90 | 1.70 ± 2.62 | 5.86 ± 11.61 | 0.02 ± 0.06 |
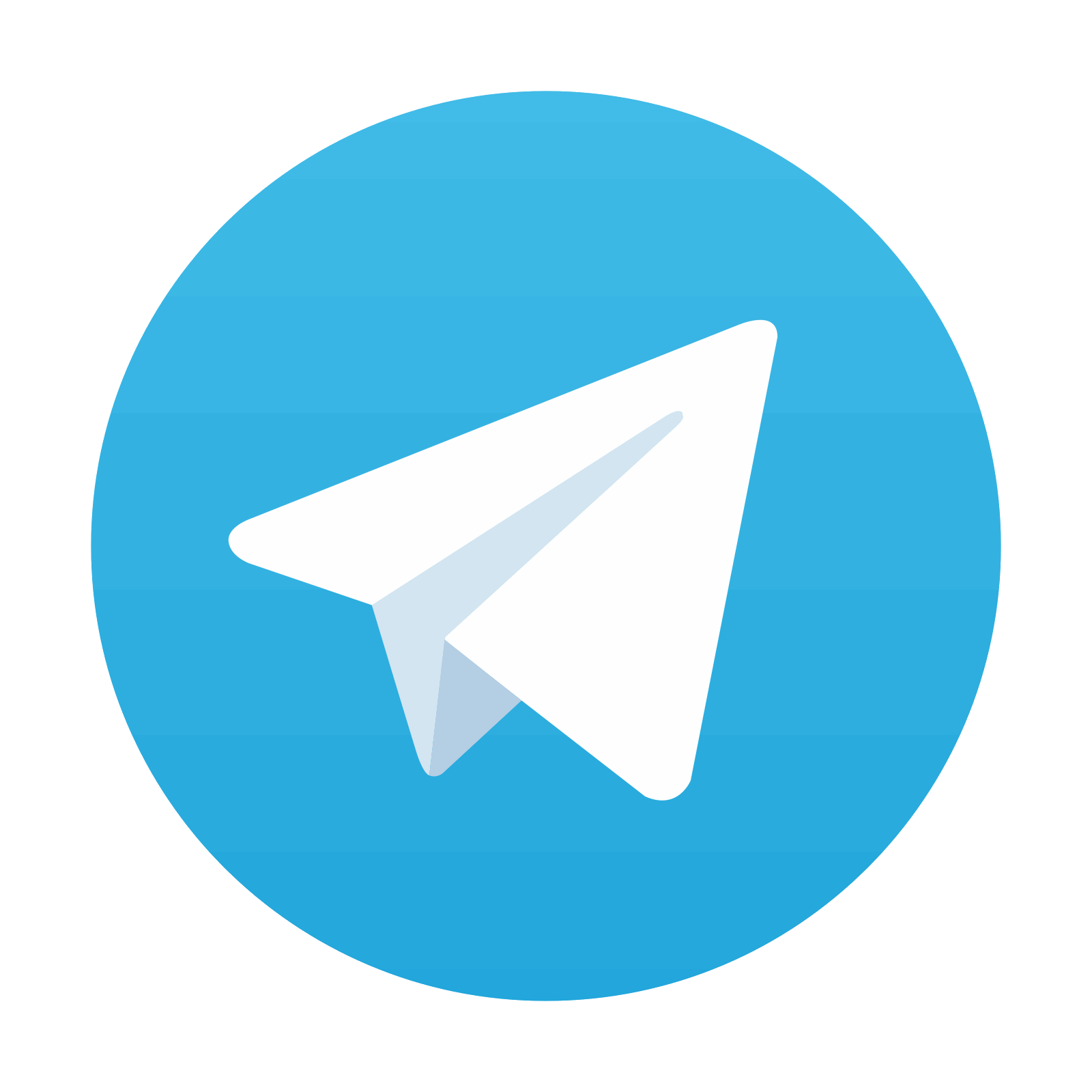
Stay updated, free dental videos. Join our Telegram channel

VIDEdental - Online dental courses
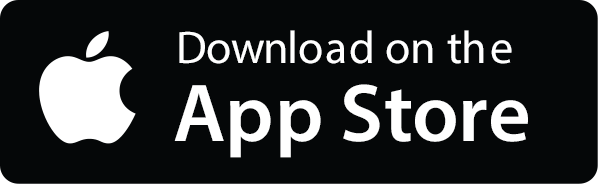
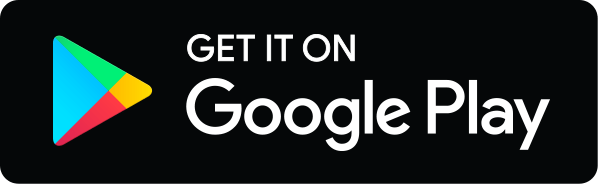
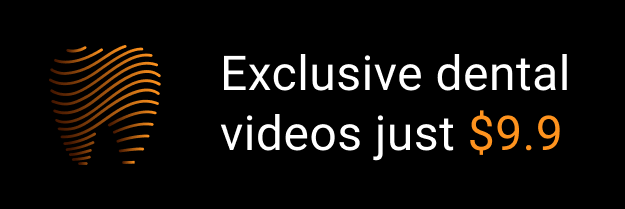