Abstract
Objectives
This study characterized the feldspathic ceramic surfaces after various silanization protocols.
Methods
Ceramic bars (2 mm × 4 mm × 10 mm) ( N = 18) of feldpathic ceramic (VM7, VITA Zahnfabrik) were manufactured and finished. Before silane application, the specimens were ultrasonically cleaned in distilled water for 10 min. The ceramic specimens were randomly divided into nine groups ( N = 2 per group) and were treated with different silane protocols. MPS silane (ESPE-Sil, 3M ESPE) was applied to all specimens and left to react at 20 °C for 2 min (G20). After drying, the specimens were subjected to heat treatment in an oven at 38 °C (G38), 79 °C (G79) or 100 °C (G100) for 1 min. Half of the specimens of each group were rinsed with water at 80 °C for 15 s (G20B, G38B, G79B, G100B). The control group (GC) received no silane. Attenuated total reflection infrared Fourier transform analysis (ATR FT-IR) was performed using a spectrometer. Thickness of silane layer was measured using a spectroscopic ellipsometer working in the λ = 632.8 nm (He–Ne laser) at 70° incidence angle. Surface roughness was evaluated using an optical profilometer. Specimens were further analyzed under the Scanning Electron Microscopy (SEM) to observe the topographic patterns.
Results
ATR FT-IR analysis showed changes in Si–O peaks with enlarged bands around 940 cm −1 . Ellipsometry measurements showed that all post-heat treatment actions reduced the silane film thickness (30.8–33.5 nm) compared to G20 (40 nm). The groups submitted to rinsing in hot water (B groups) showed thinner silane films (9.8–14.4 nm) than those of their corresponding groups (without washing) (30.8–40 nm). Profilometer analysis showed that heat treatments (Ra ≈ 0.10–0.19 μm; Rq ≈ 0.15–0.26 μm) provided a smoother surface than the control group (Ra ≈ 0.48 μm; Rq ≈ 0.65 μm). Similar patterns were also observed in SEM images.
Significance
Heat treatment after MPS silane application improved the silane layer network. Rinsing with boiling water eliminated the outmost unreacted regions of the silane yielding to thinner film thicknesses.
1
Introduction
The cementation process of a resin-bonded restoration is the final step of operative treatment after a series of procedures in dentistry. For adhesive cementation of glassy matrix ceramics, after etching with hydrofluoric acid gel (HF), it is a well-established method to use a silane coupling agent, a monomer with reactive organic radicals and hydro-soluble monovalent group. Silane coupling agents promote adhesion between inorganic phase of ceramic and organic phase of bonding agent applied on the ceramic surface through siloxane bonds. In addition, silane increases the surface energy of ceramic substrates and improves cement damping. These events consequently promote microscopic interactions between both parts. This approach made it possible today to adhere minimal invasive restorations to dental tissues that do not rely on mechanical retention principles.
The randomly distributed groups of silicon oxides on the glass surface adsorb water as a hydroxyl group, forming a weak hydrogen bond . It is also possible that other oxides present on ceramic surface absorb or adsorb water. In fact, there is a kind of competition between silane and water at available bond sites. Hydrogen bonds are formed during and after silanization but according to Plueddemann there are also covalent bonds between silane and the substrate surface.
The poly-condensation of bifunctional silane monomers with the general formula of (R–O–) 3-Si–O–R, R being the first and O–R the second silane functionality, can provide a highly cross-linked and reactive poly-organosiloxane layer to ceramics . However, their hydrolytic degradation is still of concern . Therefore, attempts are being made to increase their cross-linking . Hydrolysis of silane in water diminishes the performance of silane and thereby, limits the lifetime of adhesive joints . When small molecules of water penetrate into the adhesive interface, existing physical bonds are destroyed and from the untreated surfaces cracks may initiate yielding to detachment of the resin-based materials . In order to minimize this effect, prior to silane application, glass ceramic surfaces are etched with HF that selectively removes the glassy matrix. Consequently, with the increased surface roughness, micromechanical retention is facilitated for adhesive procedures. However, the high chemical toxicity, the possibility to produce insoluble silica–fluoride salts that can interfere with the resin bond and the fact that some high crystalline content ceramics may not benefit from HF etching, can be considered as some of the reasons to eliminate HF from the bonding procedure . When the goal is to eliminate the HF etching procedure without jeopardizing the chemical reaction at the ceramic–silane–resin interfaces, the traditional bonding protocol for cementation or repairs needs to be modified to obtain the best silane effect in bonding resin to ceramic .
The silane drying conditions and post-heat treatment procedures reduce solvents and enhances the cross-linking reaction . The extent of cross-linking is found to increase from the outer layers of the silane toward the glass surface with the corresponding increase in the mechanical and hydrothermal stability . The thickness, orientation and cross-linking of the silane layers are determined by a number of factors, such as conditions of deposition, solution pH, silane concentration, nature of solvents, hydrolyzing time, drying temperature, and time between silane application and bonding .
Three different structural regions are proposed in the deposited silane film: (a) a physiosorbed region; (b) a chemisorbed region; and (c) a chemically reacted region. The outermost physisorbed region, that is the bulk of the deposited silane, consists of weak oligomeric siloxanols that hydrolyzes easily and can be eliminated in room temperature at relative humidity. The chemisorbed region, consisting of stronger oligomeric siloxanols, offers a greater resistance to hydrolysis and can be eliminated at high temperatures in water. The most inner region that is close to the ceramic surface, is stable and resistant to extraction in hot water and may be regarded as the chemically reacted region of the silane film .
Heat treatment could be achieved either in an oven or by application of hot water. From clinical perspective, the latter may be more difficult to achieve in chairside applications. If the heat treatment in oven would result in similar silane quality, its application would be more convenient on ceramic surfaces prior to adhesive cementation procedures.
The objective of this study therefore, was to characterize the feldspathic ceramic surface after various silanization protocols including post-heat treatment in an oven or rinsing with hot water. The tested hypothesis was that heat treatment of the silane film would improve its chemical reactivity and the resistance to hydrolysis.
2
Materials and methods
2.1
Specimen preparation and experimental groups
The brand name, type, chemical composition, manufacturer and batch number of each material used in this study are listed in Table 1 .
Brand | Type | Chemical composition | Manufacturer | Batch number |
---|---|---|---|---|
VM7 | Feldspathic ceramic | Glass frits melted in metal oxides | Vita Zanhfabrik, Bad Säckingen, Germany | 7404 |
ESPE-Sil | Silane coupling agent | Ethyl alcohol, 3-methacryloxypropyltrimethoxy, methyl ethyl ketone | 3M ESPE, St. Paul, MN, USA | 286318 |
One dental technician fabricated all the ceramic bar-shaped specimens ( N = 18) out of a feldspathic ceramic (VM7, VITA Zahnfabrik, Bad Säckingen, Germany) according to the manufacturer’s instructions. Initially, an acrylic resin pattern (2.5 mm × 5 mm × 13 mm) was fabricated and impression was taken by polyvinyl siloxane impression material (Elite HD, Zhermach, Badia Polesine, Italy). The ceramic powder (VITA VM7 Dentin 5M2, VITA Zahnfabrik) and modeling liquid (Modeling for Liquid Vita VM7, VITA Zahnfabrik) were mixed according to the manufacturer’s instructions. The ceramic slurry was placed in small portions using a spatula, condensed inside the mold and vibrated manually. The excess fluid was removed with soft absorbent paper. The green ceramic bars were carefully removed from the mold, allowed to dry and sintered in the furnace (Vacumat 40, VITA Zahnfabrik) following the firing cycle recommended by the manufacturer. Considering a sintering contraction of about 20%, the bar-shaped ceramic specimens had, approximately, 2 mm × 4 mm × 10 mm.
Subsequently, the ceramic specimens were ground finished to 1200-grit silicon carbide metallographic abrasive paper (3M ESPE, St. Paul, MN, USA) under water-cooling in a polishing machine (DP-10, Panambra, São Paulo, Brazil). Prior to the silane application, the specimens were ultrasonically cleaned (Vitasonic, Vita Zanhfabrik) in distilled water bath for 10 min and dried using oil-free air spray for 30 s.
The ceramic specimens were randomly divided into nine groups ( N = 2) and were treated with different silane protocols by one person. A 3-methacryloxypropyltrimethoxy silane (ESPE-Sil, 3M ESPE) was applied on the ceramic surface and left to react for 2 min with the surface as follows:
-
G20 – at room temperature (20 °C) and 23% relative humidity;
-
G38 – similar to G20. Subsequently, the specimens were subjected to heat treatment at 38 °C for 1 min using an oven;
-
G79 – similar to G20. Subsequently, the specimens were subjected to heat treatment at 79 °C for 1 min using an oven;
-
G100 – similar to G20. Subsequently, the specimens were subjected to heat treatment at 100 °C for 1 min using an oven;
-
GC (control group) – received no silane.
After drying, two specimens of each group (G20, G38, G79, G100) were rinsed with water at 80 °C for 15 s (G20B, G38B, G79B, G100B).
2.2
Silane film measurement
The thickness of the silane film was determined using a Spectroscopic Ellipsometer (DRE-ELX02, Ratzeburg, German) working with λ = 632.8 nm (He–Ne laser) at 70° incidence angle. The measurements were made after surface drying using oil-free air spray for 30 s and after rinsing with hot water. Since the ceramic specimens did not provide adequate light reflection, silane was applied onto smooth (Ra < 10 nm) and highly reflecting electropolished silicon specimens (10 mm × 10 mm). The optical constants of the substrate were determined from measurements (4 s) of the ellipsometric parameters ( ψ and Δ) before silane application.
2.3
Surface roughness analysis
The average surface roughness (Ra) and the root mean square roughness (Rq) of the ceramic surfaces were measured before and after silane application. Roughness measurements were made in 3 different regions (75 μm × 75 μm) of the ceramic surfaces using an optical profilometer (Wyko NT 1100, Veeco, Tucson, USA) that generated 3D images of the surfaces that were analyzed with the Wyko Vision 32 software (Veeco, USA). The vertical measurement range of the optical profilometer was 0.1 nm–1 mm with the vertical resolution of Ra < 1 Å.
Specimens were further gold sputtered in a sputtering device and analyzed under the Scanning Electron Microscope (SEM) (Zeiss DSM 950, Carl Zeiss, Jena, Germany) at 25 kV to observe the topographical changes of the silane treated surfaces.
2.4
FT-IR analysis
The Attenuated total reflection infrared Fourier transform (ATR FT-IR) analysis was performed using an FT-IR spectrophotometer (BOMEM MB-102, Quebec, Canada) and an optically dense zinc–selenide crystal.
The ceramic surface was placed in contact with the ATR crystal. An infrared beam was directed to a high refractive index crystal. The internal reflectance creates a passing wave that extends outside the crystal to the entire specimen surface that is in contact with the crystal. The attenuated energy from each passing wave returns to the IR beam that is located on the opposite side of the crystal. Once the IR beam is detected, the IR spectrometer system generates an infrared spectrum. All transmission spectra were recorded at 4 cm −1 resolution in the range of 400–4000 cm −1 . Sixty-four scans were made to obtain each spectrum.
2
Materials and methods
2.1
Specimen preparation and experimental groups
The brand name, type, chemical composition, manufacturer and batch number of each material used in this study are listed in Table 1 .
Brand | Type | Chemical composition | Manufacturer | Batch number |
---|---|---|---|---|
VM7 | Feldspathic ceramic | Glass frits melted in metal oxides | Vita Zanhfabrik, Bad Säckingen, Germany | 7404 |
ESPE-Sil | Silane coupling agent | Ethyl alcohol, 3-methacryloxypropyltrimethoxy, methyl ethyl ketone | 3M ESPE, St. Paul, MN, USA | 286318 |
One dental technician fabricated all the ceramic bar-shaped specimens ( N = 18) out of a feldspathic ceramic (VM7, VITA Zahnfabrik, Bad Säckingen, Germany) according to the manufacturer’s instructions. Initially, an acrylic resin pattern (2.5 mm × 5 mm × 13 mm) was fabricated and impression was taken by polyvinyl siloxane impression material (Elite HD, Zhermach, Badia Polesine, Italy). The ceramic powder (VITA VM7 Dentin 5M2, VITA Zahnfabrik) and modeling liquid (Modeling for Liquid Vita VM7, VITA Zahnfabrik) were mixed according to the manufacturer’s instructions. The ceramic slurry was placed in small portions using a spatula, condensed inside the mold and vibrated manually. The excess fluid was removed with soft absorbent paper. The green ceramic bars were carefully removed from the mold, allowed to dry and sintered in the furnace (Vacumat 40, VITA Zahnfabrik) following the firing cycle recommended by the manufacturer. Considering a sintering contraction of about 20%, the bar-shaped ceramic specimens had, approximately, 2 mm × 4 mm × 10 mm.
Subsequently, the ceramic specimens were ground finished to 1200-grit silicon carbide metallographic abrasive paper (3M ESPE, St. Paul, MN, USA) under water-cooling in a polishing machine (DP-10, Panambra, São Paulo, Brazil). Prior to the silane application, the specimens were ultrasonically cleaned (Vitasonic, Vita Zanhfabrik) in distilled water bath for 10 min and dried using oil-free air spray for 30 s.
The ceramic specimens were randomly divided into nine groups ( N = 2) and were treated with different silane protocols by one person. A 3-methacryloxypropyltrimethoxy silane (ESPE-Sil, 3M ESPE) was applied on the ceramic surface and left to react for 2 min with the surface as follows:
-
G20 – at room temperature (20 °C) and 23% relative humidity;
-
G38 – similar to G20. Subsequently, the specimens were subjected to heat treatment at 38 °C for 1 min using an oven;
-
G79 – similar to G20. Subsequently, the specimens were subjected to heat treatment at 79 °C for 1 min using an oven;
-
G100 – similar to G20. Subsequently, the specimens were subjected to heat treatment at 100 °C for 1 min using an oven;
-
GC (control group) – received no silane.
After drying, two specimens of each group (G20, G38, G79, G100) were rinsed with water at 80 °C for 15 s (G20B, G38B, G79B, G100B).
2.2
Silane film measurement
The thickness of the silane film was determined using a Spectroscopic Ellipsometer (DRE-ELX02, Ratzeburg, German) working with λ = 632.8 nm (He–Ne laser) at 70° incidence angle. The measurements were made after surface drying using oil-free air spray for 30 s and after rinsing with hot water. Since the ceramic specimens did not provide adequate light reflection, silane was applied onto smooth (Ra < 10 nm) and highly reflecting electropolished silicon specimens (10 mm × 10 mm). The optical constants of the substrate were determined from measurements (4 s) of the ellipsometric parameters ( ψ and Δ) before silane application.
2.3
Surface roughness analysis
The average surface roughness (Ra) and the root mean square roughness (Rq) of the ceramic surfaces were measured before and after silane application. Roughness measurements were made in 3 different regions (75 μm × 75 μm) of the ceramic surfaces using an optical profilometer (Wyko NT 1100, Veeco, Tucson, USA) that generated 3D images of the surfaces that were analyzed with the Wyko Vision 32 software (Veeco, USA). The vertical measurement range of the optical profilometer was 0.1 nm–1 mm with the vertical resolution of Ra < 1 Å.
Specimens were further gold sputtered in a sputtering device and analyzed under the Scanning Electron Microscope (SEM) (Zeiss DSM 950, Carl Zeiss, Jena, Germany) at 25 kV to observe the topographical changes of the silane treated surfaces.
2.4
FT-IR analysis
The Attenuated total reflection infrared Fourier transform (ATR FT-IR) analysis was performed using an FT-IR spectrophotometer (BOMEM MB-102, Quebec, Canada) and an optically dense zinc–selenide crystal.
The ceramic surface was placed in contact with the ATR crystal. An infrared beam was directed to a high refractive index crystal. The internal reflectance creates a passing wave that extends outside the crystal to the entire specimen surface that is in contact with the crystal. The attenuated energy from each passing wave returns to the IR beam that is located on the opposite side of the crystal. Once the IR beam is detected, the IR spectrometer system generates an infrared spectrum. All transmission spectra were recorded at 4 cm −1 resolution in the range of 400–4000 cm −1 . Sixty-four scans were made to obtain each spectrum.
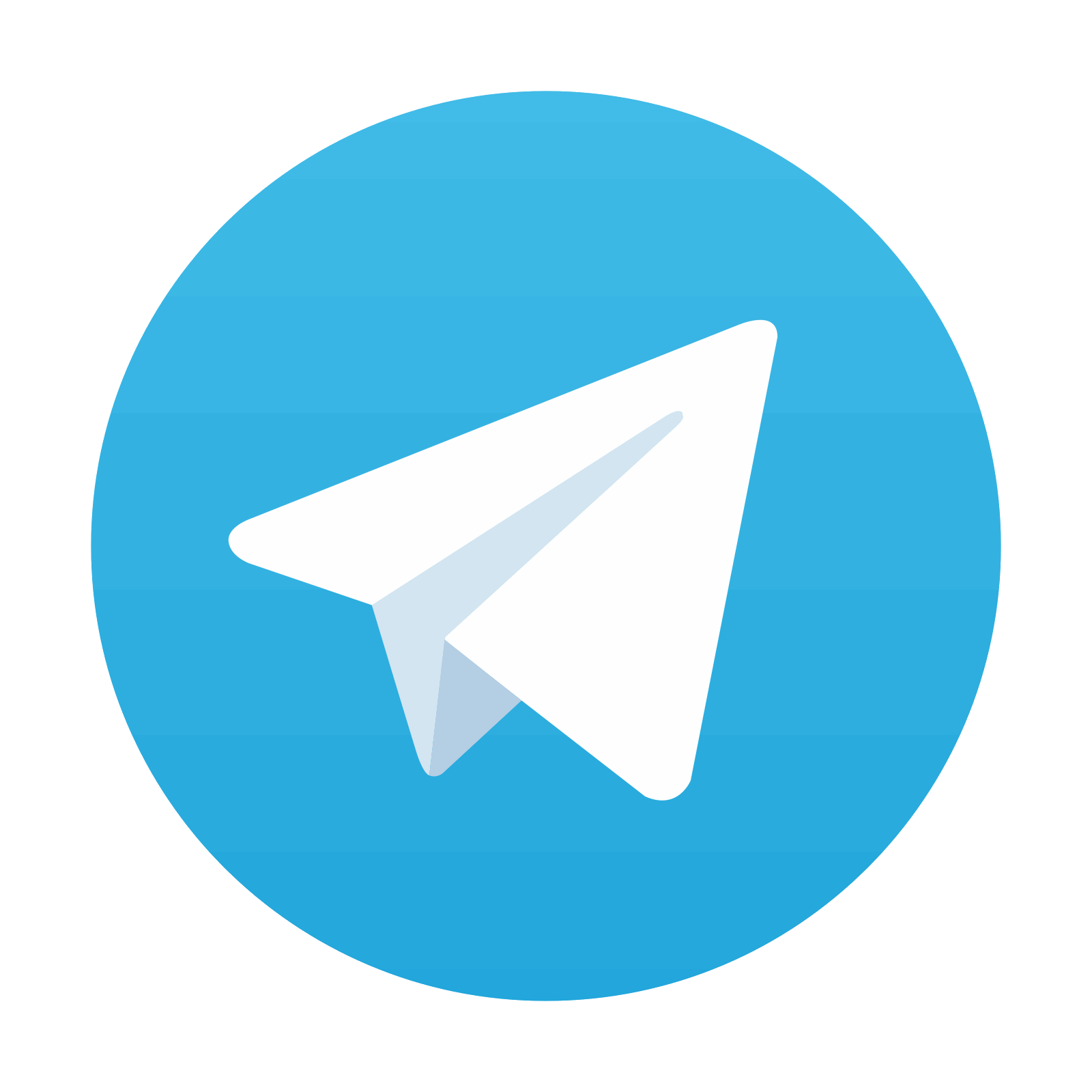
Stay updated, free dental videos. Join our Telegram channel

VIDEdental - Online dental courses
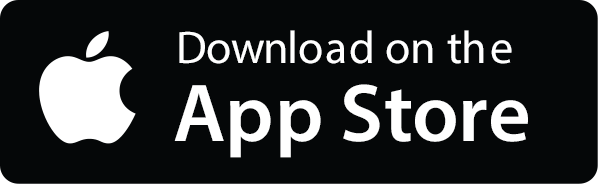
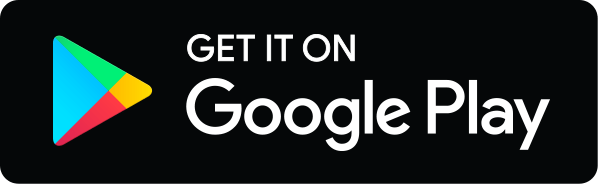