Introduction
In this study, we analyzed the surface roughness (SR) and surface free energy (SFE) characteristics of various orthodontic adhesives and their effects on the adhesion of mutans streptococci (MS).
Methods
Three nonfluoride-releasing composites, 1 fluoride-releasing composite, 1 polyacid-modified composite (compomer), and 2 resin-modified glass ionomers (RMGIs) were analyzed with confocal laser scanning microscopy and the sessile drop method. Each material was incubated with whole saliva or phosphate-buffered saline solution. Adhesion assays were then performed by incubating each material with tritium-labeled cariogenic streptococci.
Results
We found that SFE and its components were significantly different among the adhesives, despite relatively uniform SR. RMGIs showed significantly higher SFE—particularly, dispersive, polar, and acidic characteristics—than did the composites. The compomer had intermediate characteristics between the composites and RMGIs. MS adhered to RMGIs significantly more than to the composites and the compomer, but there was no significant difference among the composites. The longer incubation time increased MS adhesion, whereas saliva coating did not significantly influence MS adhesion. There was a correlation between SFE and MS adhesion. SFE and its dispersive, polar, and acid components significantly influenced MS adhesion irrespective of saliva coating.
Conclusions
This study suggests that initial MS adhesion is significantly influenced by SFE characteristics of adhesives rather than SR or saliva coating.
Enamel demineralization is a commonly recognized complication of orthodontic treatment with a fixed orthodontic appliance. Enamel demineralization is caused by organic acids that are mainly produced by mutans streptococci (MS). The placement of a fixed orthodontic appliance causes more MS in the dental plaque, and the elevated levels return to normal after appliance removal.
Clinical observations have indicated that the most common sites for demineralization are at the orthodontic adhesive and enamel junction, which is peripheral and commonly gingival to the bracket base. The remaining adhesive on the enamel surface around the bracket base is a potent predisposing factor for enamel demineralization, because a rough adhesive surface can provide the site for rapid attachment and growth of oral microorganisms. Bacterial adhesion to adhesive surfaces is an important initial stage in dental plaque formation and enamel demineralization during orthodontic treatment.
There are many commercially available orthodontic adhesives, of which composite resins and glass ionomer cements are the 2 main classes. Recently, hybrid materials comprising resin and glass ionomer components have been developed for greater bonding strength and sustained fluoride release, because conventional glass-ionomer cements were reported to be unreliable for bracket bonding. Although the physical properties and fluoride-releasing capacities of hybrid materials have been extensively studied, biologic properties associated with MS adhesion have not been thoroughly investigated.
Surface free energy (SFE), and its components, and surface roughness (SR) are reported to be important surface characteristics for bacterial adhesion. SFE has been related to the ability of dental materials to promote or prevent adhesion of dental plaque in the oral cavity. Orthodontic adhesives vary in their surface characteristics because of different matrices and setting mechanisms, which can in turn induce differences in MS adhesion. The purposes of this study were to analyze the differences in SR and SFE and its components of various orthodontic adhesives and to evaluate the relationship between surface characteristics and MS adhesion patterns.
Material and methods
Seven light-cured orthodontic adhesives were selected: 3 nonfluoride composites, a fluoride-releasing composite, a polyacid-modified composite (compomer), and 2 resin-modified glass ionomer cements (RMGI) ( Table I ). Specimens were prepared by using synthetic fluorine-containing resin templates (3.0 mm wide and 2.0 mm deep for adhesion assays, and 12.0 mm wide and 2.0 mm deep for surface characterization). Template plates were positioned on top of the glass slides. Each adhesive was placed into the holes until the materials were flush with the top of the templates. A second slide was placed on top, pushed down to ensure flat dorsal surfaces, and then gently removed. All materials were handled according to the manufacturers’ instructions and light cured for 40 seconds (20 seconds from the top and 20 seconds from the bottom).
Adhesive | Characteristics | Manufacturer |
---|---|---|
Enlight | Light-cured composite | Ormco/A Company, Glendora, Calif |
Lightbond | Fluoride-releasing light-cured composite | Reliance Orthodontics, Itasca, Ill |
Monolok2 | Light-cured composite | Rocky Mountain Orthodontics, Denver, Colo |
Transbond XT | Light-cured composite | 3M Unitek, Monrovia, Calif |
Transbond Plus | Light-cured polyacid-modified composite | 3M Unitek, Monrovia, Calif |
Fuji Ortho LC | Fluoride-releasing light-cured resin-modified glass ionomer | GC Corporation, Tokyo, Japan |
Multi-cure | Fluoride-releasing light-cured resin-modified glass ionomer | 3M Unitek, Monrovia, Calif |
SR was analyzed by using confocal laser scanning microscopy (Axiovert 200M, Carl Zeiss, Thornwood, NY). The arithmetic SR mean was calculated from a mean plane in the sampling area (245 × 245 × 60 μm) by using a multi-argon, 633-nm wavelength laser.
SFE, an important parameter for describing the reactivity of a surface, is defined as the work required to increase the area of a substance by 1 cm 2 . It is possible to measure SFE in terms of its components parts: the dispersive (γ LW ) and polar acid/base component (γ AB ), which is divided into acid (γ + ) and base (γ – ) components, by the Lifschitz van der Waals acid-base method. In this study, SFE and its component parts were measured by the sessile drop method. Three probe liquids of different polarities were used: 1-bromonaphthalene (>99%; ACROS Organics, Fairlawn, NJ), formamide (>98%; Mallinckrodt Baker, Phillipsburg, NJ), and deionized distilled water. A video camera with an image analyzer (Phoenix 300, Surface Electro Optics, Seoul, Korea) visualized the shape of the drop and provided the contact angle. Right and left contact angles of each drop were averaged to provide 1 contact angle. The solid SFE and its components were determined from the contact-angle measurements, according to the van Oss model. Each experiment was repeated 5 times for 3 specimens of each material.
Unstimulated whole saliva was collected in a chilled sterile tube with the spitting method. Saliva was collected from a healthy volunteer (S.J.A.) with good oral health, who had refrained from eating, drinking, and brushing for at least 2 hours before collection. The volunteer had no acute dental caries or periodontal lesions. Saliva collection was performed from 8:00 to 9:00 am to minimize the effects of diurnal variability in salivary composition. The saliva sample was centrifuged at 4500 g for 10 minutes to remove cellular debris. The resulting supernatants were used for adhesion assays.
Streptococcus mutans strain OMZ65 and Streptococcus sobrinus strain 6715 were used. The bacteria were stored at –70°C in brain heart infusion (Gibco, Grand Island, NY) broth containing 40% glycerol. Radiolabeling was performed by anaerobically incubating a loop of bacteria in 10 mL of brain heart infusion broth containing 50 μCi [ 3 H] thymidine ([methyl- 3 H] thymidine, Amersham Pharmacia Biotech, Piscataway, NJ) for 16 hours at 37°C. The tritium-labeled bacteria were harvested by centrifugation at 4500 g for 5 minutes and washed twice in Hank’s Balanced Salt Solution (Gibco) supplemented with 4 mmol sodium bicarbonate, 1.3 mmol calcium chloride, and 0.8 mmol magnesium chloride, (HBSS) (pH 7.2). Pellets were resuspended in HBSS and adjusted to a final concentration of 5 × 10 8 cells per milliliter by using a Petroff-Hauser cell counter (Hauser Scientific Partnership, Horsham, Pa) at A 660 .
Forty specimens of each adhesive were incubated in 2 mL of unstimulated whole saliva agitated for 2 hours at room temperature. For the negative control tests, the same procedure was performed with sterile phosphate-buffered saline solution (pH 7.2) instead of unstimulated whole saliva. After washing 3 times in sterile phosphate-buffered saline solution, saliva-coated specimens were incubated with 1 × 10 9 tritium-labeled bacteria in 2 mL of HBSS containing 0.5% bovine serum albumin (HBSS-BSA) agitated for either 3 or 6 hours at 37°C. All specimens were then washed 3 times with HBSS-BSA and transferred to scintillation vials. The radiolabeled bacteria were dislodged as described previously. Briefly, the specimens were incubated with 300 μL of 8 mol/L urea, 1 mol/L sodium chloride, and 1% sodium dodecyl sulfate agitated for 1 hour at 37°C. Then, 3.5 mL of scintillation cocktail was added, and the number of adherent cells was determined by using a liquid scintillation counter (LS-5000TA, Beckman Instruments, Fullerton, Calif). The radioactive counts were divided by the total counts per minute of the bacterial suspension solution. All test specimens were counted in triplicate, and each experiment was repeated at least 5 times.
Differences in SR and SFE and SFE components of the adhesives were analyzed by using multivariate analysis of variance (ANOVA). Four-way ANOVA was used to analyze the MS adhesion and the interaction effects caused by different strains, adhesive types, incubation times, and saliva coating. Multiple comparisons were performed by using the Bonferroni adjustment. Spearman rank correlation coefficients were calculated to analyze the relationship between surface characteristics and MS adhesion patterns. All values were considered significant at P <0.05.
Results
There were statistically significant differences in SR between the adhesives, but the mean variation of SR was relatively small (<0.05 μm) ( Table II ). The order of SR was, from the lowest to highest, Enlight and Transbond XT, Transbond Plus and Fuji Ortho LC, and Lightbond, Monolok2, and Multi-cure. There were also significant differences in SFE and its components between the adhesives, and the pattern was completely different from the SR results. A great difference in SFE and its components occurred between the RMGIs and the composites, whereas the compomer (Transbond Plus) showed an intermediate behavior. Compared with the compomer and the composites, the RMGIs showed higher SFE and γ AB . On the contrary, the composites showed lower SFE, γ AB , and γ LW than did the RMGIs and the compomer.
Composites | Compomer | RMGIs | |||||
---|---|---|---|---|---|---|---|
Enlight | Lightbond | Monolok2 | Transbond XT | Transbond Plus | Fujio Ortho LC | Multi-cure | |
Surface roughness (μm) | 0.39 ± 0.01 a | 0.44 ± 0.01 c | 0.44 ± 0.03 c | 0.39 ± 0.02 a | 0.41 ± 0.01 b | 0.40 ± 0.02 b | 0.44 ± 0.03 c |
Surface free energy (mJ/m) | 40.74 ± 1.08 b | 41.50 ± 1.09 b | 41.73 ± 1.57 b | 40.26 ± 0.92 b | 46.77 ± 0.96 c | 48.26 ± 0.85 d | 48.74 ± 1.00 d |
Dispersive component (mJ/m) | 40.19 ± 1.18 a | 40.08 ± 0.97 a | 40.80 ± 0.85 ab | 40.27 ± 1.21 a | 42.11 ± 0.73 c | 41.23 ± 0.74 b | 41.88 ± 0.52 c |
Polar component (mJ/m) | 0.56 ± 1.26 ab | 1.46 ± 1.09 b | 0.90 ± 1.39 ab | 0.22 ± 0.72 a | 4.59 ± 0.80 c | 6.98 ± 0.71 d | 6.86 ± 1.01 d |
Acid component (mJ/m) | 0.11 ± 0.13 a | 0.11 ± 0.10 a | 0.07 ± 0.12 a | 0.02 ± 0.02 a | 0.88 ± 0.32 b | 2.28 ± 0.68 d | 1.12 ± 0.47 c |
Base component (mJ/m) | 6.57 ± 1.76 b | 8.39 ± 2.78 c | 8.71 ± 1.51 c | 8.67 ± 2.02 c | 6.44 ± 1.48 b | 4.19 ± 1.03 a | 11.44 ± 2.77 d |
Table III presents the MS adhesion patterns for the adhesives, incubation times, and saliva coatings. The results indicate that 3 main factors—orthodontic adhesives, bacterial species, and incubation time—had significant effects on MS adhesion, whereas saliva coating did not significantly influence adhesion. No interaction effect was significant. With respect to bacterial species, there were significant differences in MS adhesion. Adhesion of S mutans OMZ65 to the orthodontic adhesives was greater than that of S sobrinus 6715. The adhesion of MS also varied according to the adhesive type. The order of MS adhesion was, from the lowest to highest, composites, compomer, and RMGIs. Longer incubation times increased MS adhesion, which was highest after 6 hours of incubation ( Table III ).
Adhesives (%) | |||||||||
---|---|---|---|---|---|---|---|---|---|
Composites | Compomer | RMGIs | |||||||
Strain | Saliva | Incubation time (h) | Enlight A | Lightbond A | Monolok2 A | Transbond A | Transbond B | Fujio Ortho C | Multi-cure C |
S sobrinus 6715 ∗ | Uncoated | 3 † | 0.17 ± 0.06 | 0.20 ± 0.13 | 0.15 ± 0.07 | 0.23 ± 0.14 | 0.32 ± 0.09 | 0.46 ± 0.17 | 0.40 ± 0.10 |
6 | 0.29 ± 0.15 | 0.27 ± 0.17 | 0.25 ± 0.12 | 0.36 ± 0.19 | 0.42 ± 0.11 | 0.55 ± 0.16 | 0.61 ± 0.11 | ||
Average | 0.23 ± 0.10 | 0.24 ± 0.15 | 0.20 ± 0.11 | 0.29 ± 0.14 | 0.37 ± 0.11 | 0.50 ± 0.17 | 0.50 ± 0.15 | ||
Saliva coated | 3 † | 0.17 ± 0.09 | 015 ± 0.12 | 0.22 ± 0.14 | 0.21 ± 0.10 | 0.32 ± 0.06 | 0.46 ± 0.22 | 0.50 ± 0.12 | |
6 | 0.20 ± 0.10 | 0.23 ± 0.14 | 0.22 ± 0.15 | 0.30 ± 0.14 | 0.38 ± 0.08 | 0.61 ± 0.11 | 0.51 ± 0.08 | ||
Average | 0.19 ± 0.09 | 0.19 ± 0.13 | 0.22 ± 0.14 | 0.25 ± 0.13 | 0.35 ± 0.07 | 0.52 ± 0.19 | 0.50 ± 0.10 | ||
Total | 3 † | 0.17 ± 0.08 | 0.18 ± 0.12 | 0.18 ± 0.11 | 0.21 ± 0.12 | 0.31 ± 0.07 | 0.46 ± 0.19 | 0.45 ± 0.12 | |
6 | 0.25 ± 0.16 | 0.25 ± 0.15 | 0.24 ± 0.13 | 0.33 ± 0.15 | 0.40 ± 0.09 | 0.56 ± 0.13 | 0.55 ± 0.11 | ||
Total | 0.21 ± 0.12 | 0.22 ± 0.14 | 0.21 ± 0.12 | 0.27 ± 0.14 | 0.36 ± 0.09 | 0.51 ± 0.18 | 0.50 ± 0.12 | ||
S mutans OMZ65 | Uncoated | 3 † | 0.27 ± 0.12 | 0.20 ± 0.12 | 0.22 ± 0.10 | 0.30 ± 0.14 | 0.38 ± 0.16 | 0.54 ± 0.21 | 0.54 ± 0.23 |
6 | 0.35 ± 0.15 | 0.31 ± 0.15 | 0.31 ± 0.12 | 0.38 ± 0.18 | 0.43 ± 0.16 | 0.68 ± 0.14 | 0.60 ± 0.14 | ||
Average | 0.31 ± 0.14 | 0.26 ± 0.14 | 0.26 ± 0.12 | 0.34 ± 0.14 | 0.41 ± 0.16 | 0.61 ± 0.19 | 0.57 ± 0.19 | ||
Saliva coated | 3 † | 0.28 ± 0.17 | 0.22 ± 0.15 | 0.34 ± 0.17 | 0.29 ± 0.14 | 0.37 ± 0.14 | 0.51 ± 0.18 | 0.58 ± 0.20 | |
6 | 0.40 ± 0.25 | 0.40 ± 0.16 | 0.32 ± 0.13 | 0.44 ± 0.09 | 0.41 ± 0.15 | 0.61 ± 0.09 | 0.59 ± 0.15 | ||
Average | 0.33 ± 0.21 | 0.33 ± 0.21 | 0.33 ± 0.15 | 0.36 ± 0.14 | 0.39 ± 0.15 | 0.56 ± 0.15 | 0.58 ± 0.17 | ||
Total | 3 † | 0.28 ± 0.15 | 0.21 ± 0.13 | 0.28 ± 0.15 | 0.29 ± 0.16 | 0.38 ± 0.15 | 0.52 ± 0.19 | 0.56 ± 0.21 | |
6 | 0.37 ± 0.20 | 0.36 ± 0.16 | 0.31 ± 0.13 | 0.41 ± 0.14 | 0.42 ± 0.15 | 0.64 ± 0.12 | 0.60 ± 0.14 | ||
Total | 0.32 ± 0.17 | 0.28 ± 0.16 | 0.30 ± 0.14 | 0.35 ± 0.16 | 0.39 ± 0.15 | 0.58 ± 0.17 | 0.58 ± 0.18 |
∗ Significant difference between S mutans and S sobrinus ( P <0.0001)
† significant difference between 3- and 6-hour incubation times ( P <0.0001).
The Spearman rank correlation test indicated that MS adhesion was mainly correlated to SFE, particularly, γ LW , γ AB , and γ + ( Table IV ). However, there were some differences between saliva-coated and uncoated specimens. The relationship between SFE characteristics and MS adhesion was more evident in uncoated specimens than saliva-coated specimens. After 3 hours of incubation, SFE and its components were not significantly related to MS adhesion in the saliva-coated specimens.
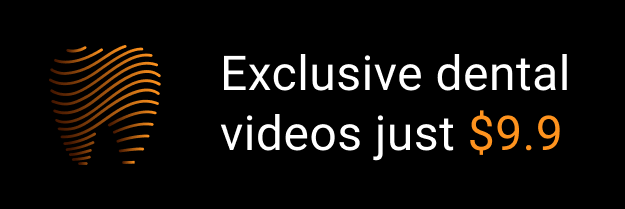