Abstract
Objectives
Selective caries removal (SCR) is recommended over non-selective removal for managing deep carious lesions to avoid pulp exposure and maintain pulp vitality. During SCR, residual carious dentin is left behind and sealed beneath the restoration. The biomechanical effects of such residual lesions on the restored tooth remain unclear and were assessed using finite element modeling (FEM).
Methods
Based on μ-CT images of a healthy permanent human third molar, we developed five finite element models. Generic class I and II cavity restorations were modeled where residual lesions of variable sizes were either left or fully removed on occlusal and proximal surfaces. The cavities were restored with adhesive composite. All 3D-FE models were compared with a model of a healthy, non-treated molar. A vertical load of 100 N was applied onto the occlusal surface.
Results
Regardless of the lesion size, in molars with occlusal lesions higher mean stresses were predicted along the filling-lesion interface than in all other models. The smallest occlusal lesion (Ø 1 = 1 mm) resulted in the highest maximum stresses at the filling-lesion interface with large stress concentrations at the filling walls indicating failure risk. In conclusion, lesion site and extent are influencing parameters affecting the filling-lesion interactions and thus the biomechanical behavior of the tooth after SCR.
Significance
Retaining carious lesions around the pulpal floor affects the deformation and stress states in tooth-filling complexes. The higher stresses observed in molars with occlusal lesions may affect restoration stability and longevity. Suprisingly, more extended occlusal lesions may provide a more favorable tooth performance than less extended ones. In contrast, in molars with proximal lesions the residual lesion had only limited effect on the tooth’s biomechanical condition.
1
Introduction
Dental caries affects more than 35% of the world population at all ages and is the most prevalent disease worldwide [ ]. Several factors, comprising the material properties of the tooth, environmental conditions in the oral cavity and a sugary diet, lead to a dysbiosis within the dental biofilm with significant growth of cariogenic bacteria [ ]. The resulting imbalance in the demineralization-remineralization dynamics affects enamel and dentin by dissolving mineral [ ]. Without arresting or reversing the process, that is without dental treatment, the demineralization leads to a deeply cavitated lesion [ ] that may reach the pulp with the risk of pulp exposure during operative treatment, or pulp inflammation, pulp necrosis and loss of vitality [ , ].
Histologically, two regions with different structural and chemical characteristics have been distinguished in carious dentin: an outer layer of contaminated dentin and an inner layer of demineralized dentin, formerly addressed as “caries-infected” and “caries-affected” dentin, respectively [ ]. The outer layer of contaminated dentin is infected by bacteria, leading to a substantial degradation of the organic matrix. This degraded matrix cannot be remineralized by physiological processes. The inner layer, in contrast, is only partially demineralized. The main difference is that the functionality of this caries-affected dentin may be re-established by a remineralization process [ , , ].
The conventional approach to treat cavitated carious lesions is non-selective caries removal (NCR), i.e. an attempted “full” removal of any carious dentin until only hard, healthy dentin remains, followed by the restoration of the cavity [ , ]. This procedure is associated with several risks like over-preparation of the cavity into healthy tooth tissue [ , ] which may expose the pulp [ , ] and weaken the remaining tooth structure [ ].
To overcome these disadvantages and risks, selective caries removal (SCR) has been proposed as a minimally invasive treatment for managing deeply cavitated lesions. During this treatment, bacterially infected dentin is excavated from the peripheral walls of the cavity, while so-called residual, demineralized, dentin is left in direct proximity of the pulp, and subsequently sealed beneath the filling. The procedure mainly aims at reducing the risk of pulp exposure and the related complications [ , , , ]. Sealing of carious dentin deprives bacteria of their source of nutrition so that they become inactive [ , ]. This step arrests the progress of caries, and it induces remineralization of the dentin [ ]. Further, the remains of caries-affected dentin have been reported to preserve pulp vitality [ ].
Although there is sound scientific evidence supporting SCR to reduce pulpal risks [ , , ], there are uncertainties towards the impact of carious dentin remaining beneath the filling on both the biomechanical behavior and the fracture resistance of the tooth-filling complex. These concerns arise from the notion that caries-affected dentin is less mineralized and thus more compliant than healthy dentin [ , , , , ].
However, in various biological tissues soft inclusions have proven to be beneficial to the structure’s strength and toughness [ , ]. From this perspective, some authors claim that the integrity of the restauration in SCR treated teeth is not affected by the presence of caries-affected dentin [ , , , ]; others have shown cracks in fillings after SCR due to the filling not being sufficiently supported by the underlying residual carious dentin. This may lead to an increased risk of filling fracture during mastication [ ]. While these results help us understand the implications of SCR on the mechanical stability of treated teeth, none of these studies can provide information on the stress and strain distribution within the tooth-filling complex induced by the carious lesion size and site, nor the stresses required to cause fracture. Here, state of the art modelling tools may provide useful insights.
Finite element modeling (FEM) is a non-invasive computational method which allows us to predict the biomechanical state of a restored tooth, comprising the stress distribution and stress concentrations induced by a defined external mastication force, in the different tooth tissues as well as within the dental materials. The mechanical stresses are relevant indicators where damage initiation is to be expected in a structure for a defined loading state, thereby clarifying the mechanical impact of dental procedures, specifically on whether or to what degree these procedures may weaken teeth [ ]. However, FE models are often simplified in terms of tooth geometry, material definition and loading conditions (e.g. [ ]). Their accuracy strongly depends on precise input data: nowadays, exact geometries and structural data are available from X-ray computed tomography (CT) or micro-computed tomography (μCT) scans of real teeth [ , , ]. Several studies have addressed the biomechanical impact of different restorative materials [ , ], whereas others have focused on design aspects of the cavity [ , ]. As to be expected, imperfections in dental materials act as stress raisers [ ], thus contributing to failure by facilitating crack initiation [ ]. To the best of our knowledge, no work has been performed so far on the biomechanical influence of SCR treatment using FEM. Specifically, the stress concentrations around and at the interface between carious dentin and filling have not been investigated yet.
We, therefore, investigated the biomechanical behavior of an SCR treated molar by means of FEM, in comparison to an NCR treated molar and a healthy, non-treated molar. We present displacement and stress distributions at the interface between filling and residual lesion. We further examined the influence of lesion size and anatomical site on the occurrence of stress concentrations in SCR treated teeth.
2
Materials and methods
2.1
Sample preparation and 3D imaging
An extracted, healthy human upper third molar was used to create various FE models. The sample was obtained under an ethics protocol approved by the ethics committee of the Charité – Universitätsmedizin Berlin EA4/102/14. Micro-computed tomography images were obtained using a SKYSCAN 1172 μCT (Bruker, Billerica, USA) with an effective isotropic pixel resolution of 13.21 μm. The X-ray voltage and the current were set at 80 kV and 124 μA, respectively. Image data were visualized using VGSTUDIO (v3.0, Volume Graphics, Heidelberg), cropped, and downscaled to obtain isotropic 20.97 × 20.97 × 20.97 μm³ voxels. Image data were visualized as grey-scale patterns. Based on the characteristic linear attenuation coefficients and geometries, enamel and dentin were clearly identified so that both tooth tissues of the molar could be segmented into two volumes. The remaining tooth tissues, for instance the periodontal ligament, were not considered in this study.
2.2
Finite element modeling
Image data were imported into an open source software ISO2MESH (Fang, Q., Northeastern University, Boston, USA) and converted into surface elements. The segmented data of enamel and dentin were smoothed and meshed with volumetric tetrahedral elements in HYPERMESH (v.17, Altair Engineering Inc. Troy, Michigan, USA). We created four FE models of a restored molar with different modifications. Two of these FE models represented selective caries removal (SCR) and the other two FE models emulated a restored molar which had undergone non-selcteive caries removal (NCR). For this purposed, we modified the tooth with residual lesions by replacing the residual caries lesion with filling material. We introduced class I and class II preparations. The residual lesion was manually designed in two different anatomical sites of the molar tooth, situated either occlusally or proximally (see Fig. 1 a-b). The residual lesion was modeled as a truncated multilayered cone positioned towards dentin and characterized by two geometric parameters: the largest extent Ø i and the maximum depth d i . An addtional FE model of a healthy molar was created for comparison.

The following summarizes all five FE models, each with the given modifications:
- a)
SCR-O: SCR treated molars with occlusal lesions beneath a filling centered in the enamel-dentin volume. The residual lesion never reached the pulp, independent of its size ( Fig. 1 a).
- b)
SCR-P: SCR treated molars with proximal lesions in dentin and adjacent to the filling on the mesial side. The residual lesion never reached the pulp, independent of its size ( Fig. 1 b).
- c)
NCR-O: NCR treated molar with an occlusal filling centered in the enamel-dentin volume ( Fig. 1 c).
- d)
NCR-P: NCR treated molar with a proximal filling situated on the mesial side ( Fig. 1 d).
- e)
H: healthy molar without a dental restoration ( Fig. 1 e).
An additional 2D FE model representing a mesio-distal section of the SCR-O model was used to study the influence of the ratio of lesion to filling extent on the local stress distribution.
2.2.1
Boundary conditions
All FE models were imported to the ABAQUS/CAE 2017 software (Dassault Syst è mes Simulia, Johnston, RI, USA). For all simulations, the entire dentin surface in the root area was fixed in all directions. Comparison with a model where the root was fixed in the load direction showed no significant difference in the deformation and stress state within the crown (data not shown). This prevented any translatory and rotatory movements. All material interfaces were assumed fully bonded. A vertical force of 100 N was assumed to be a typical masticatory load scenario (see summary in [ ]) which was applied onto the occlusal surface of the crown acting in occluso-apical direction (z-axis) ( Fig. 1 f). The load position and direction were identical for all simulations.
2.2.2
Material properties for tooth tissues, residual lesion and filling
Enamel, dentin, and filling material were assumed to be isotropic, homogeneous, and linear-elastic in all FE models. Young’s moduli of enamel and dentin have been reported to span 80–120 GPa [ , ] and 18.1–21.6 GPa [ ], respectively. We calculated averages from linear interpolation between the minimum and maximum values and used these for our FE analysis ( Table 1 ) . While the elastic material properties of healthy tooth tissues are well investigated, less certainty exists regarding the structural heterogeneity and elastic mechanical properties of the residual carious lesion [ ]. We, therefore, modelled the residual lesion as a four-layered material system ( Fig. 1 a, b) with different material properties in each layer (i.e. variable mineral density, Table 1 ). According to literature [ , , ], the outermost layer, L 1 , is the most compliant one. Thus, stiffness and strength increase towards the fourth layer, L 4 , i.e. closest to dentin ( Table 1 ). For the second and the third layer, L 2 and L 3 , the values were calculated by linear interpolation between the values for L 1 and L 4 . To represent the very thin residual lesion with a depth of 0.25 mm, we used only two layers with the properties of L 1 and L 3 of the four-layered residual lesion ( Table 1 ). For the filling material, we assumed a resin-based composite [ ].
Young’s modulus E [MPa] | Poisson‘s ratio [-] | |
---|---|---|
enamel | 100000 | 0.30 |
dentin | 19800 | 0.31 |
filling | 15000 | 0.30 |
residual lesion: | ||
L 1 | 1600 | 0.35 |
L 2 | 6150 | 0.34 |
L 3 | 10700 | 0.33 |
L 4 | 15250 | 0.32 |
FEM calculations were conducted by the commercial solver ABAQUS STANDARD 2017 (Dassault Syst è mes Simulia, Johnston, RI, USA) at the North German Supercomputing Alliance hardware (HLRN, Zuse Institute, Berlin, Germany). The FE models contained on average 10 7 elements. In the volume of the residual lesion, we used a very fine mesh with a mesh element size of ∼100 μm. The convergence of the mesh was successfully tested for all models.
3
Results
3.1
Displacements at the interface between filling and lesion
Fig. 2 shows color-coded plots of the displacements induced within the SCR treated teeth with occlusal lesions (SCR-O) in comparison with the NCR treated molar (NCR-O) and a healthy, non-treated molar (H). The maximum displacements in the occluso-apical (z-) direction occurred in the central crown region in all models. The maximum values in SCR-O and NCR-O treated teeth were similar (+16 μm), but four times higher than in the healthy molar (H). In the mesio-distal (y-) and bucco-lingual (x-) directions, in contrast, the predicted displacements were smaller, while again SCR-O and NCR-O treated teeth ( Fig. 2 a-i) showed higher displacements than the healthy molar ( Fig. 2 j–l). In the bucco-lingual direction, we observed the smallest displacements spanning 0.1 μm (H) to 0.7 μm (SCR-O and NCR-O).

In SCR treated teeth with proximal lesions (SCR-P) ( Fig. 3 ), the displacements were in general smaller than those in the teeth with occlusal lesions (less than 0.3 μm in all directions). Absolute differences between groups were hence also smaller.

3.2
Stress response at the interface between filling and lesion
Von Mises stress distributions were evaluated to identify regions prone to failure. According to the von Mises yield criterion, structures fail when the von Mises stress reaches a specific value, e.g. the tensile yield strength of the filling material (appr. 15 MPa). The von Mises stresses induced within the filling at the interface between the filling and the residual lesion in the SCR treated teeth are shown in Figs. 4 to 6 .
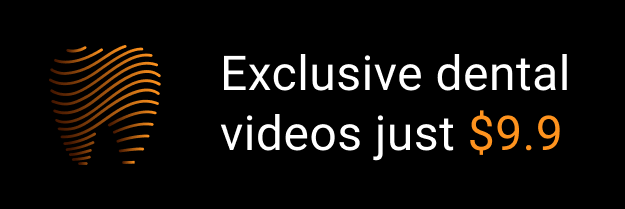