Graphical abstract

Highlights
- •
Sol–gel derived bioactive glass nanoparticles (BGNs) were successfully fabricated with ∼42 nm in diameter with bioactivity.
- •
The incorporation of BGNs into glass ionomer cement (GIC) led to increased mechanical and bioactive properties.
- •
The incorporation of BGNs into GIC led to increased biomineralization with human dental pulp stem cells without cytotoxicity.
- •
The developed BGNs-incorporated GIC is considered as a promising restorative dental material.
Abstract
Objective
This study investigated the mechanical and in vitro biological properties (in immortalized human dental pulp stem cells (ihDPSCs)) of bioactive glass nanoparticle (BGN)-incorporated glass ionomer cement (GIC) with or without chitosan as a binder.
Methods
After the BGNs were synthesized and characterized, three experimental GICs and a control (conventional GIC) that differed in the additive incorporated into a commercial GIC liquid (Hy-bond, Shofu, Japan) were produced: BG5 (5 wt% of BGNs), CL0.5 (0.5 wt% of chitosan), and BG5 + CL0.5 (5 wt% of BGNs and 0.5 wt% of chitosan). After the net setting time was determined, weight change and bioactivity were analyzed in simulated body fluid (SBF) at 37 °C. Mechanical properties (compressive strength, diametral tensile strength, flexural strength and modulus) were measured according to the incubation time (up to 28 days) in SBF. Cytotoxicity (1 day) and biomineralization (14 days), assessed by alizarin red staining, were investigated using an extract from GIC and ihDPSCs. Data were analyzed using one-way analysis of variance (ANOVA) followed by Tukey’s post hoc test; p < 0.05.
Results
BGNs were sol–gel synthesized to be approximately 42 nm in diameter with a spherical morphology and amorphous structure. After the bioactivity and suspension ability of the BGNs were confirmed, all the experimental GIC groups had setting times of less than 6 min and approximately 1% weight loss after 28 days of incubation. In addition, BGNs incorporated into GIC (BG5 and BG5 + CL0.5) exhibited surface bioactivity. The mechanical properties were increased in the BGN-incorporated GICs compared to those in the control (p < 0.05). Without cytotoxicity, the biomineralization capacity was ranked in the order BG5, BG5 + CL0.5, control, and CL0.5 (p < 0.05).
Significance
BGN-incorporated GIC showed enhanced mechanical properties such as compressive, diametral tensile and flexural strength as well as in vitro biomineralization properties in ihDPSCs without cytotoxicity. Therefore, the developed BGN-incorporated GIC is a promising restorative dental material, although further in vivo investigation is needed before clinical application.
1
Introduction
Glass ionomer cement (GIC) is a tooth-colored material introduced by Wilson and Kent in the late 1960s. GIC is set based on a reaction between a fluoroaluminosilicate-based glass powder and an acidic liquid primarily composed of polyacrylic acid. These substances bond chemically to the tooth structure via a carboxyl (from GIC)-calcium (from enamel or dentin) interaction and release fluoride ions for a relatively long period, which is a beneficial anticariogenic property . Along with the above advantages, GIC has been used as luting cement, a restorative material or a base and liner before a final direct or indirect restoration.
Although GIC has a high compressive strength after setting, it is vulnerable to tension and shear force and consequently shows lower flexural and diametral tensile strength . Therefore, increasing its mechanical properties using additives has been investigated. Metal alloy (i.e., silver–tin or silver–palladium/titanium) has been successfully incorporated into glass powder to enhance the mechanical properties, but the bioactivity was compromised with a change of color to an unaesthetic metal color .
In 1969, Hench reported that certain degradable glass compositions (Bioglass 45S5) were able to form chemical bonds with bone due to released ions such as Na + , Si 4+ and Ca 2+ . These materials are referred to as bioactive glasses and have been used as regenerative biomaterials for damaged hard tissues, including teeth. In dentistry, bioactive glass has been widely investigated as a bone graft material or additive to restorative materials, cement and bonding agents for enhancing bioactivity . In terms of its application in GIC (including GIC derivatives), commercially available bioactive glasses (NovaBone and S53P4) containing (10–20%) GIC or air abrasion with bioactive glass (45S5) before resin-modified GIC application have been reported to exhibit enhanced bioactivity in simulated body fluid and bonding durability, thus enabling re-mineralization of human dentin . However, the addition of bioactive glass into GIC decreased its compressive strength, flexural strength and surface hardness . Thus, developing bioactive GIC without decreasing its mechanical properties remains a challenge.
Due to their high surface area, nanoparticles are of special interest for applications to dental materials including GIC to enhance the mechanical properties of the matrix and enhance communication with dental tissue-derived cells to aid their regeneration . In addition to many nanoadditives (i.e., hydroxy(or fluoro)apatite, titanium oxide, zirconia, resin, and their combinations) that have been incorporated into conventional GIC, one of the promising nanoparticulates for use in GIC is bioactive glass nanoparticles (BGNs) .
BGNs increase the surface area and bioactivity when combined with the matrix in GIC and thereby provide a greater enhancement of mechanical/biological properties as an additive per particle weight than conventional micro-sized bioactive glass particles . Since BGNs were first produced using the sol–gel method with the development of nanotechnology, the application of BGNs has been diversified to overall dentistry due to their enhanced bioactivity and mechanical properties as a nanocomposite . However, no studies have evaluated the effect of BGNs incorporated into GIC on the GIC’s mechanical properties (i.e., compressive, flexural and diametral tensile strength) and biological effects (i.e., cytotoxicity and biomineralization).
Chitosan, a linear polysaccharide composed of randomly distributed β-(1-4)-linked d -glucosamine and N -acetyl- d -glucosamine, has been used in the dental (or biomedical) field due to its natural adhesive properties, biocompatibility, antibacterial properties, and pH-sensitive solubility . Chitosan has also been incorporated into GIC to enhance its mechanical properties by acting as a physical or chemical binder between the glass filler and matrix in GIC or to induce antibacterial effects , depending on the concentration of chitosan in GIC.
In the present study, the mechanical and biological properties of BGN ( ϕ = ∼42 nm)-incorporated GIC with or without chitosan were investigated in vitro study (immortalized human dental pulp stem cells (ihDPSCs)) to support further study in vivo. The null hypothesis is that there is no significant difference in the mechanical and biological properties of GIC with or without BGNs or chitosan.
2
Materials and methods
2.1
Fabrication of bioactive glass nanoparticle
Tetraethyl orthosilane (TEOS), calcium nitrate tetrahydrate (Ca(NO 3 ) 2 ·4H 2 O), ammonia solution (28%) and Pluronic 123 (P123) were used as precursors for the synthesis of BGNs (85% SiO 2 –15% CaO). All chemicals were purchased from Sigma–Aldrich unless otherwise stated. Spherical bioglass nanoparticles containing 85SiO 2 /15CaO (mol%) were synthesized via a base-catalyzed sol–gel approach in the presence of templates according to our previously reported method . In a 150-mL solution that contained 100 mL of distilled water (DW), 25 mL of ethanol, and 25 mL of ammonia solution (28%), 1 g of calcium nitrate (4.23 mM) and 10 g of P123 were dissolved at 50 °C under mechanical stirring at 500 rotations per minute (rpm) for 30 min. After the pH was adjusted to 12.5 by adding NH 4 OH, 1 g of Ca(NO3) 2 ·4H2O was dissolved in the above transparent solution. In another vessel, 5.36 mL of TEOS (24 mM) was diluted in 40 mL of absolute methanol and slowly added to the pH 12.5 solution prepared above while applying high-power ultra-sound in a sonoreactor (LH700S ultra-sonic power generators, Ulsso Hitech, Cheongwon, South Korea) for 30 min at 20 kHz and 700 W. After maintaining mechanical stirring overnight, the milky BGNs (white precipitate) were collected by centrifugation at 5000 rpm. The BGNs were serially washed with distilled water (DW), acetone, and 70% ethanol. The fine BGNs were dried at 70 °C overnight and finally calcined at 600 °C for 5 h with a constant (1°/min) heating rate. The yield of BGNs from the above process was 1.35 g.
2.2
Characterization of bioactive glass nanoparticles
The surface morphology of the BGNs was examined with a scanning electron microscope (SEM; Sigma 500, ZEISS, Jena, Germany) at 3 kV, and the elements were analyzed by energy-dispersive spectroscopy (EDS, UltraDry, Thermo Fisher, Waltham, MA, USA) at 15 kV after plasma coating for 5 min using an ion sputterer (IB-3 Eiko, Tokyo, Japan) according to a previous procedure . The particle size and morphology of the BGNs were investigated by transmission electron microscopy (TEM; 7100, JEOL, Japan) . The density was measured with a microbalance (AUW220D; Shimadzu, Kyoto, Japan) with 0.01-mg accuracy for weight and a gas pycnometer (AccuPyc 1330, Shimadzu, Kyoto, Japan) with 0.02% accuracy for 1 cm 3 according to the previous procedures . The phase of the nanoparticles was analyzed with X-ray diffraction (XRD, Rigaku, Ultima IV, Japan) using CuKα radiation (λ = 1.5418 Å) . X-rays were generated at 40 kV and 40 mA, and two theta (2θ) was obtained from 4 to 70° with a step size of 0.02°. The in vitro apatite-forming ability of the BGNs was tested in simulated body fluid (SBF) at 37 °C. The SBF was prepared by dissolving NaCl (142.0 mM), KCl (5.0 mM), NaHCO 3 (4.2 mM), CaCl 2 (2.5 mM), MgCl 2 ·6H 2 O (1.5 mM), K 2 HPO 4 ·3H 2 O (1.0 mM), and Na 2 SO 4 (0.5 mM) in DW buffered to pH 7.4 with Tris–HCl. The ion concentrations of the SBF solution were nearly equal to those of human body blood plasma. Ten milligrams of BGNs was immersed in 10 mL of SBF for up to 28 days. The SBF solution was replaced with fresh solution every 7 days. The phase of the nanoparticles after immersion in SBF was analyzed with X-ray diffraction using the same conditions described above. Zeta potential (ζ) measurements were carried out using a Zetasizer (ZEN 3600, Malvern, Worcestershire, UK) at 25 °C based on dynamic light scattering. BGNs incorporated into the DW solution (50 mg/mL) at 5 wt% were placed in disposable polystyrene cuvettes for analysis . The stability of nanoparticles in media was determined at 25 °C using Turbiscan (LAB Science), which scanned the turbidity change from the top of the cuvette (40 mm) to the bottom (0 mm) with transmittance (%) and backscattering (%) for 24 h at 1 h intervals . BGNs incorporated into the DW solution (200 μg/mL, 40 mL) were prepared as a representative concentration for investigating the general dispersion ability of BGNs in liquid for 24 h. Three replicate tests were carried out for each assay, and representative data and images are shown.
2.3
Fabrication of glass ionomer cement
We prepared four different groups of GIC according to the Table 1 : Control, CL0.5 (chitosan low-molecular-weight powder 0.5%), BG5 (BGNs 5%), and BG5 + CL0.5 (BGNs 5% + chitosan low-molecular-weight powder 0.5%). As the control, commercial GIC (HY-BOND GLASIONOMER CX, Shofu, Kyoto, Japan) was used. For CL0.5, low-molecular-weight chitosan powder (50–190 kDa, 75–85% deacetylation, Sigma, St. Louis, Missouri, USA) was added at 0.5 wt% into the provided liquid (lot number: 050904) of control cement, including water (40–50%), poly (acrylic acid) (40–50%) and tartaric acid (1–10%) . For BG5, BGNs were added to the cement liquid at 5.0 wt%. For BG5 + CL0.5, both chitosan powder (0.5 wt%) and BGNs (5 wt%) were dissolved in the cement liquid. After the incorporation of the additive into the liquid, the liquid was sonicated and vortexed for 1 min each for homogenization. Homogenization of the additives in liquid was achieved immediately before mixing with powder. Before cementation, the homogeneity of the liquid was checked visually with a light microscope. The GIC was hardened according to the manufacturer’s instructions. Briefly, the mixing ratio for the cement was set at 2:1 for powder (lot number: 051162), including fluoroaluminosilicate glass (>90%), to liquid as wt% and they were mixed for 30 s. All steps were performed at a clean bench to prevent contamination.
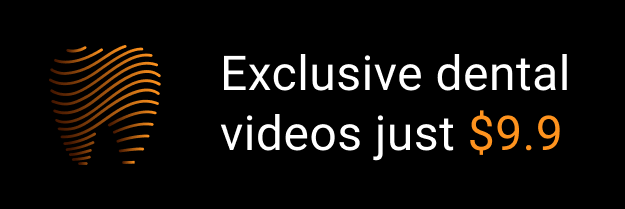