Graphical abstract

Highlights
- •
A 10 μm thick altered zone is present under the airborne-particle abraded 3Y-TZP surface.
- •
Ageing penetrates through the altered zone and decreases the surface strengthening.
- •
When the altered zone is annihilated, strength returns to values measured in intact 3Y-TZP.
- •
Ageing of airborne-particle abraded 3Y-TZP follows a two-step linear kinetics.
- •
Airborne-particle abrasion is not harmful to the 3Y-TZP ceramics’ stability.
Abstract
Objective
The combined effects of alumina airborne-particle abrasion and prolonged in vitro ageing on the flexural strength of 3Y-TZP ceramic have been studied. The aim was to identify the different effects on the surface and subsurface regions that govern the performance of this popular bioceramic known for its susceptibility to low-temperature degradation (LTD).
Methods
As-sintered or airborne-particle abraded 3Y-TZP discs were subjected to ageing at 134 °C for up to 480 h. Biaxial flexural strength was measured and the relative amount of monoclinic phase determined using X-ray diffraction. The transformed zone depth (TZD) was observed on cross-sections with scanning electron microscopy coupled with a focused ion beam. Segmented linear regression was used to analyze the flexural strength and TZD as functions of the ageing time.
Results
A two-step linear ageing kinetics was detected in airborne-particle abraded specimens, reflecting the different microstructures through which the LTD proceeds into the bulk. A 10 μm thick altered zone under the abraded surface was involved in both the surface strengthening and the increased ageing resistance. When the zone was annihilated by the LTD, the strength of the ceramic specimens and the speed of LTD returned to the values measured before abrasion. Even at prolonged ageing times, the strength of abraded groups was not lower than that of as-sintered groups.
Significance
Both the ageing kinetics and the flexural strength were prominently affected by airborne-particle abrasion, which altered the subsurface microstructure and phase composition. Airborne-particle abrasion was not harmful to the 3Y-TZP ceramics’ stability.
1
Introduction
Tetragonal zirconia polycrystalline ceramic, which is partially stabilized by yttria (3Y-TZP), is highly regarded for its combination of excellent mechanical properties and its enhanced aesthetic appearance compared to metals. It is a popular choice for all-ceramic dental restorations . Its high strength and toughness are achieved through tetragonal-to-monoclinic ( t – m ) transformation. Under stress, parts of the metastable tetragonal grains transform martensitically to the stable monoclinic variants. Because the associated volume increases, compressive stresses are developed, inhibiting the growth of cracks, thus resulting in toughening of the structure .
The undesirable side effect of the metastability of 3Y-TZP ceramic is a spontaneous phase transformation in the presence of moisture . The process is also known as ageing or low-temperature degradation (LTD) and it is accompanied by micro-cracking, grain pull-out and roughening of the surface, with potentially harmful deterioration of the mechanical characteristics and ultimately affecting the material’s reliability. Efforts are in progress to control the LTD by using dopants, reducing grain sizes, modifying the surface or using composite materials . However, preventing LTD is possible and is usually achieved by strong stabilization of the material, but with an inevitable trade off—decrease in its ability to undergo a t – m transformation impairs the mechanical properties due to lack of toughening. Alternatively, chemical modification using alternative dopants might also result in a lower flexural strength through coarsened microstructure, as evident from ceria-stabilized zirconia . Both stress- and moisture-induced transformations are integral to 3Y-TZP ceramics, but an understanding of the underlying mechanisms in connection with the microstructure and the mechanical properties has yet to be achieved.
The combination of stress- and moisture-induced transformations of the 3Y-TZP ceramics can be expected clinically, where dental restorations are subjected to hydrothermal and mechanical stresses. The ceramic surfaces are commonly treated mechanically by grinding and fine polishing to adjust occlusion, and by airborne-particle abrasion to improve bonding of the dental restorations . The roughened surfaces increase the mechanical interlocking of the luting agent, albeit at the expense of introducing potentially damaging surface flaws. Moreover, although the surface is damaged, the flexural strength actually increases through the substantial effect of surface strengthening . Surface strengthening is the increase in strength due to techniques placing the surfaces into compression . In case of metastable tetragonal zirconia, strengthening by surface damage has been described both for grinding and airborne-particle abrasion . In addition, the ageing process is apparently delayed if the surface has been mechanically treated, which could be due to the residual surface compressive stresses , partitioning of the surface tetragonal grains , or to formation of a thin layer of recrystallized and textured nano-size grains that prevent the formation of monoclinic variants .
The progress of the LTD has been the focus of intensive research and it was recently demonstrated that the degradation penetrates the tetragonal bulk linearly . The situation in zirconia fixed dental prostheses is more complex. Commonly used preparation techniques namely alter the microstructure on and below the immediate surface. The nature and extent of phase transformation upon mechanical stress is still an unresolved question. X-ray diffraction (XRD) readily discerns the monoclinic phase upon LTD , but not upon mechanical damage , and there is no correlation between the amount of monoclinic phase and strength . Additional crystallographic phenomena that were ascribed tentatively to the appearance of rhombohedral phase and ferroelastic domain switching, have all been linked to the mechanical or thermal treatments of 3Y-TZP ceramic surfaces . Neither the reason for the remarkable surface strengthening nor the resistance of the strengthened surfaces to LTD have been fully clarified. The less-studied phase transformation effects might have an important role in understanding the material’s long-term behavior and mechanical properties when subjected to hydrothermal conditions triggering the LTD, ultimately affecting the materials’ reliability.
We have investigated the combined effect of alumina airborne-particle abrasion and prolonged in vitro ageing on the flexural strength of 3Y-TZP ceramic in order to differentiate between their distinctive effects on the ceramic surface and on the subsurface region that ultimately determines the overall performance and reliability of this popular bioceramic. The aim was to determine how the microstructure altered by airborne-particle abrasion changes the LTD kinetics and how the degradation affects the bulk mechanical strength.
2
Materials and methods
2.1
Specimen preparation
Materials were prepared from commercially available, biomedical grade 3Y-TZP granulated powder containing 3 mol% of yttria, 0.25 wt.% of alumina and 3 wt.% of an organic binder (TZ-3YB-E, Tosoh, Japan). 240 specimens were dry pressed into discs uniaxially at 150 MPa and sintered in a conventional resistance oven at 1500 °C for 2 h in air with heating and cooling rates of 5 °C/min. After sintering, the final diameter of the discs was 15 mm and their thickness 1.5 mm. The grain size was estimated from field emission scanning electron microscopy (FE-SEM) images (GeminiSEM, Carl Zeiss AG, Jena, Germany) using the linear-intercept procedure based on the ASTM E112-13 standard without introducing any correction factors . The specimen surfaces were mirror polished, thermally etched (1400 °C for 30 min in air) to expose the grain boundaries and examined without any surface coating applied.
The relative density was determined by the Archimedes’ method, using deionised water as the immersion liquid. Even though the specimens were sintered in the two-phase ( t + c ) region, a theoretical density of ρ T = 6.08 g/cm 3 for the tetragonal phase was used in the calculations. Vickers indentation tests with a load of 100 N and 10 s dwell time were used to measure fracture toughness. An average value from 10 indentations was obtained.
2.2
Experimental protocol and characterizations
The specimens were divided randomly into two groups of 120 specimens each. One group was left in the as-sintered state, the other was airborne-particle abraded with 110 μm Al 2 O 3 particles at a pressure of 2.5 bar, using an air-abrasion unit (Basic IS, Renfert Dental, Hilzingen, Germany). The discs were abraded on one side only and then cleaned ultrasonically in acetone for 10 min. Twenty-four groups of 10 samples were formed and subjected to accelerated ageing in vitro , using deionised water at 134 °C. Both as-sintered and airborne-particle abraded groups were aged for up to 20 days, with twelve ageing times: 0 h, 12 h, 24 h, 48 h, 72 h, 96 h, 120 h, 144 h, 192 h, 288 h, 384 h and 480 h. X-ray diffraction patterns (XRD) were collected from three randomly selected samples from each group, using Cu-Kα radiation at 45 kV and 40 mA over the range of 25–40° 2 θ (X’Pert PRO X-Ray diffractometer, PANalytical, Almelo, The Netherlands). The amount of monoclinic zirconia ( Xm ) was estimated using the Garvie and Nicholson method . The biaxial flexural strength was determined after each time-point of accelerated ageing using a universal material-testing machine (Quasar 100, Galdabini, Cardano al Campo, Italy). The experimental setup consisted of a piston-on-3-balls fixture with crosshead speed set to 1 mm/min. The airborne-particle-abraded specimens were loaded with the treated side under tension. The load at fracture was recorded in newtons (N) and the strength calculated according to Wachtman et al. .
The depth of the transformed zone (TZD) after ageing was observed on cross-sections prepared by grinding with 2400 grit silicon carbide grinding paper (Struers, Ballerup, Denmark), using optical microscopy (Axio Imager 2, Carl Zeiss AG, Jena, Germany) to ensure the correct mounting position in the metallographic resin and the achieval of necessary contrast between the transformed and untransformed portions. Two cross-sections were prepared for each group and coated with carbon prior to examination with FE-SEM (GeminiSEM, Carl Zeiss AG, Jena, Germany) to determine the TZD. On each cross-section, 5 measurements of the TZD were taken to obtain the average value.
Further insight into the subsurface transformation zone before and after airborne-particle abrasion and LTD was obtained using the SEM coupled with a focused ion beam (FIB) (Helios Nanolab 650, FEI, Hillsboro, USA). A 0.5 μm layer of platinum film was sputtered on the area of interest, using the ion-beam-assisted gas injection system at 30 kV and 0.43 nA to prevent the extensive curtain effect. FIB trenches were cut with ion beam machining at 30 kV and 65 nA and finalized by ion polishing at 30 kV and 21 nA. The cross-sections were observed in situ , at an angle of 52°, using the electron probe at 2 kV and 100 pA.
2.3
Statistical analysis
Statistical analyses were conducted with the statistics software package R 3.1.2 . The “segmented” package was used to fit the segmented linear regression to the flexural strength and to TZD data as functions of the accelerated ageing time. Standard errors and confidence intervals were computed with the delta method and Davies’ test was used to determine the presence of changes in the slopes . Asymptotic regression was used to model the XRD Xm data as a function of the accelerated ageing time. The significance level was set to α = 0.05.
2
Materials and methods
2.1
Specimen preparation
Materials were prepared from commercially available, biomedical grade 3Y-TZP granulated powder containing 3 mol% of yttria, 0.25 wt.% of alumina and 3 wt.% of an organic binder (TZ-3YB-E, Tosoh, Japan). 240 specimens were dry pressed into discs uniaxially at 150 MPa and sintered in a conventional resistance oven at 1500 °C for 2 h in air with heating and cooling rates of 5 °C/min. After sintering, the final diameter of the discs was 15 mm and their thickness 1.5 mm. The grain size was estimated from field emission scanning electron microscopy (FE-SEM) images (GeminiSEM, Carl Zeiss AG, Jena, Germany) using the linear-intercept procedure based on the ASTM E112-13 standard without introducing any correction factors . The specimen surfaces were mirror polished, thermally etched (1400 °C for 30 min in air) to expose the grain boundaries and examined without any surface coating applied.
The relative density was determined by the Archimedes’ method, using deionised water as the immersion liquid. Even though the specimens were sintered in the two-phase ( t + c ) region, a theoretical density of ρ T = 6.08 g/cm 3 for the tetragonal phase was used in the calculations. Vickers indentation tests with a load of 100 N and 10 s dwell time were used to measure fracture toughness. An average value from 10 indentations was obtained.
2.2
Experimental protocol and characterizations
The specimens were divided randomly into two groups of 120 specimens each. One group was left in the as-sintered state, the other was airborne-particle abraded with 110 μm Al 2 O 3 particles at a pressure of 2.5 bar, using an air-abrasion unit (Basic IS, Renfert Dental, Hilzingen, Germany). The discs were abraded on one side only and then cleaned ultrasonically in acetone for 10 min. Twenty-four groups of 10 samples were formed and subjected to accelerated ageing in vitro , using deionised water at 134 °C. Both as-sintered and airborne-particle abraded groups were aged for up to 20 days, with twelve ageing times: 0 h, 12 h, 24 h, 48 h, 72 h, 96 h, 120 h, 144 h, 192 h, 288 h, 384 h and 480 h. X-ray diffraction patterns (XRD) were collected from three randomly selected samples from each group, using Cu-Kα radiation at 45 kV and 40 mA over the range of 25–40° 2 θ (X’Pert PRO X-Ray diffractometer, PANalytical, Almelo, The Netherlands). The amount of monoclinic zirconia ( Xm ) was estimated using the Garvie and Nicholson method . The biaxial flexural strength was determined after each time-point of accelerated ageing using a universal material-testing machine (Quasar 100, Galdabini, Cardano al Campo, Italy). The experimental setup consisted of a piston-on-3-balls fixture with crosshead speed set to 1 mm/min. The airborne-particle-abraded specimens were loaded with the treated side under tension. The load at fracture was recorded in newtons (N) and the strength calculated according to Wachtman et al. .
The depth of the transformed zone (TZD) after ageing was observed on cross-sections prepared by grinding with 2400 grit silicon carbide grinding paper (Struers, Ballerup, Denmark), using optical microscopy (Axio Imager 2, Carl Zeiss AG, Jena, Germany) to ensure the correct mounting position in the metallographic resin and the achieval of necessary contrast between the transformed and untransformed portions. Two cross-sections were prepared for each group and coated with carbon prior to examination with FE-SEM (GeminiSEM, Carl Zeiss AG, Jena, Germany) to determine the TZD. On each cross-section, 5 measurements of the TZD were taken to obtain the average value.
Further insight into the subsurface transformation zone before and after airborne-particle abrasion and LTD was obtained using the SEM coupled with a focused ion beam (FIB) (Helios Nanolab 650, FEI, Hillsboro, USA). A 0.5 μm layer of platinum film was sputtered on the area of interest, using the ion-beam-assisted gas injection system at 30 kV and 0.43 nA to prevent the extensive curtain effect. FIB trenches were cut with ion beam machining at 30 kV and 65 nA and finalized by ion polishing at 30 kV and 21 nA. The cross-sections were observed in situ , at an angle of 52°, using the electron probe at 2 kV and 100 pA.
2.3
Statistical analysis
Statistical analyses were conducted with the statistics software package R 3.1.2 . The “segmented” package was used to fit the segmented linear regression to the flexural strength and to TZD data as functions of the accelerated ageing time. Standard errors and confidence intervals were computed with the delta method and Davies’ test was used to determine the presence of changes in the slopes . Asymptotic regression was used to model the XRD Xm data as a function of the accelerated ageing time. The significance level was set to α = 0.05.
3
Results
3.1
Characteristics of the materials
The microstructure of the as-sintered 3Y-TZP surface is presented in Fig. 1 . The final grain size was 0.40 ± 0.04 μm in diameter. Several darker alumina grains were present in the microstructure. The relative density exceeded 99% of the theoretical value and the measured indentation toughness was 4.84 ± 0.17 MPa m −1/2 .
3.2
Depth of the transformed zone
Ground cross-sections revealed the extent of the LTD process penetrating from the surface into the bulk. The grinding procedure pulled the ceramic grains out of the microcracked transformed layer, making it clearly distinguishable from the underlying unaffected region of “healthy“ zirconia ( Fig. 2 ). The difference in TZD of the as-sintered and airborne-particle abraded specimens indicated that the LTD progressed at a rate different from that if the surface had been mechanically treated with airborne-particle abrasion ( Fig. 3 ).
The TZD in the as-sintered samples exhibited a uniformly growing linear trend upwards with ageing time ( p < 0.0001, adjusted R 2 = 0.9987). The estimated increase was 1.6 μm per 10 h of accelerated ageing, reaching the mean value of 79 μm after 480 h. In airborne-particle abraded specimens, the progression of TZD began at a slower rate, but later increased, as shown on Fig. 3 . The presence of two separate linear trends was demonstrated using Davies’ test. Using the fitted two-segment linear regression model the breakpoint was estimated to be at 119.6 h (standard error 5.4 h).
In the first segment of the trend, the LTD proceeded at a rate of 0.7 μm per 10 h of accelerated ageing. In the second segment, the rate increased to 1.7 μm per 10 h of accelerated ageing, which is very similar to the rate measured for the as-sintered samples. The regression model results are summarized in Table 1 .
Estimate | Standard error | t -value | 95% confidence interval | ||
---|---|---|---|---|---|
Lower bound | Upper bound | ||||
Airborne-particle abraded groups | |||||
Slope 1 | 0.70 | 0.03 | 20.38 | 0.63 | 0.76 |
Slope 2 | 1.74 | 0.01 | 125.90 | 1.72 | 1.78 |
As-sintered groups | |||||
Slope | 1.64 | 0.01 | 212.56 | 1.62 | 1.65 |
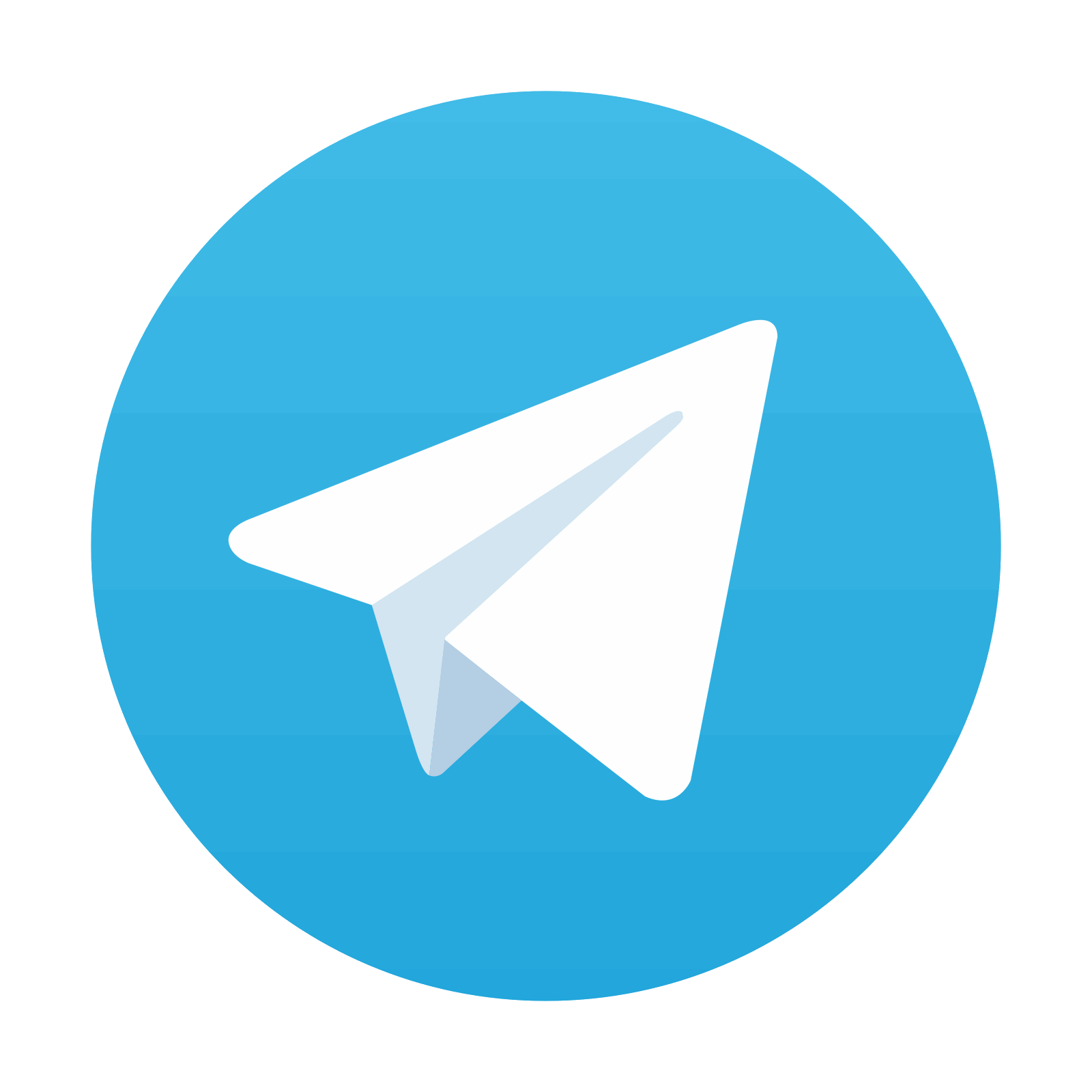
Stay updated, free dental videos. Join our Telegram channel

VIDEdental - Online dental courses
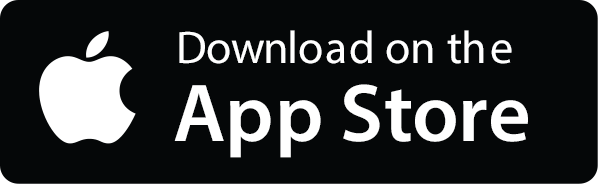
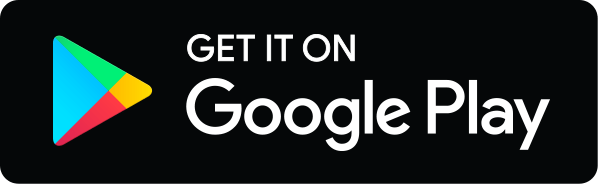
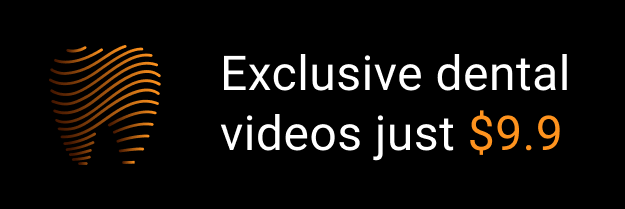