Introduction
Cytokines and chemokines regulate bone remodeling during orthodontic tooth movement. CC chemokine ligand 2 (CCL2) is involved in osteoclast recruitment and activity, and its expression is increased in periodontal tissues under mechanical loading. In this study, we investigated whether the CC chemokine receptor 2 (CCR2)-CCL2 axis influences orthodontic tooth movement.
Methods
A coil spring was placed in CCR2-deficient (CCR2 −/− ), wild-type, vehicle-treated, and P8A-treated (CCL2 analog) mice. In a histopathologic analysis, the amounts of orthodontic tooth movement and numbers of osteoclasts were determined. The expression of mediators involved in bone remodeling was evaluated by real-time polymerase chain reaction.
Results
Orthodontic tooth movement and the number of TRAP-positive cells were significantly decreased in CCR2 −/− and P8A-treated mice in relation to wild-type and vehicle-treated mice, respectively. The expressions of RANKL, RANK, and osteoblasts markers (COL-1 and OCN) were lower in CCR2 −/− than in wild-type mice. No significant difference was found in osteoprotegerin levels between the groups.
Conclusions
These data suggested a reduction of osteoclast and osteoblast activities in the absence of CCR2. The CCR2-CCL2 axis is positively associated with osteoclast recruitment, bone resorption, and orthodontic tooth movement. Therefore, blockage of the CCR2-CCL2 axis might be used in the future for modulating the extent of orthodontic tooth movement.
Orthodontic tooth movement is achieved by remodeling of the periodontal ligament and alveolar bone in response to mechanical stimulation. Bone is resorbed by osteoclasts on the pressure sites, and it is formed by osteoblasts on the tension sites. This process is regulated by an aseptic and transient inflammatory response that is characterized by the releasing of several mediators, such as cytokines and chemokines.
Chemokines, a large family of chemotactic cytokines, provide key signals for trafficking, differentiation, and activity of bone cells. The CC chemokine ligand 2 (CCL2, formerly known as monocyte chemotatic protein-1) has been found to promote chemotaxis, differentiation, and activation of osteoclasts. The cellular effects of CCL2 are mediated by its engagement with the CC chemokine receptor 2 (CCR2), which is expressed by osteoclast precursors. In addition, CCL2 expression is greatly increased in periodontal tissues with orthodontic loading, as well as in other inflammatory conditions such as rheumatoid arthritis, bone cancer metastasis, periodontal disease, and periapical osteolysis.
Studies in vitro and in vivo demonstrated that the blockage or absence of CCR2 significantly prevents bone resorption in experimental arthritis, osteoporosis, and bone fracture healing. Although the expression of CCL2 has been shown in the periodontium with orthodontic force, the functional roles of CCL2 and CCR2 in orthodontic tooth movement are not known. In this study, we aimed to investigate the roles of CCR2 and CCL2 axis in osteoclast recruitment and activity using a well-established mouse model of orthodontic tooth movement. We hypothesized that the CCR2-CCL2 axis would contribute significantly to osteoclast recruitment and, consequently, to orthodontic tooth movement.
Material and methods
Twenty-five wild-type mice (C57BL6/J) (10 weeks old), 25 CCR2-deficient mice (CCR2 −/− ) obtained from the Jackson Laboratory (Bar Harbor, Me), 5 vehicle- treated (PBS) mice, and 15 P8A-treated (a monomeric variant of the chemokine CCL2 able to inhibit CCR2-mediated leukocyte recruitment) mice were used in this experiment. CCR2 knockout mice have been previously bred into the C57BL/6 background for 9 generations. In the genome of CCR2 knockout mice, the entire coding region except the first 39 nucleotides and 5′ untranslated region of the CCR2 gene in chromosome 9 were recombined with the neomycin-resistant gene (the coding region and 3′ untranslated region are replaced with a polII-neo cassette). No expression of CCR2 has been observed in this mouse. Absence of transcript was confirmed by real-time polymerase chain reaction (PCR) by using mRNA isolated from spleens and thioglycolate-elicited peritoneal exudate cells of homozygous mutant animals. Overall, mice that are homozygous for the targeted mutation are viable, fertile, and normal in size, without any gross physical or behavioral abnormalities.
All animals were treated under the ethical regulations for animal experiments, defined by the institutional ethics committee of Universidade Federal of Minas Gerais. Each animal’s weight was recorded throughout the experimental period, and there was no significant loss of weight.
Tooth movement was induced as previously described. Briefly, the mice were anesthetized intraperitoneally with 0.2 mL of a solution containing xylazine (0.02 mg/mL) and ketamine (50 mg/mL). An orthodontic appliance consisting of a nickel-titanium 0.25 × 0.76-mm coil spring (Lancer Orthodontics, San Marcos, Calif) was bonded by light-cured resin (Transbond; 3M Unitek, Monrovia, Calif) between the maxillary right first molar and the incisors ( Fig 1 ). The magnitude of force was calibrated by a tension gauge (Shimpo Instruments, Itasca, Ill) to exert a force of 35 g in the mesial direction. There was no reactivation during the experimental period. This study was divided in 2 parts. In the first, named general, 2 groups were compared: wild-type and CCR2 −/− mice. In the second part, named specific, 4 groups were evaluated: vehicle-treated mice and 3 P8A-injected groups each given a different dose (subcutaneous administration of 0.5, 1.5, or 3.0 mg/kg/day). For the histomorphometric analysis, the left side without the maxillary appliance was used as the control. Two subgroups were used for molecular analysis: control (mice without appliances) and experimental (mice with activated coil springs). For the histopathologic analysis, the wild-type and CCR2 −/− mice were killed with an overdose of anesthetic after 6 and 12 days of mechanical loading ( Appendix Table I ). For the molecular examination, these groups were killed at 0, 12, and 72 hours ( Appendix Table II ). The vehicle- and P8A-treated groups (at the 3 different doses) were killed after 12 days of orthodontic force for histomorphometric analysis ( Appendix Table I ). For every set of experiments, 5 animals were used at each time.
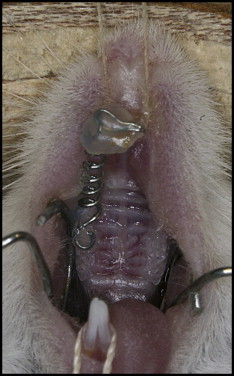
In the histopathologic analysis, the right and left maxillae halves, including the first, second, and third molars, were dissected and fixed in 10% buffered formalin (pH 7.4). After fixation, each hemi-maxilla was decalcified in 14% ethylenediaminetetraacetic acid (pH 7.4) for 20 days and embedded in paraffin. Samples were cut into sagittal sections of 5-μm thickness. The sections were stained with tartrate resistant acid phosphatase (TRAP; Sigma-Aldrich, St Louis, Mo), counterstained with hematoxylin, and used for histologic examination. The first molar’s distobuccal root, on its coronal two-thirds of the mesial periodontal site, was used for the osteoclast counts, on 5 sections per animal. Osteoclasts were identified as TRAP-positive, multinucleated cells sited on the bone surface. The slides were counted by 2 examiners (S.T. and C.Q.), and the intraclass correlation coefficient showed average measures of 0.977, validating the measurement.
Image J software (National Institutes of Health, Bethesda, Md) was used to quantify the amount of tooth movement, as previously described. Tooth movement was obtained as the difference between the distance of the cementoenamel junctions of the first molar and the second molar of the experimental side (right hemi-maxilla) in relation to the control side (left hemi-maxilla) of the same animal. Five vertical sections per animal were evaluated under a microscope (Axioskop 40; Carl Zeiss, Göttingen, Germany) adapted to a digital camera (PowerShot A620; Canon, Tokyo, Japan). Three measurements were made for each evaluation; the variability was below 5%.
With a stereomicroscope, the periodontal ligament and the surrounding alveolar bone samples were extracted from the maxillary first molars. Gingival tissue, oral mucosa, and tooth were discarded. These tissues were subjected to RNA extraction and real-time PCR to evaluate the expression of molecules known to regulate osteoclast function (receptor activator of nuclear factor kappa-B [RANK], receptor activator of nuclear factor kappa-B ligand [RANKL], and osteoprotegerin [OPG]) and osteoblast markers (osteocalcin [OCN] and collagen-1 [COL-1]). RNA was extracted by using TRIZOL reagent (Invitrogen, Carlsbad, Calif). Complementary DNA was synthesized by using 2 μg of RNA through a reverse transcription reaction (Superscript II, Invitrogen). Real-time PCR analysis was performed in MiniOpticon (BioRad, Hercules, Calif) by using the SYBR-green fluorescence quantification system (Applied Biosystems, Foster City, Calif). Standard PCR conditions were 95°C (10 minutes) and then 40 cycles of 94°C (1 minute), 58°C (1 minute), and 72°C (2 minutes), followed by the standard denaturation curve. The primer sequences are described in Appendix Table III .
The mean cycle threshold (Ct) values from duplicate measurements were used to calculate expression of the target gene, with normalization to an internal control (β-actin) by using the 2 −ΔΔCt formula.
Statistical analysis
The results in each group were expressed as the mean ± SEM. Because the data sets had normal distributions, differences among the groups were analyzed by 1-way analysis of variance (ANOVA) followed by the Newman-Keuls multiple comparison test. P <0.05 was considered statistically significant.
Results
The histomorphometric results showed that the amount of tooth movement ( Fig 2 , A , and Table ) and the numbers of TRAP-positives osteoclasts ( Fig 2 , B ) were increased after 6 and 12 days of mechanical loading in the wild-type mice ( P <0.05). On the other hand, diminished tooth movement ( Fig 2 , A , and Table ) and fewer TRAP-positive cells ( Fig 2 , B ) were observed in the CCR2 −/− mice after 12 days ( P <0.05). There was no significant difference between the groups after 6 days of mechanical loading. Moreover, microscopic analysis showed that, in the control side, TRAP activity was on the distal side of the alveolar bone surface, and no activity was observed in the mesial region of the periodontium ( Fig 2 , C ). After 6 days of orthodontic loading, there appeared to be an increase in TRAP activity on the mesial periodontium of the distobuccal root (the pressure side) and a reduction on the distal side of this root (the tension side). On day 12, TRAP activity appeared to increase more extensively in the wild-type mice ( Fig 2 , D and F ), which had greater alveolar bone resorption areas than did the CCR2 −/− mice ( Fig 2 , E and G ). In contrast, a wide hyalinized area on the mesial side was observed in the CCR2 −/− mice ( Fig 2 , F and G ).
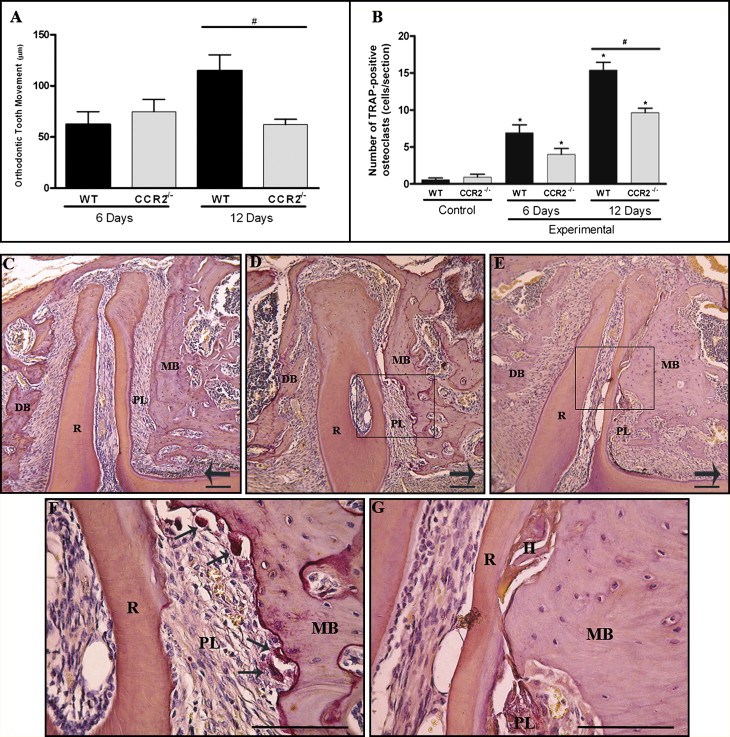
Wild type (mean ± SEM) |
CCR2 −/− (mean ± SEM) |
P value | |
---|---|---|---|
6 days | 62.5 ± 12.5 | 75 ± 11.9 | >0.05 |
12 days | 115 ± 15.5 | 62.5 ± 4.7 | <0.05 |
To investigate the importance of the CCR2 in this model, we further analyzed whether P8A, a CCL2 monomeric variant that is able to inhibit CCR2-mediated leukocyte recruitment, would also change the amount of tooth movement and osteoclast recruitment. Tooth movement ( Fig 3 , A ) and the numbers of TRAP-positive osteoclasts ( Fig 3 , B ) were reduced in the P8A-treated mice in a dose-dependent way ( Fig 3 ) when compared with the mice treated with the vehicle ( P <0.05).
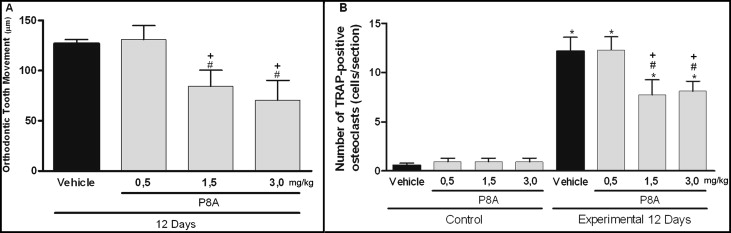
To understand the mechanisms involved in the altered bone remodeling of CCR2 −/− mice during orthodontic tooth movement, we also evaluated the expression of osteoclast regulators (RANK, RANKL, and OPG) and osteoblast markers (OCN and COL-1). RANK and RANKL mRNA levels were significantly increased after mechanical loading ( P <0.05) but were smaller in the CCR2 −/− mice than in the wild-type mice ( P <0.05) ( Fig 4 , A and B ). The mechanical stress up-regulated the OPG levels, but the increases were similar in the wild-type and the CCR2 −/− mice ( Fig 4 , C ). Expressions of OCN and COL-1 were significantly increased after mechanical loading ( P <0.05), but they were lower in the CCR2 −/− mice than in the wild-type mice ( P <0.05) ( Fig 5 ).
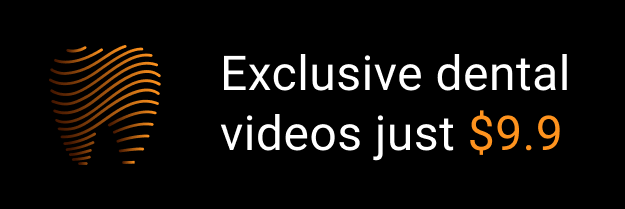