Abstract
Objective
To study the effect of compositional variables and temperature (25 °C and 37 °C) on the rheological properties of contemporary resin composites.
Methods
Fourteen commercial resin composites with different resin matrices of Bis-GMA and TEGDMA, different filler loading (41–65, v/v) and particle sizes (ranging 5 nm to 20 μm) were studied using a parallel plate rheometer. Shear sweep measurements were made to determine each composite’s viscosity over the angular frequency range of ω = 10 −4 –10 2 rad/s. Data were analyzed using one way ANOVA, Bonferroni post hoc and t -independent tests ( p < 0.05).
Results
All composites exhibited viscosity reducing with shear rate (pseudoplasticity). Viscosity averages were calculated over the shear rate range. These viscosities increased as the percentage of filler loading (by volume) increased ranging between 0.05–349.33 kPa s at 25 °C and 0.03–132.00 kPa s at 37 °C. Filtek Supreme XTE exhibited the highest viscosity at both temperatures ( p < 0.05). Filtek Bulk Fill on the other hand, showed the lowest viscosity at both temperatures ( p < 0.05). Viscosity significantly decreased with the increase in the temperatures for all materials ( p < 0.05). The reduction ranged from 40.8% (for Venus Diamond Flow) to 92.2% (for Spectrum TPH3).
Significances
There was a considerable variation in the resin composite viscosities measured at 25 °C as an average value for shear-rate sweep. The factor between the least and highest viscosities was about 7000.
The formulation differences that led to such a great variation were primarily: (i) volume fraction of fillers and (ii) particle size, the latter influencing total particle surface area.
Rheological properties such as viscosity are key parameters influencing perceived differences in handling behavior of resin composites.
1
Introduction
Resin composites are used every day in almost every dental clinic to create permanent tooth-colored restorations. Favorable handling properties of such materials should include ease of placement, shaping within the restored site, adherence to tooth surface but not to an instrument, and maintaining shape or being resistance to slumping. Prior to light curing these characteristics are indispensable in restorative procedures. One of the main characteristics is the consistency, as it affects the application and manipulation of the material . Composites are available with a wide range of consistencies and/or viscosities. The more fluid ones are termed flowable composites. Generally, composites are categorized as visco-elastic materials positioned intermediately between elastic materials, such as ceramics, and viscous materials, such as oils . The effect of any external forces on any direct dental restorative material is governed by the absolute values of their elasticity and viscosity, and their viscosity to elasticity ratios . Rheological properties of unset materials govern the way materials perform which in turn affects the handling properties of the material. A material that adheres to tooth structure, performs well within the hands of dental practitioners, and is able to reconstruct the lost original anatomy of the tooth plays a vital role in the final success of the restoration, by which the function and esthetics of the tooth is restored.
Resin composites are highly filled with inorganic particles in an organic matrix. They have both liquid-like and solid-like behaviors. Such mixtures usually show complex rheological properties such as shear-thinning (i.e. the viscosity decreases as the shear rate increases), thixotropy (i.e. the viscosity depends on the time) or yield stress (i.e. the material does not flow if the applied stress is insufficient) .
The viscosity of resin composites has an impact on the handling properties of the material. It affects the ease of manipulation, the ability of the composite and associated adhesives to adhere to the tooth structure , the operation time and the quality of the restoration . Flowable composite are usually formed by retaining the same small particle sizes of the conventional hybrid composites, but at reduced filler contents, which allow reduced viscosity of the mixture . They can be applied into a cavity using a loading tip and syringe and can be used in situations of difficult access or that require good penetration. Nevertheless, packable or condensable posterior resin composites are now available. Their viscosity is increased by increasing the filler content or by modifying the filler particle morphology and even by incorporating short glass fibers . Such packable resin composites can be placed into a cavity and packed using amalgam instruments, and good interproximal contacts in a class II cavity can be achieved.
Classification of composites has often been based on filler particle size: macrofill, minifill, microfill, and hybrid composites . Nowadays, they can also be classified as flowable, medium viscosity and packable based on viscosity or fluidity .
Several methodologies can be applied to measure the visco-elasticity of dental materials, such as: creep, stress relaxation, steady shear and dynamic measurements. In particular, dynamic oscillatory shear tests are commonly used to study the linear viscoelastic properties of a polymer . Ferracane et al. used a rotational cone and plate viscometer to measure the viscosity of chemically cured resin composites. Opdam et al. used a press method to compare the consistencies of different resin composites. Lee et al. used a dynamic oscillatory rheometer and a vertical oscillation rheometer to evaluate the viscoelastic properties of resin composites. Mesquita et al. studied the viscoelastic properties of resin composites after polymerization by dynamic mechanical analysis (DMA) .
The aim of the present study was to evaluate the influence of resin mixture, filler content and temperature on the viscosity of recent resin composites, using a dynamic oscillatory shear test. The null hypotheses were that there are no significant effects of resin mixture, filler content and temperature on the viscosity of resin composites.
2
Materials and methods
Fourteen resin composites were studied. There filler amount incorporated ranged between 41% and 65% by vol. The brand names and manufacturers are listed in ( Table 1 ) together with further information about the filler sizes and matrix monomers.
Code | Material | Manufacturer | Lot. no. | Composite description | Resin matrix | Filler content | |
---|---|---|---|---|---|---|---|
Size | Load (vol%) | ||||||
VDF | Venus Diamond Flow | Heraeus Kulzer GmbH, Hanau, Germany | 010105 | Flowable nano-hybrid | Multifunctional methacrylates (UDMA, EBADMA) | Ba-Al-F silicate glass, YbF 3 and SiO 2 (0.02–5 μm) | 41 |
FBF | Filtek Bulk Fill | 3M ESPE, ESPE, St. Paul, MN, USA | N390563 | Bulk-Fill | Bis-GMA, bis-EMA, UDMA | A combination of ytterbium trifluoride filler (sizes range from 0.1 to 5.0 μm) and zirconia/silica (size range of 0.01 to 3.5 μm) | 42.5 |
GAUF | G-aenial Universal Flo | GC Corporation Tokyo, Japan | 1204112 | Flowable nano-hybrid | UDMA bis-MePP TEGDMA | Silicon dioxide (16 nm), strontium glass (200 nm) | 50 |
TEC | Tetric EvoCeram | Ivoclar Vivadent AG, Schaan, Liechtenstein | P35262/P18603 | Nano-hybrid composite | Bis-GMA, UDMA, DMDMA | Barium glass, ytterbium trifluoride, mixed oxide and prepolymer. Mean particle size 550 nm | 54 |
SP TPH3 | Spectrum TPH3 | Dentsply Detrey GmbH, Konstanz, Germany | 1107001855 | Sub-micron hybrid composite | Bis-GMA, bis-EMA, TEGDMA | Barium aluminio borosilicate (<1 μm). Barium fluoro aluminio borosilicate (<1 μm) Highly dispersed silicon dioxide (10–20 nm) | 57 |
eXP | ever XPosterior | GC Corporation Tokyo, Japan | 1212261 | Reinforced base | Bis-GMA, TEGDMA, PMMA | Hybrid filler fractions & E-glass fibers | 57 |
IPS ED | IPS Empress Direct | Ivoclar Vivadent AG, Schaan, Liechtenstein | P43019 | Nano-hybrid composite | Dimethacrylates (20–21.5 wt%) | 40–3000 nm with a mean particle size of 550 nm | 58 |
VP | Venus Pearl | Heraeus Kulzer GmbH, Hanau, Germany | VP101210 | Nano composite | TCD-DI-HEA and UDMA | Barium aluminum fluoride glass, pre-polymerized filler, highly discrete nanoparticles (5 nm to 5 μm) | 59 |
EXHD | Esthet. XHD | Dentsply Detrey GmbH, Konstanz, Germany | 1105311 | Nano-hybrid composite | Bis-GMA, a bis- EMA, and TEGDMA | Barium fluoroborosilicate glass (<1 μm) and nano filler silica (0.04 μm) | 60 |
GAA | G-aenial Anterior | GC Corporation Tokyo, Japan | 1204232 | Micro-filled hybrid composite | UDMA and dimethacrylate. bis-GMA free | Pre-polymerized fillers (16–17 μ). Silica, strontium and lanthanide fluoride. Silica and fluoroaluminosilicate >100 nm, fumed silica <100 nm | 63 |
FS XTE | Filtek Supreme XTE | 3M ESPE, ESPE, St. Paul, MN, USA | N284875 | Nano-hybrid composite | Bis-GMA, UDMA, TEGDMA, PEGDMA and bis-EMA | 20 nm silica filler, 4–11 nm zirconia filler, and aggregated zirconia/silica cluster filler (comprised of 20 nm silica and 4–11 nm zirconia particles) | 63.3 |
VD | Venus Diamond | Heraeus Kulzer GmbH, Hanau, Germany | 010046 | Nano composite | TCD-DI-HEA and UDMA | Barium aluminum fluoride glass – highly discrete nanoparticles (5 nm to 20 μm) | 64 |
GDP | Gradia Direct Posterior | GC Corporation Tokyo, Japan | 1203121 | Micro-filled hybrid composite | UDMA and dimethacrylate co-monomers | Methacrylates of average particle size: 0.85 μm, fluoro-alumino-silicate glass average particle size: 0.85 μm | 65 |
GAP | G-aenial Posterior | GC Corporation Tokyo, Japan | 1204251 | Micro-filled hybrid composite | UDMA and dimethacrylate. bis-GMA free | Pre-polymerized fillers (16–17 μ). Silica, strontium and lanthanide fluoride. Silica and fluoroaluminosilicate >100 nm, fumed silica <100 nm | 65 |
A rheometer (Bohlin Instruments Ltd., UK, Fig. 1 ) was used to measure the dynamic viscoelastic behavior of the resin composite. A shear sweep was used to measure the viscosities. The geometry used in this test was a parallel plate design. Composite paste was placed on the lower plate of the rheometer, after which the upper aluminum plate with a diameter of 20 mm was moved downward to adjust the gap to 250 μm. This was the smallest gap that could be used with all of the materials. Any excess composite and air bubbles present around the circumference of the plate were removed, prior to initiating active measurements.

After the residual normal stress was relieved, a frequency sweep was completed over the angular frequency range of ω = 10 −4 –10 2 rad/s . The resulted shear-rate range experienced by each material was dependent upon its viscosity. The specimens were placed under increasing torque frequencies (synonymous with increasing shear rates) while the resulting shear stress developed in the resin was measured. The shear stress to shear strain values obtained enabled the calculation of the materials instantaneous viscosity (Eq. (1) ).
where ή is the viscosity, τ is the shear stress, and γ is the shear strain rate (i.e. velocity gradient).
Since the viscosity varied with shear-rate, viscosities over the measured shear-rate range were averaged and tabulated for each material and temperature. Three measurement runs were made for each material both at 25 °C and at 37 °C.
Data were analyzed using statistical software (SPSS ver.20, IBM Corp, Armonk, NY, USA) at ( p < 0.05) significance level. One-way ANOVA and Bonferroni post hoc test were used to compare the viscosity between resin composites at each temperature (25 °C and 37 °C). Also, t -test for independent data was used to compare the viscosity at both temperatures (25 °C and 37 °C) within each resin composite.
Reduction in viscosity was calculated according to (Eq. (2) )
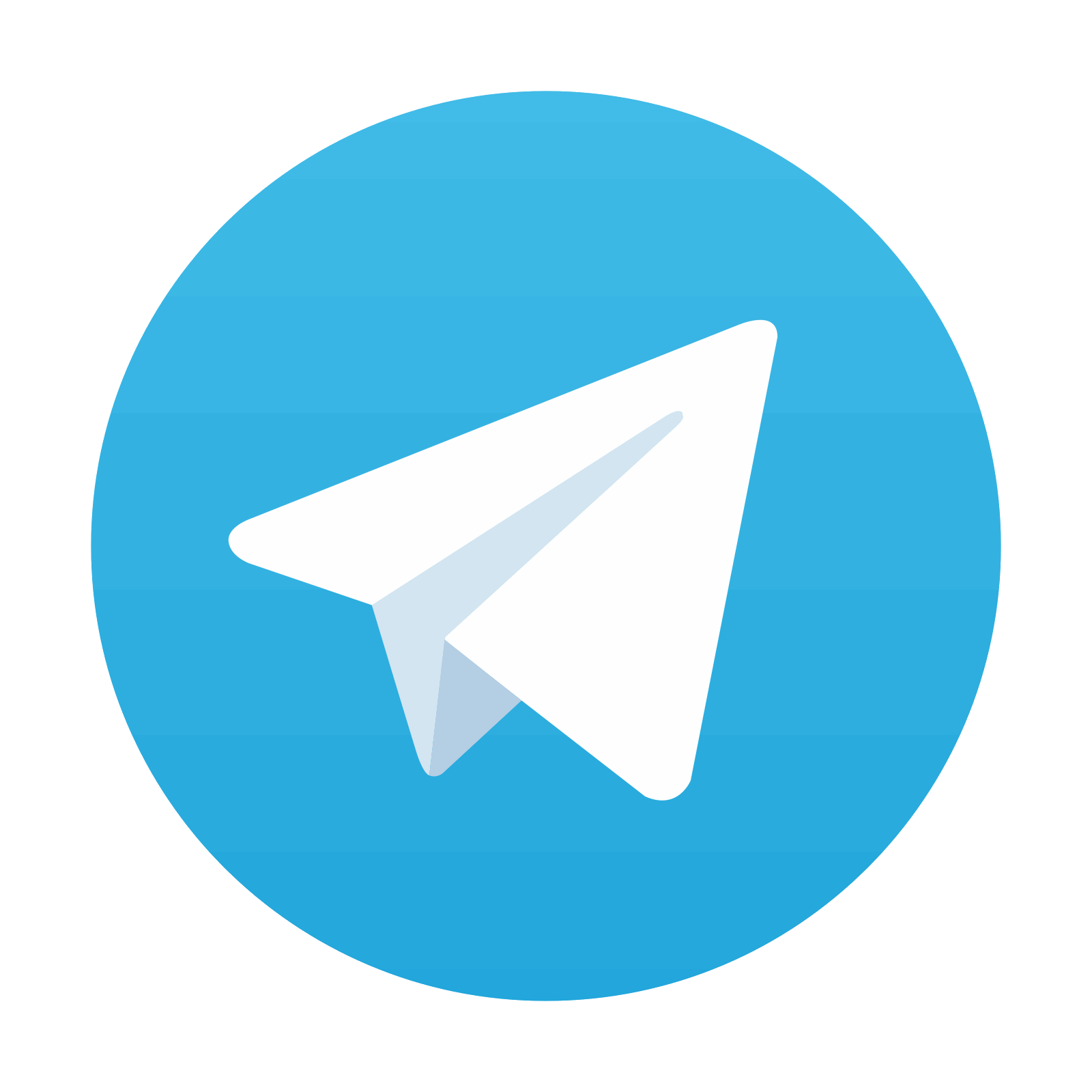
Stay updated, free dental videos. Join our Telegram channel

VIDEdental - Online dental courses
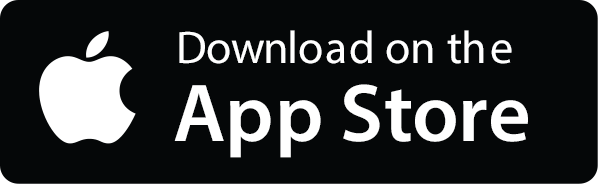
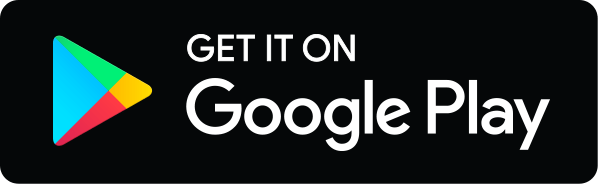