Abstract
Objective
To test the null hypothesis that the replacement of a small fraction of glass particles with random short glass fibers does not affect degree of conversion (DC), flexural strength (FS), fracture toughness (FT) and post-gel polymerization shrinkage (PS) of experimental composites.
Methods
Four experimental photocurable composites containing 1 BisGMA:1 TEGDMA (by weight) and 60 vol% of fillers were prepared. The reinforcing phase was constituted by barium glass particles (2 μm) and 0%, 2.5%, 5.0% or 7.5% of silanated glass fibers (1.4 mm in length, 7–13 μm in diameter). DC ( n = 4) was obtained using near-FTIR. FS ( n = 10) was calculated via biaxial flexural test and FT ( n = 10) used the “single edge notched beam” method. PS at 5 min ( n = 8) was determined using the strain gage method. Data were analyzed by ANOVA/Tukey test (DC, FS, PS) or Kruskal–Wallis/Dunn’s test (FT, alpha: 5% for both tests).
Results
DC was similar among groups ( p > 0.05). Only the composite containing 5.0% of fibers presented lower FS than the control ( p < 0.001). FT increased significantly between the control (1.3 ± 0.17 MPa m 0.5 ) and the composites containing either 5.0% (2.7 ± 0.6 MPa m 0.5 ) or 7.5% of fibers (2.8 ± 0.6 MPa m 0.5 , p < 0.001). PS in relation to control was significantly reduced at 2.5% fibers (from 0.81 ± 0.13% to 0.57 ± 0.13%) and further reduced between 5.0% and 7.5% (from 0.42 ± 0.12% to 0.23 ± 0.07%, p < 0.001).
Significance
The replacement of a small fraction of filler particles with glass fibers significantly increased fracture toughness and reduced post-gel shrinkage of experimental composites.
1
Introduction
In spite of the significant improvements in mechanical properties and wear over the last decades, bulk fracture remains one of the most prevalent causes of failure of composite restorations . Thus, strategies to improve composite fracture toughness (FT) should be investigated. Glass fibers have been used to reinforce resin-based materials for many decades. The first reports, dating back to the 1960s, described the reinforcement of poly(methyl methacrylate) devices, such as full dentures, with long unidirectional fibers, short multidirectional fibers or glass fiber weaves . In the 1990s, resin-impregnated unidirectional fiber bundles or weaves referred to as “fiber-reinforced composites” (FRC) became available for clinical use . These materials have several uses, such as splints or frameworks for direct, semi-direct and indirect composite fixed prostheses , as well as for fiber posts . Around the same time, silanized glass fibers with a relatively low aspect ratio ( i.e. length: diameter) of 5 (25 μm in length and 5 μm in diameter) were tested in experimental dimethacrylate-based composites . In that study, authors pointed out the difficulty of incorporating high fiber levels into the organic matrix due to incomplete wetting of the fibers (which favors air entrapment) and tendency to clustering.
Notwithstanding, light-cured restorative composites containing randomly oriented low aspect ratio glass fibers as part of their reinforcing phase became commercially available in 1990s. One of them (Restolux, Lee Pharmaceutical, South El Monte, CA, USA), no longer available, had 52 wt% of fibers (80–120 μm in lenght, aspect ratio: 5) and a total filler content of 85 wt% . The second one (ALERT, Pentron, Orange, CA, USA) has 62 vol% of filler, including glass fibers 20–50 μm in length and 6 μm in diameter . This material showed higher FT but lower flexural strength (FS) and modulus than microhybrid composites .
High aspect ratio fibers have also been tested as fillers in dental restoratives. In one study, the addition of 35–50 wt% random fibers (3 mm in length, 9 μm in diameter) to commercial particulate composites significantly increased their flexural properties . Recently, a bulk-fill composite containing 54 vol% of fillers, part of them consisting of high aspect ratio E-glass fibers (1–2 mm in length) was introduced (EverX Posterior, GC Europe, Leuven, Belgium, formerly commercialized as Xenius base, Stick Tech Ltd., Turku, Finland). Indicated as a substructure material, it has shown a significantly higher FT compared to other bulk-fill composites . Regarding its FS, however, the available reports are inconclusive when comparing the fiber-reinforced composite with other bulk-fill materials and was not significantly different than that of the composite containing low-aspect ratio fibers . A recent in vitro study did not find differences in strength of teeth restored with or without a layer of short fiber reinforced composite underneath a particulate composite .
The potential effects of fiber addition on composite post-gel polymerization shrinkage (PS) are another clinically relevant aspect. Fiber orientation has an important role on PS, as FRC with long continuous fibers were shown to shrink significantly more transversally than longitudinally to the fiber length . Short fiber reinforced composites, on the other hand, should present an isotropic behavior unless fiber orientation is somehow influenced by specimen dimensions. For example, if specimen thickness is lower than fiber length, fibers will tend to be orientated perpendicularly to specimen thickness, resulting in a quasi -anisotropic behavior. EverX Posterior displayed lower PS (determined by the strain gage method) than particulated composites with higher overall filler content . However, in a study using the “bonded-disk” method an experimental composite containing 22.5 wt% of 3 mm E-glass fibers and 55 wt% of silanized particles showed higher PS compared to a commercial composite containing 87 wt% of particles .
Studies systematically evaluating the effect of fiber content on dimethacrylate-based composite properties are scarce. Specifically regarding high aspect ratio fibers, it has been demonstrated that strength and modulus of dimethacrylate resins increased linearly with fiber content . To our knowledge, however, there are no reports on the actual influence of replacing particulated fillers with randomly oriented fibers on composite mechanical properties or shrinkage. Therefore, the aim of the present study was to test the null hypothesis that FS, FT and PS of experimental composites are not affected by the replacement of silanized glass particles with high aspect ratio glass fibers.
2
Methods
2.1
Composite preparation
S-2 glass fibers, 7–13 μm in diameter (Advanced Glassfiber Yarns, Aikin, SC, USA) were cut into 1.4-mm long segments (standard deviation: 0.5 mm, Fig. 1 ) and silanized using 3-methacryloxypropyl-trimethoxysilane (3-MPTS). The silane content on the fibers was determined by thermogravimetric analysis as being 6%. Five experimental composites were formulated, all with a total filler content of 60 vol%, divided between fibers (0%, 2.5%, 5% or 7.5%) and silanized barium glass particles (2 μm). Maximum fiber percentage was limited to 7.5 vol% because at 10 vol% the composite mass had low cohesion, and it became impossible to produce specimens without the incorporation of large flaws. The resin matrix contained equal parts by weight of bisphenol-A glycidyl dimethacrylate (BisGMA) and triethylene glycol dimethacrylate (TEGDMA), 0.5% (by weight) of camphorquinone and ethyl-4 dimethylamino benzoate (EDMAB, all components from Sigma-Aldrich, St. Louis, MO, USA).

2.2
Degree of conversion
Degree of conversion (DC, n = 4) was determined by Fourier-transformed near-infrared spectroscopy (near-FTIR). The unpolymerized material was placed in a silicone mold with 7 mm in diameter and 1 mm in thickness and pressed between two microscopy glass slides. The spectrum of the unpolymerized material was obtained between 4000 and 10,000 cm −1 by the co-addition of 32 scans with 4 cm −1 resolution (Vertex 70; Bruker Optik GmbH, Ettlingen, Germany). The composite was photoactivated through the glass slide (Radii Cal, SDI, Bayswater, Australia), receiving 24 J/cm 2 (1200 mW/cm 2 for 20 seconds) and the whole setup was dry-stored at 37 °C. After 24 h, a new spectrum was obtained. DC was calculated as the ratio between the areas of the absorption band located at 6165 cm −1 (corresponding to the absorption of the C H 2 group) of the polymerized and the unpolymerized material.
2.3
Biaxial flexural test
Disc-shaped specimens (12-mm diameter by 1.2-mm thick, n = 10) were built using a stainless steel mold. Composite was inserted into the mold, covered with a Mylar strip and photoactivated by four 10-s overlapping exposures, one per quadrant (Radii-cal, SDI). The overlapping exposures were necessary because the diameter of the light tip (12 mm) was not large enough to guarantee that the entire specimen would receive the necessary power density need for maximum DC. After 24 h in water at 37 °C, specimens were fractured on a “piston on three balls” device placed on a universal testing machine (model 5565, Instron Corp, Canton, MA, USA). The specimen was placed with the irradiated surface facing the loading piston and loaded at a crosshead speed of 0.5 mm/min. Flexural strength (FS) in MPa, was calculated according to the following equations:
where P is the fracture load (in N); b is the specimen thickness (in mm); υ Poisson’s ratio; r 1 is the radius of the supporting spheres circumference (5.0 mm); r 2 is the radius of the loading piston (0.6 mm); r 3 is the specimen radius (in mm). A Poisson’s ratio of 0.3 was adopted for all composites. Surface fractures were gold-sputtered and observed under scanning electron microscopy (LEO 430, LEO Electron Microscopy Ltda, Cambridge, UK).
2.4
Fracture toughness
FT was determined using the “single-edge notched beam” method. Beam-shaped specimens (25 mm × 10 mm × 2 mm, n = 10) with a 5 mm central notch were built using a stainless steel split mold containing a razor blade fragment at the midpoint of the mold’s length. The uncured paste was inserted in the mold and around the blade, covered with a Mylar strip and photoactivated using three overlapping exposures of 40 s (Radii Cal, SDI). The specimen was removed from the mold and stored in water at 37 °C for 24 h. Prior to testing, the edges were finished with 400-grit sandpaper and the notch was inspected under low magnification. The specimens were fractured under three-point bending (span: 20 mm) at a crosshead speed of 0.5 mm/min (Instron 5565), with the notch opening on the tensile side. After the test, the specimen notch (a), width (w) and thickness (b) were measured under a stereomicroscope (10×) using ImageJ software (National Institute of Health, Bethesda, MD, USA). Only the specimens with a / w between 0.45 and 0.50 were used. FT ( K Ic ) was calculated according to the following equations:
where P is the fracture load (in N) and S is the span (in m). The f ( a / w ) value was calculated as follows:
2.5
Post-gel shrinkage
PS was determined using the strain gage method . An adaptor (Instron Corp., model CP107495) was used to connect the strain gage to the transducer input of a universal testing machine (model 5565, Instron). The composite was pressed on a unidirectional, 3 mm × 3 mm strain gage (model PA-06-125AA-120-LEN, 120 ohms, Excel Sensores Ltda, Embu, SP, Brazil) and shaped as a hemisphere. Photoactivation was performed for 20 s, with the tip of the curing unit (Radii Cal, SDI) placed 2 mm above the surface of the composite. Microstrain data was recorded for 5 min (Blue Hill software, Instron Corp.) at an acquisition rate of four data points per second. Volumetric PS was calculated by converting the linear microstrain values to percentage linear strain (% strain = 10 −6 μ strain × 100) and multiplying by three. Eight specimens were tested per group.
2.6
Statistical analysis
Data were tested for homoscedasticity and normality using Levene and Anderson–Darling tests, respectively. DC, FS, and PS were subjected to one-way ANOVA/Tukey test. FT data were analyzed by Kruskal–Wallis/Dunn’s test. In all instances, the global significance level of 5% was used.
2
Methods
2.1
Composite preparation
S-2 glass fibers, 7–13 μm in diameter (Advanced Glassfiber Yarns, Aikin, SC, USA) were cut into 1.4-mm long segments (standard deviation: 0.5 mm, Fig. 1 ) and silanized using 3-methacryloxypropyl-trimethoxysilane (3-MPTS). The silane content on the fibers was determined by thermogravimetric analysis as being 6%. Five experimental composites were formulated, all with a total filler content of 60 vol%, divided between fibers (0%, 2.5%, 5% or 7.5%) and silanized barium glass particles (2 μm). Maximum fiber percentage was limited to 7.5 vol% because at 10 vol% the composite mass had low cohesion, and it became impossible to produce specimens without the incorporation of large flaws. The resin matrix contained equal parts by weight of bisphenol-A glycidyl dimethacrylate (BisGMA) and triethylene glycol dimethacrylate (TEGDMA), 0.5% (by weight) of camphorquinone and ethyl-4 dimethylamino benzoate (EDMAB, all components from Sigma-Aldrich, St. Louis, MO, USA).
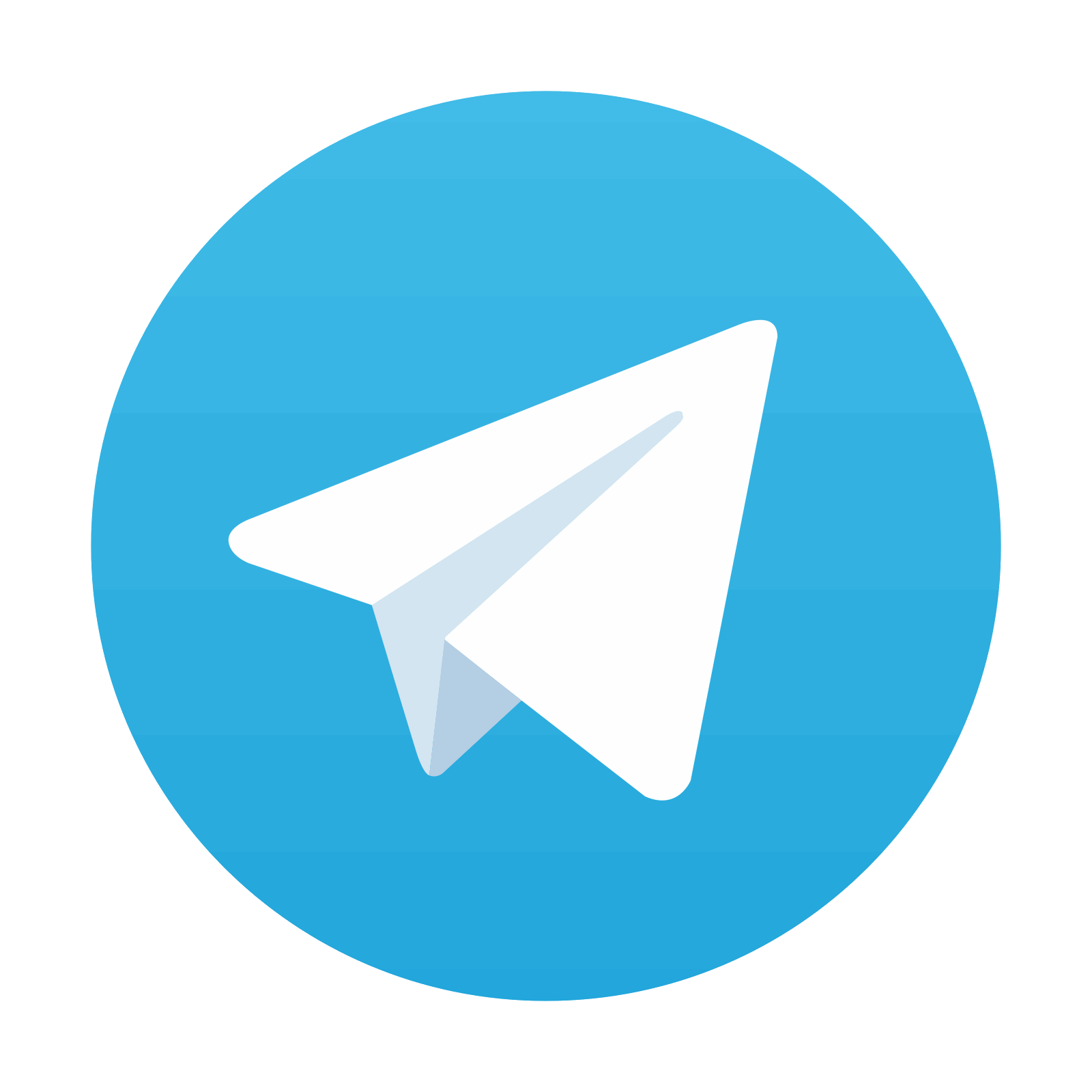
Stay updated, free dental videos. Join our Telegram channel

VIDEdental - Online dental courses
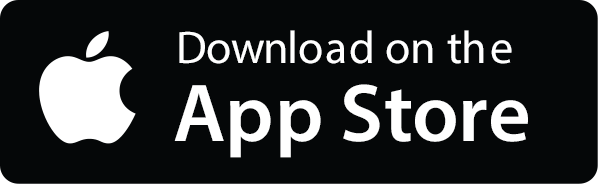
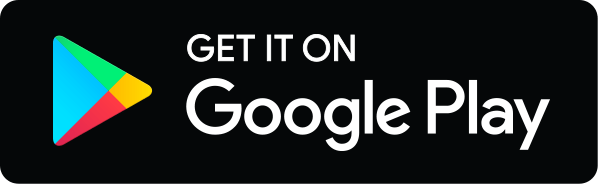
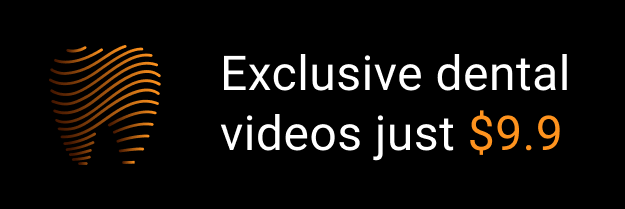