Overview of Regenerative Dentistry
Advancements in tissue engineering are dramatically changing medicine and dentistry. Tissue engineering is an interdisciplinary field that applies the principles of engineering and the life sciences to restoring, maintaining, or replacing biologic function. It involves the interplay among stem cells, growth factors, and scaffolds (biologic matrices). It has become increasingly clear that the intentional manipulation of these three factors can lead to the regeneration of tissue function that would not otherwise occur if repair had taken place without intervention. This relatively young field was first applied to medicine with many examples of regenerative medicine approaches used in the clinical practice. Although the inclusion of tissue engineering in dentistry is more recent, it is also fundamentally changing the way clinicians are treating patients while providing a fertile research field that fosters future advancements and therapies.
Most of the history of dentistry is marked by the evolution of dental materials and techniques tailored to the replacement of lost or diseased tissues with inert materials. This prosthetic replacement of missing dental tissues has prevailed in dentistry since the primordial examples of dental treatments in ancient civilizations. In contrast, the goal of regenerative dentistry is to induce biologic replacement of dental tissues and their supporting structures. The potential for regenerative dentistry is in large part due to advancements in biologic therapies that apply principles of tissue engineering with the spatial and temporal assembly of stem cells, growth factors, and scaffolds to achieve the functional regeneration of a missing tissue.
Pioneering work supporting the concept of regenerating dental tissues was reported in the 1960s when Dr. B.W. Hermann described the application of calcium hydroxide (Ca[OH] 2 ) for vital pulp therapy and Professor Nygaard-Østby evaluated a revascularization method for reestablishing a pulp-dentin complex in permanent teeth with pulpal necrosis (discussed later). The scope and clinical application of regenerative dental procedures have advanced to now include guided tissue or bone regeneration (GTR, GBR) procedures and distraction osteogenesis, the application of platelet-rich plasma for bone augmentation, Emdogain for regeneration of periodontal tissues and pulp, recombinant human bone morphogenic protein (rhBMP) for augmentation of bone, and clinical trials on the use of fibroblast growth factor 2 (FGF-2) for periodontal tissue regeneration. The potential of regenerative procedures in endodontics has been emphasized by elegant studies demonstrating the regeneration of pulp, dentin, and enamel using scaffold materials and stem cells. Thus, regenerative dental procedures are emerging as a vital, evolving field of dental care, creating a paradigm shift in many dental specialties, including endodontics. This chapter reviews the current status of regenerative endodontic procedures with an emphasis on biologic principles and the advantages and limitations of currently available clinical procedures.
Overview of Regenerative Endodontics
The developing dentition is at risk for pulpal necrosis due to trauma, caries, and developmental dental anomalies such as dens evaginatus. Loss of an immature permanent tooth in young patients with mixed dentition can be devastating, leading to loss of function, malocclusion, and inadequate maxillofacial development. These teeth were traditionally treated with apexification procedures using either long-term calcium hydroxide treatment or immediate placement of a mineral trioxide aggregate (MTA) apical plug. Although these treatments often resolve the signs and symptoms of pathosis, they provide little to no benefit for continued root development. Thus, immature teeth treated with these procedures are considered in a state of arrested development, and no further root growth, normal pulpal nociception, and immune defense should be expected.
Regenerative endodontic procedures (REPS) have been defined as biologically based procedures designed to replace damaged structures such as dentin, root structures, and cells of the pulp-dentin complex. This new treatment modality has emerged as an alternative that, in addition to healing apical periodontitis, aims to promote normal pulpal physiologic functions. These include continued root development, immune competency, and normal nociception, as seen in some published cases. Thus, the ultimate goal of these procedures is to regenerate the components and normal function of the pulp-dentin complex.
Regenerative endodontics is founded on the seminal work of Dr. Nygaard-Østby, completed in the 1960s. He hypothesized that a blood clot could be the first step in the healing of a damaged dental pulp, similar to the role of the blood clot in the healing process observed in other areas (e.g., alveolar bone following extraction). To test the hypothesis that the presence of a blood clot within a root canal system promotes healing, mature teeth diagnosed with either vital or necrotic pulp received pulp space debridement followed by foraminal enlargement, medicament dressing for the necrotic cases, evoked intracanal bleeding, and a kloroperka obturation placed coronal to the formed blood clot. Patients (n = 17) were followed for various time periods (17 days to 3.5 years), and then the treated tooth was extracted and the newly formed tissues were histologically examined. The outcomes were similar for all teeth: (1) resolution of symptoms of inflammation related to foraminal enlargement and over-instrumentation in as early as 17 days; (2) resolution of signs and symptoms of pathosis for the necrotic cases; and, in certain cases, (3) radiographic evidence of apical closure. For the histologic analysis, it was observed that there was ingrowth of connective tissue into the canal space and varied levels of mineralized tissue found along the canal walls as well as “islands” of mineralized tissue embedded within the newly formed tissue ( Fig. 10-1 ). Because dental pulp is a type of connective tissue with a rich supply of fibroblasts, this general finding was quite promising. However, the inclusion of undesired cell types (e.g., cementoblasts) and the lack of desired cell types (e.g., odontoblasts) indicate that this protocol did not lead to complete histologic regeneration of dental pulp. Despite its shortcomings, this pioneer study laid the foundation for the subsequent studies in the field of regenerative endodontics.

In 1966, a study was published reporting that disinfection could be established primarily with inter-appointment medication with polyantibiotic mixes (three different formulations used in five cases). The investigators did not purposely evoke intracanal bleeding in this study, but instrumented canals short of what they thought to be vital tissue, determined by visualization of tissue and pain sensation upon instrumentation. Signs and symptoms of disease and continued root development were resolved for all reported cases. The study represented the first reported case in which polyantibiotic pastes were used in immature necrotic teeth for disinfection and to promote root development. Five years later, another study was published that included the use of antibiotics in the disinfection protocol and the intentional promotion of intracanal bleeding. Resolution of symptoms and continued root development was a common finding. However, the histology of the extracted teeth demonstrated that connective tissue was formed in 28 out of 35 teeth, whereas cellular cementum was formed in 18 out of 35 teeth. Again, these protocols generated acceptable clinical outcomes (e.g., healing of apical periodontitis, lack of symptoms, etc.) with only partial evidence of dental pulp phenotype. Collectively, these findings laid the foundation for contemporary regenerative endodontics, demonstrating that repair could take place following root canal disinfection in immature teeth.
The first case report of a “contemporary” regenerative endodontic procedure occurred in 2001. Since then, there has been an exponential increase in published cases reporting unprecedented clinical outcomes such as resolution of signs and symptoms of apical periodontitis, continued root development, and, in certain cases, normal nociceptive responses to vitality testing. Despite the lack of randomized clinical trials, these published clinical observations support the hypothesis that patients with otherwise limited treatment options benefit from these procedures. Importantly, the field of regenerative endodontics has seen a dramatic increase in knowledge gained from translational basic science studies evaluating the interplay of the tissue engineering components (stem cells, growth factors, and scaffolds) applied to the clinical need and challenges.
Preclinical Studies on Regenerative Endodontics
Applying the principles of tissue engineering to the development of regenerative endodontic procedures requires research on the correct spatial assembly of distinct stem cells, growth factors/morphogens, and scaffolds to form a functional pulp-dentin complex. In this section, we review each of these critical components in turn.
Stem Cells
Stem cells are defined as a distinct subpopulation of undifferentiated cells with self-renewal and differentiation potential. They can be classified as pluripotent or multipotent cells. Pluripotent stem cells have the capacity of becoming specialized cells and belong to all three germ layers. Embryonic stem cells are the best example of pluripotent cells. There is a significant body of research on embryonic stem cells, but ethical, legal, and medical (tissue-rejection) issues can render these cell types unsuitable for clinical applications. True pluripotent stem cells can only be found in the developing embryo, and the harvesting of these cells requires destruction of the embryo, hence the legal and ethical concerns with such practice. Dr. Yamanka and colleagues reported the groundbreaking finding that somatic cells can be transformed into pluripotent stem cells—namely, induced pluripotent stem cells (iPSC). The use of iPSCs does not have the same legal and ethical concerns as the use of embryonic stem cells, but iPSCs share the lack of control over their uninhibited proliferation and differentiation of embryonic stem cells. These cells tend to form teratomas after implanted into a host, a true testament of their high proliferative and differentiation capacities, but this makes them unsuitable for immediate common clinical practice. On the other hand, all adult mesenchymal stem cells are more restricted in their capacity to differentiate, only forming tissues of mesenchymal origin, and therefore are classified as multipotent. These cells can be found compartmentalized within tissues in “stem cell niches.” The mesenchymal tissues (e.g., bone, dental pulp, periodontal ligament, etc.) appear to have an enriched population of adult stem cells. These cells were first found in bone marrow decades ago and were characterized as self-renewing and plastic-adherent, and they formed cell colonies with a fibroblastic appearance. They were initially called stromal stem cells but later received the now widely accepted name mesenchymal stem cells (MSCs). Most stem cells found in the orofacial region are MSCs.
Different populations of adult stem cells have been identified in tissue compartments in the oral region. These include stem cells of the apical papilla (SCAP), inflammatory periapical progenitor cells (iPAPCs), dental follicle stem cells (DFSCs), dental pulp stem cells (DPSCs), periodontal ligament stem cells (PDLSCs), bone marrow stem cells (BMSCs), tooth germ progenitor cells (TGPCs), salivary gland stem cells (SGSCs), stem cells from human exfoliated deciduous teeth (SHED), oral epithelial stem cells (OESCs), gingival-derived mesenchymal stem cells (GMSCs), and periosteal derived stem cells (PSCs) ( Fig. 10-2 ). Although stem cells have been identified in most oral tissues, the stem cells more likely to be involved in REPS are localized around the periapical region. These include SCAP, PDLSCs, BMSCs, iPAPCs, and DPSCs (if vital pulp is still present apically).

The apical papilla and its residing stem cells (SCAP) were first characterized in 2006. The apical papilla ( Fig. 10-3 ) is a dense reservoir of undifferentiated MSCs with great proliferative and odontogenic differentiation capacity. Importantly, SCAP are regulated by Hertwig’s epithelial root sheath through a series of complex epithelial-mesenchymal interactions that dictate root development and shape. Further, the close proximity of the apical papilla to the apices of teeth in continuum with the root canal space makes this rich source of stem cells readily available for regenerative endodontic therapeutics. The IPAPCs represent another important potential source of stem cells for regenerative endodontics in teeth with well-established apical periodontitis. Lastly, stem cells of the periodontal ligament (PDL) and bone marrow should be also considered as stem cells sources for regenerative procedures because the action of mechanical disruption of the apical tissue (evoked bleeding) could also trigger the release of these cells, albeit their relative abundance is thought to be significantly less than SCAP and IPAPCs. In 2011, a study was conducted to evaluate the presence of mesenchymal stem cells following the evoked-bleeding step in regenerative procedures. It was found that there is a substantial influx of mesenchymal stem cells into root canals during regenerative procedures resulting in an increase greater than 700-fold in the expression of MSC markers ( Fig. 10-4 ). In addition, the cells could be harvested from clinical samples and examined under confocal microscopy ( Fig. 10-5 ). This was the first demonstration that REPs are stem cell-based therapies. Although this study did not evaluate whether the MSCs detected in REPs are derived from the apical papilla, it was assumed that these cells were SCAP because the evoked bleeding step lacerated the apical papilla. However, these MSCs are a heterogeneous population of cells that could come from any of the periradicular tissues after the mechanical step of evoking bleeding into the root canal system.



The delivery of substantial concentrations of MSCs into the root canal space, despite advanced apical periodontitis or abscess, points to an impressive survival capacity of these cells. In these clinical presentations, low oxygen tension, low pH, and a high concentration of endotoxins and inflammatory mediators are expected. Indeed, the finding of high concentrations of the immune cell marker CD14 in those clinical samples indicates that there was still a substantial chronic inflammatory exudate present at the apical region of those teeth. These findings raise the question of how MSCs such as SCAP can survive during apical periodontitis where a complex microflora, an array of inflammatory mediators, immune cells, and presumably low oxygen tension are commonly encountered. The biologic reason for this apparent resilient survival, despite challenging conditions, may be explained by the relatively low density of blood vessels in the apical papilla in comparison to the adjacent dental pulp, whereas the dental follicle surrounding the apical papilla is highly vascularized and may act as a capillary bed to supply nutrients to SCAP. Indeed, the apical papilla was found to remain vital despite complete pulpal necrosis and advanced apical periodontitis in an animal model of endodontic infection. Further, it has been demonstrated that hypoxic environments enhance the proliferation, survival, and angiogenic potential of dental stem cells. Interestingly, similar enhancing effects were observed when dental stem cells were exposed to bacterial by-products such as endotoxin. Thus, it appears that SCAP and surrounding stem cells are equipped to survive and maintain their potential for differentiation in adverse conditions such as apical periodontitis and apical abscesses. Nonetheless, stem cells delivered into root canals after bleeding is evoked from the periradicular tissues are likely from various apical sources or niches.
The dental pulp can be viewed as a core of innervated and vascularized loose connective tissue surrounded by a layer of odontoblasts. The major cell type of this core region is the fibroblast. Together with blood vessels, lymphatics, and neurons, this core tissue is embedded in an extracellular matrix consisting of collagen and other fiber types (see also Chapter 12 ). Dental pulp stem cells (DPSCs) can be found throughout the dental pulp but are known to accumulate in the perivascular region and the cell-rich zone of Hohl adjacent to the odontoblastic layer. Thus, DPSCs from both sources are thought to be active participants in the process of reparative dentinogenesis.
Dental pulp stem cells are recruited to the site of injury following a gradient of chemotactic agents released by resident immune cells and from the damaged dentin. The reparative dentin formed by these cells is distinct from the primary, secondary, and reactionary dentin that has been lost. It is often called “osteodentin” when found to be disorganized, atubular, and having cellular inclusions. This process of cellular repair is enhanced by bioactive materials (e.g., MTA and Biodentine). These materials increase the inherent mineralizing potential of the dental pulp when used in both indirect and direct pulp applications. However, the process of tertiary dentinogenesis requires a vital pulp and the resolution of the etiology (e.g., caries or trauma). This process becomes disrupted when the pulp succumbs to injury, resulting in liquefaction necrosis of the dental pulp. Regeneration in this case is only possible with the recruitment or delivery of autologous stem cells to the canal space following adequate disinfection.
Odontoblasts are one of the most specialized cells of the pulp dentin complex with dentinogenic, immunogenic, and possibly sensorial functions. Odontoblasts in the intact pulp-dentin complex are easily identified based on their location and distinct morphologic characteristics (i.e., columnar polarized cell body with cellular projections into the dentinal tubules). However, it is far more challenging to identify and characterize an odontoblast-like cell, mainly because these cells lack a primary odontoblast morphology and unique markers that could be used for identification. Indeed, many markers used for the identification of odontoblast-like cells are also expressed in other mineralizing cell types such as osteoblasts. For example, both odontoblasts-like cells and osteoblasts are similar in the formation of mineralized nodules and in the expression of several proteins such as dentin sialoprotein (DSP), although DSP levels are nearly 400 times greater in odontoblasts than in osteoblasts. Measuring only one or two characteristics of a cell might not conclusively identify whether the cell is a true odontoblast. Even among odontoblasts, the phenotype varies in cells located in the apical (squamous shape) versus coronal (tall columnar) pulpal tissue. Importantly, molecular studies have identified many of the genes selectively expressed in odontoblasts. Lastly, an intermediate filament protein called nestin has been shown to be preferentially expressed in odontoblasts or odontoblast-like cells when in the active secretory function. Nestin expression could be used in conjunction with other markers to better identify odontoblast-like cells. This knowledge is expected to aid future studies characterizing the conditions necessary for mesenchymal cells of multiple origins to differentiate into odontoblast-like cells. It is likely that definitive cellular identification depends on both the morphology of the cell and an assessment of the expression of multiple genes.
At least five different types of postnatal mesenchymal stem cells, in addition to DPSCs, have been reported to differentiate into odontoblast-like cells, including SHED, SCAP, IPAPCs, DFPC, and BMMSC. One study demonstrated that over-instrumentation into the periradicular tissues followed by bleeding into the canal space results in a robust influx of cells with mesenchymal stem cell markers in fully mature teeth, similar to that seen in immature teeth. Thus, it appears that MSCs from the apical region can be delivered into the root canal spaces in both immature and mature teeth. However, there is growing evidence that MSCs have decreased proliferative and differentiation potential with aging. Further research is required to elucidate the age limit for the use of autologous dental-derived stem cells, but these findings suggest that regenerative procedures may be applicable to mature fully formed teeth in adults. In fact, in a proof-of-concept case report, resolution of apical periodontitis followed by narrowing of the canal space and apical closure was seen in two fully mature teeth in adult patients treated with regenerative endodontic procedures. Thus, more research and development is required to make regenerative procedures more predictable for immature teeth, and these procedures may transition to be applicable to fully formed teeth.
Growth Factors/Morphogens
Dentine is composed of collagen fibers (90%, collagen type I) and noncollagenous matrix molecules (proteoglycans, phosphoproteins, and phospholipids). The collagen fibers act as a grid or matrix, and this structure behaves as a scaffold upon which mineralization can occur. Dentine phosphoprotein (DPP) and dentine sialoprotein (DSP) are the most abundant dentine-specific proteins among the noncollagenous proteins of organic matrix. DSP resembles other sialoproteins such as bone sialoprotein, but its precise function is still unclear; it may have a role in matrix mineralization. Both DSP and DPP make up part of the small integrin-binding, ligand N-linked glycoproteins (SIBLINGS), which include dentine matrix acidic phosphoprotein 1 (DMP-1), bone sialoprotein, osteopontin, osteocalcin, and osteonectin. These proteins are only a small part of the whole cocktail of noncollagenous proteins that form components of the dentine.
Research on dentine structure and composition has highlighted that the matrix contains some components that may be important in regulating tissue due to their bioactive properties. For this reason, dentine is today considered a reservoir of growth factors and cytokines. These growth factors/cytokines are secreted by the odontoblasts during primary dentinogenesis, becoming sequestered and “fossilized” into the dentine after biomineralization ( Table 10-1 ). However, they may become solubilized by demineralization of the matrix, bacterial acid (caries decay), chemical treatment (EDTA rinsing solution, calcium hydroxide or acid etching for bonded restorations), or restorative materials such as mineral trioxide aggregate and Biodentine.
Growth Factors in Dentin Matrix | |
---|---|
Transforming growth factor beta-1 (TGFβ-1) | Cassidy et al., 1997 |
Transforming growth factor beta-2 (TGFβ-2) | Cassidy et al., 1997 |
Transforming growth factor beta-2 (TGFβ-3) | Cassidy et al., 1997 |
Bone morphogenic protein –2 (BMP-2) | Thomadakis et al., 1999 |
Bone morphogenic protein –4 (BMP-4) | About et al., 2000 |
Bone morphogenic protein –7 (BMP-7) | Thomadakis et al., 1999 |
Insulin growth factor-1 (IGF-I) | Finkleman et al., 1990 |
Insulin growth factor-2 (IGF-II) | Finkleman et al., 1990 |
Hepatocyte growth factor (HGF) | Tomson et al., 2013 |
Vascular endothelial growth factor (VEGF) | Roberts-Clark and Smith, 2000 |
Adrenomedullin (ADM) | Musson et al., 2010 |
These growth factors and their receptors have been shown to be present at the enamel organ-dental papilla interface by immunohistochemistry and in situ hybridization during tooth development and have been implicated in odontoblast differentiation:
- ♦
Growth hormone (GH) plays a paracrine or autocrine role in dental development.
- ♦
IGF-1 and -2 (of the family of IGF: insulin-like growth factor).
- ♦
TGFβ-1, -2, and -3 and BMP-2, -4, and -6 play a role in the polarization and the differentiation of odontoblasts. Notably in adult pulp, TGFβ-1 plays an important role in the regulation of the inflammatory response and tissue regenerative processes.
The dental pulp has well recognized regenerative potential observed in the process of reparative dentinogenesis. In this process, dentin-derived growth factors are thought to play a key role to be deciphered into the regulation of progenitor cell recruitment, cell proliferation, and differentiation of new dentine-secreting cells. Indeed, the differentiation of new odontoblast-like cells has also been reported following pulp capping with basic fibroblast growth factor (FGF), TGF-β1, and BMP-7.
The sequestration of these growth factors in the dentine matrix and their subsequent “fossilization” during the mineralization process appears key to the pulp healing process where their release from the matrix may be responsible for various signaling events. These growth factors are extremely potent and have a variety of cell signaling properties. However, their precise localization in the dentine and their various biologic roles remain to be elucidated.
It is possible to imagine opportunities for therapeutic stimulation, inducing a targeted release of these proteins. For example, treatment of dentin with EDTA solution has been shown to dissolve the mineral phase, liberating growth factors that orchestrate the stimulation of progenitors or stem cell differentiation. Etching with orthophosphoric acid, used for conditioning the dentine in bonding procedures, also promotes demineralization of the dentine and liberation of biologic factors. For a long time, calcium hydroxide has been used as a protective lining, especially beneath amalgams fillings, or as a canal disinfection medication. It has been shown to have the ability to release bioactive components from the dentine, including growth factors. Unlike dentin-etching acids, which only have brief contact with the dentine, calcium hydroxide remains in place beneath restorations or in canals allowing for a gentle and continued dissolution, thus releasing growth factors; its action is prolonged and potentially controllable depending on the form of the product. Lastly, calcium hydroxide, a by-product of the use of MTA and Biodentine, appears to underlie release of bioactive dentin-derived growth factors by these two bioactive materials. Thus, clinicians may take advantage of potent growth factors stored within dentin with the use of chemical treatments and materials that promote the release of these factors.
Morphogens
It is also important to clearly keep in mind that a second level of regulation exists during dental development (and thus during pulp regeneration process)—transcription factors. Notably, Msx1 is expressed in polarized preodontoblasts, whereas Msx2 is present in mature odontoblasts. Protein and transcripts for Msx1 have been identified in the pulp mesenchyme at early stages of tooth development and their concentrations decreased at the bell stage. The expression of these transcription factors is under the control of growth factors, and they can ultimately have broad-ranging effects. Significantly, BMP4 upregulates Msx1 and Msx2 expression. In turn, transcription factors regulate further growth factor expression; for example, Msx1 upregulates BMP4 synthesis in the mesenchyme, and Msx2 regulates Runx2 and osteocalcin gene expression during odontogenesis.
Growth factors and transcription factors are central to the cascade of molecular and cellular events during tooth development and are responsible for many of the temporospatial morphologic changes observed in the developing tooth germ. For these reasons, they are also likely involved in the regeneration process.
It is also important to consider the nature of the signaling process between the injurious agent and the pulp cells. Bacteria and their toxins are key candidates in the direct stimulation of pulp cells. Lipopolysaccharides (LPS) and other bacterial toxins initiate intrapulpal inflammatory processes by activation of Toll-like receptors (e.g., TLR4 activation by LPS). Importantly, both progenitor and dental stem cells have been shown to express these receptors. Thus, stem cells within the dental pulp or periradicular tissues are equipped to detect microorganisms. Exposure of these cells to microbial antigens has been shown to directly modulate the proliferation and differentiation potential of these cells. Lastly, cytokines commonly found in the inflammatory milieu (including that of the dental pulp) have a profound effect on stem cells. For example, it has been shown that TNF-alpha stimulates differentiation of dental pulp cells toward an odontoblastic phenotype via MAP kinase pathway activation and p38 phosphorylation. Therefore, stem cell fate within the dental pulp is ultimately dictated by a complex cascade of intracellular signaling pathways activated by agents released from microorganisms, dentin, and immune cells.
Interestingly, morphogens are not only naturally occurring factors found within teeth. Several growth factors have also been evaluated for their ability to trigger the differentiation of selected mesenchymal stem cell populations into odontoblast-like cells ( Table 10-2 ). Interestingly, several case studies have reported that patients taking long-term corticosteroids often present with dramatic reduction of the radiographic size of the pulp chamber and up to a fivefold increase in the thickness of the predentin layer. Although these were medically complex patients (e.g., those experiencing renal failure) taking multiple drugs, the use of corticosteroids appeared to be associated with the observed increased activity of human odontoblasts. Further, these unexpected “side-effects” were also observed in a retrospective study evaluating the association of pulp calcifications and the long-term use of statins. These incidental effects of commonly prescribed medications were further evaluated in translational studies that have extended this general observation by demonstrating that the application of dexamethasone or statins greatly increased the differentiation of human dental pulp cells into odontoblast-like cells. This was particularly evident when dexamethasone was combined with 1,25-dihydroxyvitamin D 3 . Merely changing the composition of growth factors completely altered the differentiation of these cells, with the same population of cells able to express markers of odontoblasts, chondrocytes, or adipocytes, depending on their exposure to different combinations of growth factors. Such findings emphasize the importance of growth factors in guiding the differentiation of these cells. Other studies have evaluated growth factors administered alone or in various combinations for promoting differentiation of odontoblast-like cells.
Growth Factors | Cell Source | Phenotype | Condition | Authors |
---|---|---|---|---|
Dexamethasone | Human dental pulp | Odontoblast-like | In vitro × 8 weeks | Huang et al., 2006 |
Dexamethasone and Vitamin D 3 | Human dental pulp | Odontoblast-like | In vitro × 8 weeks | Huang et al., 2006 |
Dexamethasone and Ascorbate-2-phosphate and β-Glycerophosphate | Human or rat dental pulp | Odontoblast-like | In vitro × 3 weeks | Wei et al., 2007 Zhang et al., 2005 |
Insulin and Indomethacin and 3-Isobatyl-1-methylxanthine (IMBX) | Human dental pulp | Adipocyte | In vitro × 19 days | Wei et al., 2007 |
Dexamethasone and Insulin and Ascorbate-2-phosphate and Sodium pyruvate and TGF-β1 | Human dental pulp | Chondrocyte | In vitro × 8 weeks | Wei et al., 2007 |
Growth/differentiation factor 11 (Gdf11) | Dental pulp | Odontoblast-like | In vitro/in vivo 10 days | Nakashima et al., 2004 |
Simvastatin (statins) | Human dental pulp | Odontoblast-like | In vitro/in vivo | Okamoto et al., 2009 |
LIM mineralization protein 1 (LMP-1) | Human dental pulp | Odontoblast-like | In vitro/in vivo | Wang et al., 2007 |
Bone morphogenetic proteins | Dental pulp | Odontoblast-like | In vitro | Saito et al., 2004 Sloan et al., 2000 Chen et al., 2008 |
TGF-β1-3 | Rat/monkey dental pulp | Odontoblast-like | In vitro | Sloan et al., 1999 |
Demineralized dentin | Human or rodent pulp | Odontoblast-like | In vitro/in vivo | Smith et al., 1990 Smith et al., 2001 Tziafas, 2004 |
Nerve growth factor (NGF) | Immortalized apical papilla | Odontoblast-like | In vitro | Arany et al., 2009 |
Fibroblast growth factor 2 | Human dental pulp | Odontoblast-like | In vitro | He et al., 2008 |
Dentin matrix protein 1 | Rat dental pulp | Odontoblast-like | In vivo | Almushayt et al., 2006 |
Several of the approaches using compounds later found to have growth factor–like effects have immediate clinical implications. First, it is unlikely that a single growth factor will result in maximal differentiation, so combinations of growth factors may be required for evaluation in clinical trials. Related to this point, many of the studied growth factors (e.g., dexamethasone, insulin) are drugs already approved for human use in other medical/dental applications. Second, the demonstration that statins promote the differentiation of an odontoblast-like phenotype suggests that patients clinically taking statins may also have narrowing of the pulp chamber space, similar to the findings previously described for corticosteroids. This would be an important future area of research. Third, clinicians have long used demineralized human bone to augment healing after surgical procedures. Demineralized human bone is thought to contain a natural combination of appropriate growth factors and scaffolds, thereby providing an appropriate environment for osteoblast differentiation or function. Extending this concept, several research groups have demonstrated that demineralized human dentin has significant benefit for promoting the differentiation of odontoblast-like cells. Importantly, translational studies in regenerative endodontics have demonstrated that irrigation of dentin with 17% EDTA increases the survival of stem cells and odontoblastic differentiation, possibly due to the release of bioactive molecules from dentin. Collectively these findings suggest that EDTA irrigation of the dentinal walls as part of an REP could improve clinical outcomes.
Scaffolds
An important component of tissue engineering is a physical scaffold. Tissues are organized as three-dimensional structures, and appropriate scaffolding is necessary to (1) provide a spatially correct position of cell location and (2) regulate differentiation, proliferation, or metabolism while promoting nutrient and gaseous exchanges. Extracellular matrix molecules are known to control the differentiation of stem cells, and an appropriate scaffold might selectively bind and localize cells, contain growth factors, and undergo biodegradation over time. Thus, a scaffold is far more than a simple lattice to contain cells, but instead can be viewed as the blueprint of the engineered tissue.
Scaffolds can be classified as either natural or synthetic. Examples of natural scaffolds include collagen, glycosaminoglycans, hyaluronic acid (HA), demineralized or native dentin matrix, and fibrin. On the other hand, examples of synthetic scaffolds include poly-L-lactic acid (PLLA), polyglycolic acid (PGA), polylactic-coglycolic acid (PLGA), polyepsilon caprolactone, hydroxyapatite/tricalcium phosphate, bioceramics, and hydrogels such as self-assembly peptide hydrogels. The great majority of currently published regenerative endodontic procedures involve evoked bleeding and the formation of a blood clot to serve as a scaffold. Although it is relatively straightforward as it does not require ex vivo manipulation, this simplistic approach is not without challenges. The blood clot is often difficult to achieve, and it does not have many of the properties of the ideal scaffold. These properties include easy delivery, adequate mechanical properties, controllable biodegradation, and incorporation of growth factors. In addition, the blood clot contains a great number of hematopoietic cells that eventually undergo cell death, releasing their toxic intracellular enzymes into the microenvironment, which may be detrimental to stem cell survival.
Another approach for creating a scaffold involves the use of autologous platelet-rich plasma (PRP). It requires minimal ex vivo manipulation, being fairly easy to prepare in a dental setting. PRP is rich in growth factors, degrades over time, and forms a three-dimensional fibrin matrix. Platelet rich fibrin (PRF) is an alternative to PRP, as it has a three-dimensional architecture conducive with stem cell proliferation and differentiation and contains bioactive molecules. These autologous scaffolds have been used successfully in regenerative cases. However, it should be emphasized that, despite their reported use, there are some drawbacks to their clinical use: the process requires collection of intravenous blood that can be challenging in children, the diversity and concentration of growth factors within PRP and PRF preparations are not controllable, and they lack temporal degradation control and the mechanical strength to support a coronal restoration. Thus, despite some desirable characteristics, other scaffold alternatives to PRP and PRF should be carefully considered.
Hydrogels are a class of scaffolds composed of three-dimensional hydrophilic polymers that absorb water or tissue fluids up to several times their weight. These water-swollen materials are easily injectable in their colloidal form, undergoing gelation by chemical (e.g., changes in pH and osmolarity) or physical (e.g., temperature change) cues. These materials are highly tunable, biocompatible, and can be designed to resemble naturally occurring extracellular matrices. They are of particular interest for regenerative endodontics because they can be easily injected into narrow root canal spaces and can be modified to deliver chemotactic and angiogenic agents to drive stem cell homing and supportive angiogenesis. Hydrogels made of self-assembly peptides (e.g., Puramatrix) show great potential to be used in endodontic tissue engineering because their sequence includes short peptide sequences, similar to those naturally occurring in tissues, enhancing cell attachment and proliferation.
Delivery System
Even with selection of the appropriate cell source, growth factors, and scaffold, the resultant mixture must be delivered in a spatially appropriate fashion into the space of the root canal system. For example, nearly all cells of the body are within 0.1 to 1 mm of a blood vessel in order to maintain adequate diffusion of oxygen and nutrients. This represents a challenge still to be overcome in the currently performed regenerative endodontic procedures that recruit stem cells to a canal space devoid of lateral vascularity and several millimeters away from apical blood vessels. If one were to inject cells, in a cell-based approach, along the entire coronal-apical extent of a root canal system, the majority of cells would be expected to succumb to tissue hypoxia. Interestingly, it has been demonstrated that under hypoxic conditions, stem cells proliferate faster and release greater levels of angiogenic factors such as vascular endothelial growth factor 1 (VEGF) that promote targeted angiogenesis into the engineered space. Thus, an alternative approach would be to inject a scaffold with chemotactic factors into the root canal. This approach is called cell homing, as cells are attracted to the scaffold along with supportive blood vessels in a progressive manner ; instead of being abruptly delivered to an avascular space (i.e., similar to the current revascularization procedures), the cell-homing approach can be applied in a cell-free (no cells implanted along the chemotactic factors, see Fig. 10-6 ) or cell-based approach (cells are delivered in the chemotactic-containing scaffold). Because dental pulp can be approximated as a loose connective tissue core surrounded by a layer of odontoblasts, the spatial arrangement of cells and growth factors within the scaffold may be particularly important to promote odontogenesis without having complete calcification of the root canal system. Complete recapitulation of the pulp-dentin complex architecture requires additional research effort.

Translational Studies
Several elegant studies in regenerative endodontics have used various translational methodologies including evaluation of clinical samples, organotypic root canal models, tooth slice models, whole tooth culture, and animal models. These studies have been crucial to provide a strong scientific foundation for the field of regenerative endodontics while allowing for clinical treatment protocol optimization and the development of new treatment strategies such as inclusion of scaffold and growth factors in regenerative procedures.
One study demonstrated that new dentin and pulplike tissue could be generated in human root segments implanted subcutaneously in immune-compromised mice. In this study, root segments had one of the openings sealed with MTA to mimic the coronal restoration of regenerative endodontic cases. The canal space was filled with either SCAP or DPSCs in a PLGA-based scaffold. The implants were harvested 3 months later and processed for immunohistochemical analysis. The results indicated that there was a dramatic circumferential apposition of dentin-like material along the dentinal walls. The new mineralized tissue was lined with polarized cells expressing odontoblastic markers. In addition, the dentin-like tissue was largely atubular and displayed cellular inclusions similar to the histologic presentation of osteodentin. Importantly, the cells from the engineered pulp were positive for human mitochondria, demonstrating that they originated from the implanted human stem cells and not from the host (mouse). Lastly, the root segments that were implanted without stem cells had only connective tissue that did not resemble a pulplike tissue, nor did it have mineralized tissues and odontoblast-like cells. Thus, a pulp-dentin complex could be engineered in human roots implanted subcutaneously in immune-deficient mice.
Nakashima and colleagues accomplished complete pulpal regeneration in dogs. In this elegant study, dental pulp was removed via a sterile pulpectomy procedure, followed by placement of sorted CD105+ DPSCs in a collagen gel to the mid-root. The remaining coronal part of the canal was back-filled with the collagen gel containing the chemotactic factor stromal derived factor 1 (SDF-1). Subsequent histology demonstrated the formation of new pulp tissue with innervation, vascularization, and odontoblast-like cells lining the dentinal walls. In addition, the engineered pulp had protein and RNA expression similar to the native dental pulp.
In another important study, mobilized DPSCs were generated by selecting DPSCs that migrated toward a concentration gradient of granulocyte-colony stimulating factor (G-CSF). These selected cells were implanted in a collagen gel into pulpectomized root canals. Complete pulpal regeneration was observed, with evidence of new dentin formation, blood vessels, and innervation in the engineered tissue ( Fig. 10-7 ). The impressive results of this cell-based approach have laid the foundation for the use of this technology in emerging clinical trials.

It is noteworthy that all of the demonstrations of pulp regeneration in animal models were in root canals without any history of infection and pulpal necrosis. Previously infected root canals must be adequately disinfected in order to suppress chronic inflammation that is detrimental to regeneration. However, many irrigants and medicaments have detrimental effects on stem cell survival and differentiation. Several studies using organotypic root canal models and animal models evaluated the combination and concentrations of irrigants and medicaments that allowed for stem cell proliferation and differentiation. Thus, adequate disinfection and resolution of inflammation appears to be a limiting factor in complete pulpal regeneration, despite exciting results from current advances in dental pulp tissue engineering.
Summary of Basic Research on Regenerative Endodontics
Regeneration of a functional pulp-dentin complex relies on the foundation of tissue engineering and can be viewed as a function of the spatially correct delivery of appropriate stem cells and growth factors embedded within a scaffold. Although considerable research has used in vitro cell-culture methods to identify key factors regulating the differentiation of odontoblast-like cells, emerging studies conducted in animal models are promising for regeneration of this pulp tissue. Preclinical studies involving surgical placement of a human tooth filled with a human stem cell/growth factor/scaffold combination into immunocompromised mice have permitted histologic analysis of neovascularization as well as the differentiation and mineralization activity of newly formed odontoblasts. The use of human cells in a mouse model permits histologic confirmation that the resulting odontoblast-like cells were of human origin. These novel findings provide strong evidence that either human SCAP or DPSC cell sources, on a PLGA scaffold, were able to regenerate a vascularized tissue that had histologic evidence of odontoblast-like cell differentiation and the spatially appropriate formation of dentin-like material onto the root canal walls. Although no specific growth factors were added to this mixture, it is important to note that the root canal walls were treated with 17% EDTA, an irrigant known to expose endogenous growth-factor proteins embedded in the dentinal walls. This and other related studies provide strong impetus for clinical translational research evaluating various potential regenerative endodontic therapies.
Clinical Studies on Regenerative Endodontics
To date, most case reports, case series, and retrospective studies published in regenerative endodontic have not fully incorporated the tissue-engineering concepts described. Instead, most of these reports present cases with variations of revascularization techniques. These procedures were initially performed empirically with a strong focus on disinfection and the intentional bleeding into the root canal. However, it became obvious that these were in fact stem-cell based procedures with all three components of the tissue engineering triad present: stem cells, growth factors, and scaffolds. Important preclinical studies previously described in this chapter have provided the foundational framework for a paradigm shift. This shift represents a clear departure from the traditional “disinfect the canals at all cost” to “disinfect while creating a microenvironment conducive for tissue engineering.” Varied terminologies have been given to these procedures, including revascularization, revitalization, and maturogenesis. Of these terms, the most popularly used has been revascularization. This term is largely based on the trauma literature observation that immature teeth could become revascularized after trauma. However, revascularization is a term better used for the reestablishment of the vascularity of an ischemic tissue, such as the dental pulp of an avulsed tooth. From this perspective, a focus on revascularization would ignore the potential importance of growth factors and scaffolds that are required for histologic recapitulation of the pulp-dentin complex. Although we appreciate that angiogenesis and the establishment of a functional blood supply are key requirements in the maintenance and maturation of a regenerating tissue, it is noteworthy that some of the published cases report positive responses to pulp sensitivity tests such as cold or electric pulp tests. This is evidence that a space that was previously vacant (debrided root canal) may become populated with an innervated tissue supported by vascularity. Taken together, the core concepts of tissue engineering distinguish a regenerative treatment philosophy from a revascularization philosophy derived from certain trauma cases (which only occur in a low percentage of replanted teeth). Lastly, a number of procedures have been performed with intentional manipulation of the principles of tissue engineering such as use of autologous platelet-rich plasma (PRP), platelet-rich fibrin (PRF), and exogenous growth factors and scaffolds. Thus, instead of using different terms for each variant of these procedures, we will refer to them simply as regenerative endodontic procedures (REPS), which include past, present, and future procedures that aim for the functional regeneration of the pulp dentin–complex.
Clinical Procedures Related to Regenerative Endodontics
Clinicians face several challenges when presented with an incompletely formed root in need of endodontic treatment. Because the apex is not fully developed and often has a blunderbuss shape, cleaning and shaping of the apical portion of the root canal system can be difficult. The process is further complicated by the presence of thin, fragile dentinal walls that may be prone to fracture during instrumentation or obturation. In addition, the open apex increases the risk of extruding material into the periradicular tissues. Traditionally, an immature tooth with an open apex is treated by apexification, which involves creating an apical barrier to prevent extrusion. In many cases, this entails an involved, long-term treatment with Ca(OH) 2 , resulting in the formation of a hard-tissue apical barrier. However, a disadvantage of the traditional apexification procedures is that the short-term or long-term use of Ca(OH) 2 has the potential to reduce root strength. This finding is consistent with a large case series using the traditional apexification protocol; it showed that a major reason for tooth loss following apexification was root fracture. In a retrospective study, the use of calcium hydroxide in apexification procedures resulted in the fracture of 23% of the teeth treated during the follow-up period of up to 18 months. The advent of one-step apexification, by the creation of artificial barriers using materials such as MTA, has greatly decreased the number of appointments and time to completion. Importantly, one-step apexification has been shown to have as high a success rate as apexification with calcium hydroxide in resolving apical periodontitis (both symptoms and radiographic presentation). However, apexification procedures do not generally result in further root development. A primary advantage of regenerative endodontic procedures in these cases is the greater likelihood there will be an increase in root length and root wall thickness, in addition to the possibility that the patient will regain vitality responses.
There have been numerous published cases of regenerative endodontic procedures. Investigators and clinicians have used a variety of medicaments to disinfect the canal space. Approximately 51% of the cases included the use of a triple antibiotic paste (a 1 : 1 : 1 mixture of ciprofloxacin/metronidazole/minocycline), whereas 37% used Ca(OH) 2 as an intracanal medicament.
The development of the triple antibiotic paste was led in large part by Hoshino and colleagues. They demonstrated the effectiveness of combinations of antibiotics (and in particular the high efficacy of the combination of ciprofloxacin, metronidazole, and minocycline) in eradicating bacteria from the infected dentin of root canals. Astute practitioners realized that the triple antibiotic paste could be a valuable adjunct for revascularization procedures, because it could be used to create an environment favorable for the ingrowth of vasculature and regenerative cells by reducing or eradicating bacteria in the canal space of teeth with necrotic pulps and incompletely formed apices. The efficacy of the triple antibiotic paste in disinfecting necrotic root canal systems has been demonstrated in a preclinical model. In this dog study, 60 teeth were accessed and infected by sealing dental plaque and sterile saline on a cotton pellet into the pulp chamber for 6 weeks. By the end of this period, each premolar was radiographically confirmed to have apical periodontitis. The canals were then sampled at three time points: before and after irrigation with 1.25% NaOCl, and 2 weeks after the delivery of the triple antibiotic paste into the root canal system using a Lentulo spiral. Before irrigation, all of the teeth had positive cultures for anaerobic bacteria, with a mean colony-forming unit (CFU) count of 1.7 × 10. After irrigation with 1.25% NaOCl, 10% of the teeth sampled cultured bacteria free. The mean CFU count was 1.4 × 10 4 , or an approximate 10,000-fold reduction in viable bacteria. After dressing with the triple antibiotic paste for 2 weeks, 70% of the teeth sampled cultured bacteria free. The mean CFU count was only 26, which is about another 1000-fold drop in bacteria. These findings were confirmed in another related dog study. This study provides strong support for the effectiveness of triple antibiotic paste in disinfection of immature teeth with apical periodontitis.
As stated previously, calcium hydroxide has been the second most used intracanal medicament in published cases. This application represents a new use of a long established intracanal medicament in endodontics. Although Ca(OH) 2 appears to be less effective against some intracanal bacterial species than antibiotic paste formulations, its use is associated with lower cytotoxicity to stem cells, release of important bioactive growth factors from the treated dentin, and greater survival and proliferation of stem cells in the presence of the conditioned dentin. Also, the relatively short-term use of this medicament in regenerative procedures does not appear sufficient to reduce fracture resistance. Another factor to consider when choosing an intracanal medicament is the ability to remove the medicament from the canal space. One study that addressed this question incorporated radioactive tracers in both calcium hydroxide paste (Ultracal, Ultradent, Inc.) and triple antibiotic paste (Champs Pharmacy, San Antonio, TX). The radiolabeled medicaments were placed in extracted teeth with standardized root canals. After 28 days of incubation, canal spaces were irrigated with a standardized protocol using different techniques. Surprisingly, greater than 80% of the triple antibiotic paste could not be removed from the tooth ( Fig. 10-8 ), and it was found not in the canal lumen, but greater than 350 µm into the dentinal tubules. In contrast, greater than 80% of calcium hydroxide was removed (see Fig. 10-8 ) with the remaining medicament present superficially within dentin. This is an important finding, given that drugs remaining within dentin are likely to have an effect on the fate of stem cells in contact with the treated dentin. One study revealed that triple antibiotic paste (TAP), when used at concentrations typically used in case reports (1 g/mL) and removed with standardized irrigation protocol, resulted in no SCAP survival. In contrast, dentin treated with calcium hydroxide promoted SCAP survival and proliferation, and dentin treated with TAP at a 1 mg/mL had no effect on SCAP survival ( Fig. 10-9 ). Thus, clinicians must carefully evaluate the advantages and disadvantages of each intracanal medicament while observing the ideal therapeutic concentration.

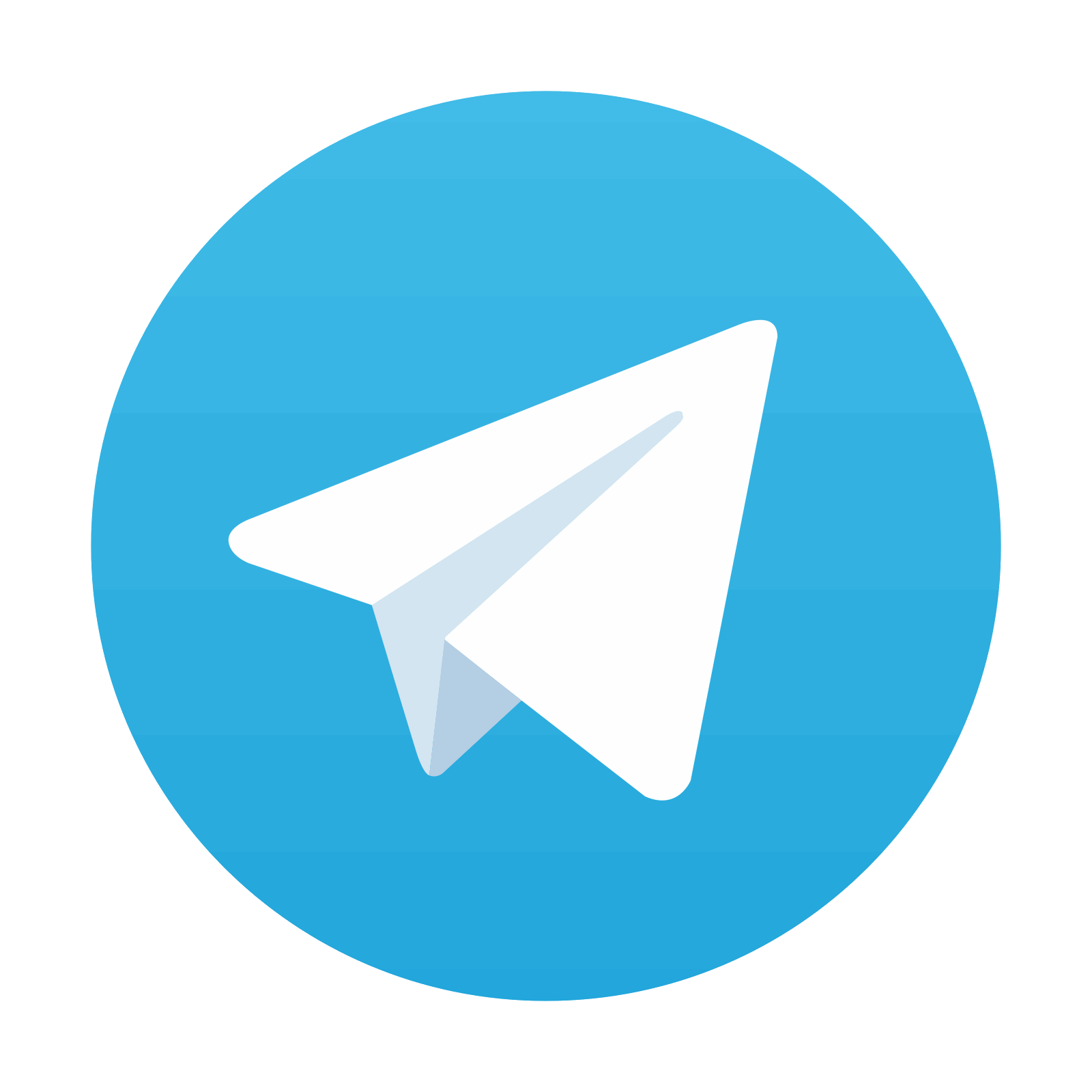
Stay updated, free dental videos. Join our Telegram channel

VIDEdental - Online dental courses
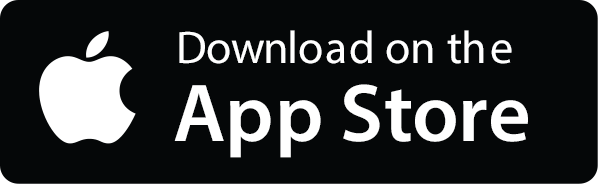
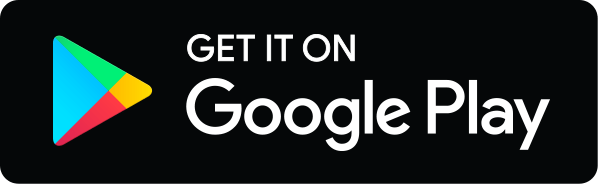
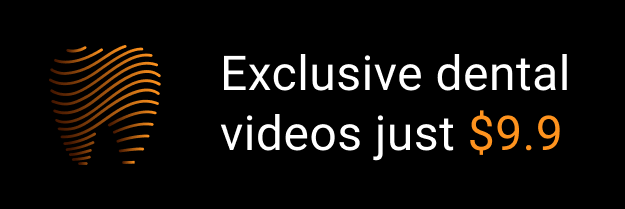