Highlights
- •
Anisotropic corrosion resistance was found in SLM-processed Co-Cr alloys.
- •
Selective corrosion occurred along the molten pool boundaries (MPBs).
- •
The MPB area was dependent on the building plane, resulting in the anisotropy.
- •
Post-fabrication heat treatment effectively reduced anisotropy by eliminating MPBs.
- •
Excessive heat treatment at high temperature produced less-protective passive films.
Abstract
Objectives
The application of selective laser melting (SLM) to dentistry has been rapidly expanding; however, SLM-processed parts possess orientation-dependent properties (i.e., anisotropy) that can affect the long-term reliability of the dental prostheses. This study aimed to evaluate the anisotropic corrosion response of SLM-processed Co–Cr–Mo alloys under various heat treatments.
Methods
Samples fabricated via SLM along the horizontal plane (x-y plane) and vertical plane (x-z plane), with respect to the build direction, were subjected to various heat treatments. The resulting microstructures of the samples were characterized, and their corrosion properties were evaluated using anodic polarization and immersion tests.
Results
All samples showed similar transpassive behavior of the polarization curves. However, the immersion tests showed that the as-built x-z plane samples released significantly more metal ions than those fabricated on the x-y plane because of the larger area of preferentially corroded molten pool boundaries (MPBs) in the x-z plane samples. Our results further demonstrated that the heat treatments eliminated the MPBs, resulting in isotropic corrosion properties. However, excessive heat treatment at high temperatures induces the formation of coarse precipitates, resulting in a less-protective passive film.
Significance
The post-build heat treatments at temperatures that eliminate the MPBs are effective in reducing anisotropic corrosion behavior, and the lowest possible temperature is suitable for reducing the amount of released metal ions. These findings are expected to facilitate the application of SLM in dentistry to allow fast and precise production of prosthetic devices.
1
Introduction
Selective laser melting (SLM) technology produces three-dimensional (3D) metal parts by layer-by-layer melting of metal powders using a scanning laser. This method enables rapid, semi-automatic, and precise fabrication of complex geometries; thus, SLM techniques have been widely applied to produce metal dental prostheses [ , ]. In particular, Co–Cr alloys prepared by SLM are increasingly used for metal copings [ ], removable partial denture frameworks [ ], and implant-supported frameworks [ ] owing to their good mechanical properties [ ] and biocompatibility [ ]. However, SLM-fabricated parts usually undergo a complex cyclic thermal history consisting of directional heat extraction, repeated melting, and rapid solidification, which creates a variety of orientation-dependent properties (i.e., anisotropy) [ , ]. In Co–Cr alloys, anisotropic microstructures, such as the epitaxial growth of columnar grains and <001> fiber texture along the build direction (BD), and anisotropic mechanical properties, determined through tensile and fatigue tests, were reported in previous studies [ , ].
Anisotropic corrosion properties have also been observed, where different behavior was measured in the different planes of SLM-produced Al–12Si [ ] and Ti–6Al–4 V [ ] alloys. However, to the best of our knowledge, no previous studies investigated the anisotropy in the corrosion properties of SLM-processed Co–Cr alloys along different planes. The surfaces of dental prostheses prepared by SLM consist of various orientation planes with respect to the BD. Therefore, the corrosion properties of the surfaces should vary depending on the site because the microstructure of the alloy depends on the sample plane (i.e., the horizontal and vertical planes with respect to the BD). The oral environment is a harsh system that promotes the corrosion of dental alloys. Metal ions and by-products released in the corrosion process can be harmful to the patient [ ]; thus, for clinical safety, the corrosion resistance of different planes with respect to the BD needs to be investigated.
As another well-known disadvantage of SLM, SLM-manufactured parts easily accumulate residual stress due to the rapid heating and cooling of the sample during fabrication, which negatively affects their dimensional accuracy and mechanical properties [ ]. Therefore, post-fabrication heat treatments are normally required to relieve residual stresses and improve mechanical properties [ ]. However, such heat treatments induce microstructural changes, which can affect the corrosion resistance. A previous study investigated the corrosion properties of SLM-processed Co–Cr alloys before and after porcelain firing, and reported that these specimens exhibited similar corrosion behavior at both pH 5.0 and 2.5, and had significantly better corrosion resistance than traditional cast specimens [ ]. However, the holding time at high temperature during porcelain firing is short and differs from that in post-fabrication heat treatment to relieve residual stress [ ]. Conversely, another study performed immersion tests with SLM-processed Co–Cr alloys after solution treatments at 1323 K (1050 °C), 1423 K (1150 °C), and 1473 K (1200 °C) for 1 h followed by water quenching, and at 1423 K (1150 °C) for 1 h followed by furnace cooling [ ]. The samples heated to 1423 K (1150 °C) and furnace cooled resulted in a larger amount of released metals due to the presence of coarse precipitates, as compared to the as-built samples and the water-quenched heat-treated samples. However, no studies exist that comprehensively evaluated the influence of various heat treatments followed by furnace cooling on the corrosion properties of SLM-processed Co–Cr alloys and compared their performance with traditional cast sample. The study of furnace cooling is important as it is used in dentistry after heat treatment to retain the dimensional accuracy of the prosthesis.
In this study, SLM-processed Co–Cr–Mo alloy samples were fabricated with either the horizontal or vertical plane parallel to the BD, and various heat treatments were performed, followed by furnace cooling. The microstructures and corrosion properties of the SLM-processed Co–Cr–Mo alloy samples were evaluated, focusing on the anisotropy with respect to the planes and the influence of various heat treatments. The results were evaluated through a comparison with traditional cast samples. The first null hypothesis of this study was that there would be no difference in corrosion response between the different planes (x-y or x-z planes) for the same heat-treatment conditions. The second null hypothesis was that the different heat treatment conditions would not influence the corrosion resistance.
2
Materials and methods
2.1
Specimen preparation
Commercially available Co–Cr–Mo alloy powders (MP1, EOS, Krailling, Germany) composed of 60–65 wt.% Co, 26–30 wt.% Cr, 5–7 wt.% Mo, and <1 wt.% Si, Mn, Fe, C, and Ni, were used. Cubic samples (10 mm × 10 mm × 10 mm) were prepared using an SLM machine equipped with a fiber laser (EOSINT M280, EOS, Krailling, Germany). The SLM machine was operated using standard deposition parameters for MP1 under a laser power of 195 W and a nitrogen atmosphere. The laser scan pattern was rotated by an angle of 67° between successive layers along the z-direction ( Fig. 1 ). Samples were heated to 1023 K (750 °C) or 1423 K (1150 °C) at a rate of 333 K/min and maintained at this temperature for 1 or 6 h under an Ar atmosphere in a furnace. Subsequently, the samples were slowly cooled in the furnace to room temperature (approximately 298 K). Based on their thermal history, samples were denoted as “as-built”, “HT750-6H” (heat-treated at 1023 K (750 °C) for 6 h), “HT1150-1H” (heat-treated at 1423 K (1150 °C) for 1 h), and “HT1150-6H” (heat-treated at 1423 K (1150 °C) for 6 h). To investigate the anisotropy, the built samples were cut along the x-y and x-z planes, as defined in Fig. 1 , with a slow-speed diamond cutter to form rectangular specimens (10 mm × 10 mm × 2 mm). For comparison, samples with the same shape were also prepared by conventional dental casting (henceforth referred to as ‘as-cast’ samples). Commercially available Co–Cr–Mo alloy ingots (Cobaltan, Shofu, Kyoto, Japan) composed of 63 wt.% Co, 29 wt.% Cr, 6 wt.% Mo, and <1 wt.% Si, Mn, N, and C, were used.

2.2
Microstructural characterization
Sample microstructures were investigated using confocal laser scanning microscopy (CLSM; OLS4000, Olympus, Japan), scanning electron microscopy combined with energy dispersive X-ray spectroscopy (SEM-EDS; S-3400NX, Hitachi, Japan) with an accelerating voltage of 15 kV and an emission current of 65 mA, and transmission electron microscopy (TEM; JEM-2100 F; JEOL Ltd., Japan) operated at 200 kV. The elemental distributions of the specimens were determined using field-emission electron probe microanalysis (FE-EPMA; JXA-8530 F, JEOL Ltd., Tokyo, Japan) at an accelerating voltage of 15 kV. For CLSM, SEM-EDS, and FE-EPMA, the samples were polished to a mirror surface with waterproof emery paper (up to 1000 grit), a 9 μm diamond suspension, and a 0.04 μm colloidal silica suspension, followed by electropolishing in a solution of H 2 SO 4 /CH 3 OH (5:95) at 16–20 V and 263–268 K. For TEM, the samples were mechanically ground with waterproof emery paper (up to 1000 grit) to a thickness of 0.3 mm. Small 3-mm diameter disks were punched out from each sample. They were ground with a 9-μm diamond suspension to a thickness of 0.1 mm and further thinned with a twin-jet electrochemical polisher using a solution of HClO 4 /CH 4 (CH 2 ) 3 OH/CH 3 OH (5:35:60) at 223 K under an applied voltage of 20 V.
2.3
Anodic polarization test
Anodic polarization measurements were performed in a 0.9 wt% NaCl aqueous solution using a potentiostat (HABF-501 G, Hokuto Denko, Japan) and a function generator (HB-111, Hokuto Denko, Japan). Prior to performing the measurements, each specimen was polished with waterproof emery paper (up to 800 grit) and ultrasonically cleaned with acetone and isopropanol for 10 min. Subsequently, the entire specimen was immersed in ultrapure water for 24 h at room temperature to stabilize the passive layer. A saturated calomel electrode and a Pt plate were used as a reference and a counter electrode, respectively. The exposed area of the specimen in contact with the electrolyte was 0.35 cm 2 (6.7 mm in diameter). After immersing the specimen in the test solution at 37 °C (to simulate body temperature), the open circuit potential (OCP) was measured for 10 min. Thereafter, a gradient anodic potential was applied at a constant sweep rate of 1 mV s -1 from the initial potential, which was 100 mV lower than the final OCP value. The measurement was stopped when the current density reached 1 mA cm -2 . This measurement was performed on five specimens under the same conditions (N = 5). The corrosion potential (that just before the onset of polarization) was measured from the obtained polarization curves. The surface morphologies of the specimens after the anodic polarization tests were observed using CLSM. Additionally, the free corrosion potential was monitored for 24 h using a potentiostat.
2.4
Immersion tests
The immersion tests were performed in accordance with the ISO 10271 standard. The specimens were embedded in an epoxy resin (EpoFix, Struers, USA) to ensure that only the plane of interest was exposed to the solution. The epoxy resin was composed of 60–90% bis[4-(2,3-epoxypropoxy) phenyl] propane and 26–30% oxirane, mono[(C12-14-alkyloxy) methyl] derivs. Subsequently, the specimens were polished with waterproof emery paper (up to 800 grit) and immersed in ultrapure water for 24 h at room temperature. The polished specimens were placed in polypropylene bottles, and 10 mL of a simulated body fluid (SBF)was then poured into each bottle. To prepare the SBF, 10 g of lactic acid and 5.85 g of NaCl were dissolved in 300 mL of water, which was then diluted to 1000 mL. The pH of the test solution was measured in advance and confirmed to be 2.3 ± 0.1 immediately after being prepared. Thereafter, the specimens were immersed in the solution for 604.8 ks (7 d) at 310 K. Furthermore, the concentrations of metal ions (Co, Cr, and Mo) were then measured using an inductively coupled plasma atomic emission spectrometer (ICP-AES, ICPS-7000 ver. 2, Shimadzu, Japan). The samples were collected from the solution, rinsed with ultrapure water, and stored. Single-element standard solutions of Co, Cr, and Mo (1000 ppm) were purchased from Kanto Chemical Co., Inc., Japan. These solutions were diluted before use as the intermediate standard solution (1 ppm, 100 ppb, 10 ppb) to establish calibration curves. The detection limits for Co and Cr were approximately 1 mg m -2 . The concentrations of ions released from the specimens were determined by subtracting the values obtained for the blank measurement (test solution with no specimen). The amount of metal released (mg m -2 ), C r , was calculated using the following formula: C r = V s (C s -C b )/A, where V s is the volume of the solution (0.01 l), C s is the average metal concentration in the test solution containing the sample (ppm), C b is the average metal concentration in the blank group (ppm), and A is the surface area of the specimen (m 2 ). The test was performed using three bottles under the same conditions (N = 3).
2.5
Statistical analysis
Statistical analysis was conducted using statistical software (SPSS, v24.0; IBM Corp., USA). To test the first null hypothesis, the Student’s t-test was used to examine the difference in the amount of released metal ions and the corrosion potential between the different build planes (x-y and x-z planes) within each group. Two-way ANOVA was used to test the second null hypothesis that the heat treatment condition would not influence the amount of released metal ions and the corrosion potential. As the interaction effect between the heat-treatment condition and the different planes was significant, a Bonferroni correction was applied to analyze the simple effects of the heat treatment condition. Here, p < 0.05 was considered statistically significant.
3
Results
3.1
Microstructures
Fig. 2 depicts 3D microscopy images of the microstructures of the as-built SLM alloys. In the CLSM images, regular laser-melted tracks corresponding to the alternating 67° filling strategy were observed on the cross-section, as shown by the x-y cross-section ( Fig. 2 (a)). In the x-z/y-z cross-section, the layered half ellipses accumulated along the z-axis were identified as melt-pool traces, which were formed via the layer-by-layer melting of the metal powders. In the SEM images, numerous fine cellular dendrites were observed in the XY cross-section. In contrast, fine columnar dendritic structures were observed in the XZ/YZ cross-section.
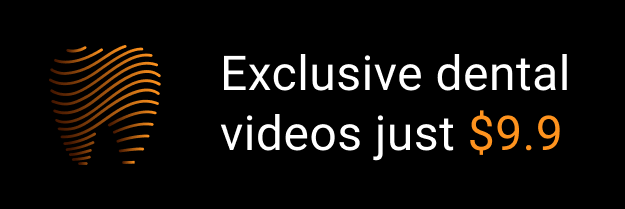