Introduction
The aim of this study was to evaluate the cytotoxic effects of orthodontic mini-implants on gingival fibroblasts and osteoblasts.
Methods
The orthodontic mini-implants used in this study were Orthodontic Mini Implant (Leone, Florence, Italy), MTN (MTN, Istanbul, Turkey), AbsoAnchor (Dentos, Daegu, South Korea), IMTEC Ortho (3M Unitek, IMTEC, Ardmore, Okla), VectorTAS (Ormco, Glendora, Calif). The materials were incubated in Dulbecco’s modified eagle’s culture medium for 72 hours according to ISO 10993-5 standards (surface area-to-volume ratio of the specimen to cell-culture medium, 3 cm 2 /mL). A real-time cell analyzer (xCELLigence, Roche Applied Science, Mannheim, Germany; ACEA Biosciences, San Diego, Calif) was used to evaluate cell survival. After seeding 200 μL of the cell suspensions into the wells of the E-plate 96, gingival fibroblasts were treated with bioactive components released by the metallic materials and monitored every 15 minutes for 190 hours. For the proliferation experiments, the statistical analyses used were 1-way analysis of variance and Tukey-Kramer multiple comparisons tests.
Results
There was no significant differences between the human gingival fibroblast cell indexes of the control and study groups ( P >0.05). When evaluated at 27 and 96 hours, only the VectorTAS mini-implants showed statistically significant decreases in the M3T3 cell index ( P <0.001) compared with the control group. No significant differences were found among the control and all study groups ( P >0.05). Furthermore, the Leone and MTN mini-implants showed statistically significant decreases ( P <0.001) at 190 hours. Also, the VectorTAS mini-implants demonstrated a significant decline ( P <0.05) at the same time in the M3T3 cell index.
Conclusions
These findings provide fundamental knowledge and new insights for future design and development of new biocompatible titanium alloys for orthodontic mini-implants and temporary anchorage devices.
Anchorage has long been a challenge since the introduction of fixed appliances in orthodontics. Typically, the orthodontic movement of a tooth is anchored by a large group of teeth to minimize unwanted movements of the anchoring teeth. Adequate anchorage becomes difficult when posterior teeth are missing. Intraoral and extraoral auxiliary devices can be used to assist movement, but the effectiveness of these measures depends on the patient’s cooperation.
Dental implants have gained wide acceptance as direct and indirect orthodontic anchorage and have been confirmed to provide absolute and rigid anchorage clinically and histologically. However, dental implants have limited anatomic placement options and require a precise 2-stage protocol and a 3 to 6 month healing period. In addition, conventional dental implants are troublesome for patients because of the severity of the surgery.
In 1997, Wehrbein et al and Kanomi used mini-implants to anchor orthodontic movement. After these 2 studies, mini-implants were introduced as temporary skeletal anchorage devices and have been gaining popularity because their small size allows more placement sites in the oral cavity and reduces bone-healing time.
In current clinical orthodontic practice, orthodontic mini implants or temporary anchorage devices are widely used for distal or mesial movement of teeth; anterior retraction or protraction of teeth; intrusion; extrusion; uprighting; corrections of midlines, transversal problems, occlusal cant, and open bite or deepbite; skeletal anchorage for mandibular or maxillary advancement, and so on. These devices are applied to the maxillary or mandibular base, maxillary or mandibular alveolar region, palate, zygoma, and retromolar region. Several studies about orthodontic mini-implants and temporary anchorage devices dramatically led to improved outcomes, and the treatment protocols changed in the last decade.
Although the dramatic developments and improvements of orthodontic mini-implants and temporary anchorage devices are satisfying and amazing, their biocompatibility is an important question for orthodontists today. No data are available in the orthodontic literature regarding the biocompatibility of commercially available orthodontic mini-implants and temporary anchorage devices. Therefore, the aim of this study was to evaluate the cytotoxic effects of 5 orthodontic mini-implants and temporary anchorage devices on human gingival fibroblasts and osteoblasts (MC3T3-E1).
Material and methods
The orthodontic mini-implants selected were Orthodontic Mini Implant (Leone, Florence, Italy), MTN (MTN, Istanbul, Turkey), AbsoAnchor (Dentos, Daegu, South Korea), IMTEC Ortho (3M Unitek, IMTEC, Ardmore, Okla), VectorTAS (Ormco, Glendora, Calif). The microstructure of the orthodontic mini-implants and temporary anchorage devices was investigated by using scanning electron microscopy and phase identification with energy dispersive x-ray analysis. A scanning electron microscope (Leo Evo-40 VP; Leo Electron Microscopy Ltd, Cambridge, UK) operated at 20 kV and an energy dispersive x-ray spectroscope (RÖNTEC, GmbH, Berlin, Germany) were used. X-ray images of the orthodontic mini-implants are shown in Figure 1 , and the details related to these products and their components are listed in Table I .
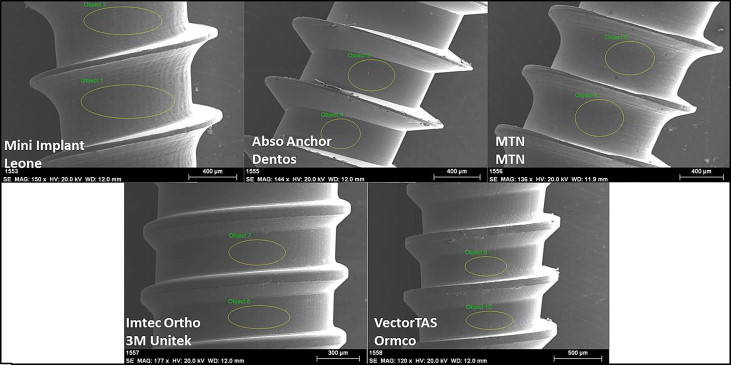
Brand | Atomic percentage | Manufacturer | |||||||
---|---|---|---|---|---|---|---|---|---|
Carbon | Aluminum | Sulfur | Chromium | Iron | Nickel | Titanium | Vanadium | ||
Mini Orthodontic Implant | 6.71 | 1.34 | 2.53 | 18.45 | 58.13 | 12.42 | – | – | Leone, Florence, Italy |
AbsoAnchor | 5.82 | 8.56 | – | 0.77 | 0.84 | 0.42 | 83.41 | 0.18 | Dentos, Daegu, South Korea |
MTN | 6.27 | 8.21 | – | 0.54 | 0.49 | 0.51 | 83.81 | 0.15 | MTN, Istanbul, Turkey |
IMTEC Ortho | 4.56 | 8.83 | – | 0.43 | 0.46 | 0.49 | 84.83 | 0.32 | 3M Unitek, IMTEC, Ardmore, Okla |
VectorTAS | 4.19 | 8.12 | – | 0.62 | 0.47 | 0.42 | 86.56 | 0.13 | Ormco, Glendora, Calif |
Six samples were used for each group. The specimens were sterilized by using ultraviolet light and immersed in 7 mL of culture medium for 24 hours at 37°C to extract metal ions or cytotoxic substances. The culture medium containing the material extracts was filtered in a sterile environment for use on the cell cultures. The test procedures for this investigation were regulated by ISO-standard 10993-5.
The xCELLigence system (Roche Applied Science, Mannheim, Germany; ACEA Biosciences, San Diego, Calif) consists of 4 main components: the impedance-based real-time cell analyzer analyzer, the real-time cell analyzer single-plate station, the real-time cell analyzer analyzer computer with integrated software, and the disposable E-plate 96. The real-time cell analyzer analyzer single-plate station fits inside a standard tissue-culture incubator. The electronic impedance of the sensor electrodes is measured to allow monitoring and detection of physiologic changes of the cells on the electrodes. The voltage applied to the electrodes during real-time cell analyzer analyzer measurement was about 20 mV root mean square. The impedance measured between electrodes in a well depends on electrode geometry, ion concentration in the well, and whether cells are attached to the electrodes. In the absence of cells, electrode impedance is mainly determined by the ion environment both at the electrode-solution interface and in the bulk solution. In the presence of cells, cells attached to the electrode sensor surfaces act as insulators and thereby alter the local ion environment at the electrode-solution interface, leading to increased impedance. Thus, the more cells that are growing on the electrodes, the larger the value of electrode impedance. The data expressed in cell index units can be exported to Excel (Microsoft, Redmond, Wash) for any mathematical analysis.
Human gingival fibroblasts were isolated from human gingival connective tissue of systemically healthy subjects. The human gingival fibroblasts were obtained from samples of gingiva removed for crown lengthening purposes. All patients gave informed consent before providing the samples. This protocol was approved by Ethics Committee of the Faculty of Dentistry of Selçuk University, Konya, Turkey. Briefly, gingival tissues were cut into small pieces, rinsed with biopsy media, placed in tissue culture dishes, and incubated in the biopsy medium in a humidified atmosphere of 95% air and 5% carbon dioxide at 37°C overnight. The next day, the biopsy medium was replaced with culture medium (Dulbecco’s modified eagle’s medium [Biological Industries, Beit Haemek, Israel] with 10% fetal bovine serum, 100 units/mL of penicillin, 100 μg/mL of streptomycin). After reaching confluency, the cells were passaged with 0.25% trypsin and 0.1% ethylene diaminotetraacetic acid. Gingival fibroblasts were used between the fourth and sixth passages for all experiments.
MC3T3-E1 (subclone 4), an immortalized cell line provided by Dr Martha J. Somerman from the University of Washington and Renny T. Franceschi from the University of Michigan that was derived from newborn mouse calvaria, was used for the evaluation. The cells were incubated in α-MEM with 10% fetal bovine serum, 1% penicillin-streptomycin, and L-glutamine at 37°C in a humidified atmosphere of 5% carbon dioxide in air.
The materials were incubated in the culture medium for 72 hours according to ISO 10993-5 standards (surface area-to-volume ratio of the specimen to cell-culture medium, 3 cm 2 /mL). Human gingival fibroblasts and MC3T3-E1 were maintained with Dulbecco’s modified eagle’s medium containing 10% fetal bovine serum. The real-time cell analyzer was used to evaluate cell survival, according to the supplier’s instructions. After seeding 200 μL of the cell suspensions into the wells (10,000 cells/well) of the E-plate 96, human gingival fibroblasts and MC3T3-E1 were treated with bioactive components released by the metallic alloy material and monitored every 15 minutes for 190 hours.
Statistical analysis
All calculations were obtained by using the real-time cell analyzer integrated software, which performs a curve-fitting of selected “sigmoidal dose-response equation” to the experimental data points. Data are represented as mean (mmol/L) ± SD (n = 5).
For the proliferation experiments, the statistical analyses used were 1-way analysis of variance (ANOVA) and Tukey-Kramer multiple comparison tests. The data are represented as means and standard deviations. A value of P <0.05 was considered to be statistically significant.
Results
First, we determined the optimal concentrations for cell proliferation and viability measurements. To this end, 40,000, 20,000, 10,000, and 5000 cells per well were seeded in the E-Plate 96, and the impedance was determined.
According to ANOVA, when left to elute for 25, 34, and 60 hours, there were no significant differences between the human gingival fibroblast cell indexes of the control and study groups ( P >0.05). But all testing groups were significantly different from the untreated control group at 190 hours ( P <0.01) ( Table II and Fig 2 ).
Group (n = 6) | 25 h Mean ± SD |
34 h Mean ± SD |
60 h Mean ± SD |
190 h Mean ± SD |
---|---|---|---|---|
Control | 3.33 ± 0.51 | 3.64 ±0.27 | 2.83 ± 0.40 | 1.79 ± 0.11 |
Leone | 3.00 ± 0.00 | 3.28 ± 0.21 | 3.03 ± 0.08 | 2.47 ± 0.10 ∗ |
MTN | 3.33 ± 0.51 | 3.89 ± 0.24 | 3.12 ± 0.18 | 2.32 ± 0.10 ∗ |
AbsoAnchor | 3.66 ± 0.51 | 3.96 ± 0.29 | 3.09 ± 0.20 | 2.75 ± 0.85 ∗ |
IMTEC Ortho | 3.33 ± 0.51 | 3.62 ± 0.25 | 2.52 ± 0.20 | 2.00 ± 0.09 † |
VectorTAS | 2.83 ± 0.40 | 3.19 ± 0.42 | 3.16 ± 0.40 | 2.30 ± 0.04 ∗ |
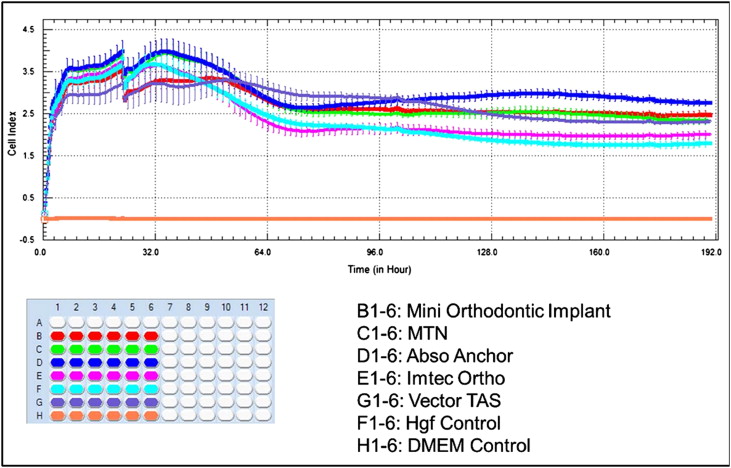
When evaluated at 27 and 96 hours, only the VectorTAS mini-implants showed statistically significant decreases in MC3T3-E1 cell index ( P <0.001) compared with the control group. There were no significant differences between the other study groups ( P >0.05). No significant differences were found among the control and all study groups ( P >0.05) ( Table III and Fig 3 ).
Group (n = 6) | 27 h Mean ± SD |
60 h Mean ± SD |
96 h Mean ± SD |
190 h Mean ± SD |
---|---|---|---|---|
Control | 9.07 ± 0.45 | 11.66 ± 0.51 | 12.48 ± 0.24 | 11.35 ± 0.37 |
Leone | 8.59 ± 0.61 | 11.83 ± 0.71 | 11.96 ± 0.46 | 4.45 ± 2.07 ∗ |
MTN | 9.19 ± 0.19 | 12.46 ± 0.51 | 12.04 ± 0.21 | 6.95 ± 2.13 ∗ |
AbsoAnchor | 9.15 ± 0.36 | 11.71 ± 0.45 | 12.08 ± 0.49 | 9.30 ± 1.85 |
IMTEC Ortho | 8.46 ± 0.65 | 11.50 ± 0.54 | 11.91 ± 0.49 | 9.67 ± 1.15 |
VectorTAS | 7.76 ± 0.44 ∗ | 10.83 ± 0.40 | 11.10 ± 0.62 † | 8.50 ± 1.37 † |
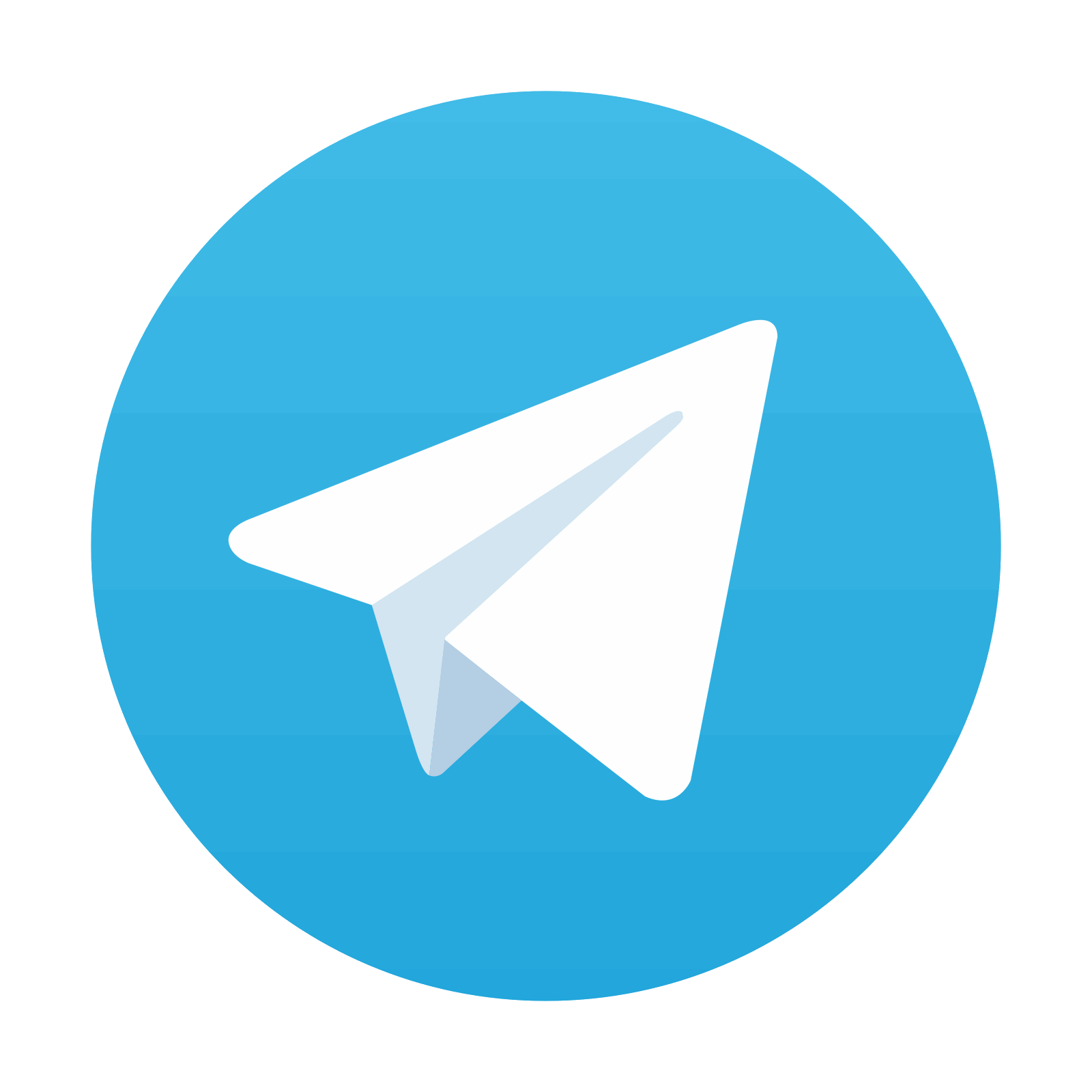
Stay updated, free dental videos. Join our Telegram channel

VIDEdental - Online dental courses
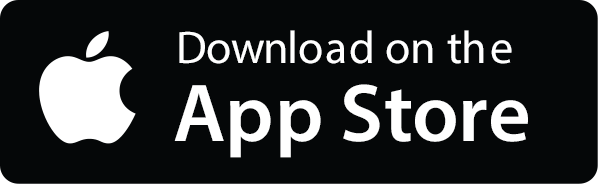
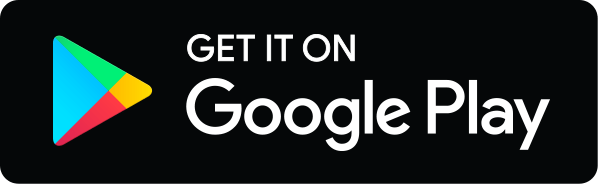
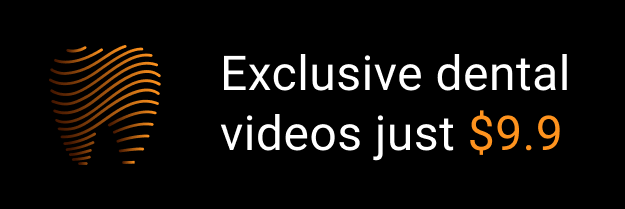