Radiographic Interpretation
Interpretation of information captured by radiographic imaging modalities is central to the diagnostic process. It is very important to capture a diagnostically useful image using appropriate exposure parameters and view it with interactive manipulation of brightness and contrast or window/level (for cone beam computed tomography [CBCT] studies) in an optimal environment to adequately evaluate anatomy and diagnose pathoses. Accurate interpretation of root and canal morphology, determination of radiographic canal length, diagnosis of radicular and periradicular disease ( Fig. 2-1 ), and postsurgical and long-term evaluation of the outcome of endodontic treatment are some of the routine diagnostic imaging tasks in endodontics. Systematic and methodical interpretation processes must be followed for all images. Recognition of anatomy, anatomic variants, and pathologic conditions or deviations from normal is important. Various imaging modalities exist in radiology. Some use ionizing radiation, whereas others use ultrasonic waves (ultrasonography, or US) or powerful external magnetic fields (magnetic resonance imaging, or MRI). Interventional and noninterventional imaging modalities are also available. Imaging modalities using ionizing radiation are most frequently used in endodontic diagnoses. The different image capture modalities include conventional intraoral film and the more modern digital receptors.

Imaging Modalities
Digital radiography using electronic sensors or photostimulable phosphor (PSP) plates is widely used in endodontics. The advantages of using digital sensors over film are many. Significant advantages include noteworthy dose reduction (especially in comparison with D-speed film used with round collimation); almost instantaneous generation of high-resolution digital images with resolution approaching or equaling that of film for specific diagnostic tasks; the ability to postprocess images for enhanced diagnostic outcomes; elimination of variables associated with wet processing of conventional film; ease of transmission and of archiving and retrieving images from databases or picture archiving and communication systems (PACS); facilitation of use of an all-electronic patient record ; reduced exposure of personnel to hazardous chemicals; and reduced environmental impact.
Digital imaging modalities in endodontics use different image capture technologies, which include a charge-coupled device (CCD), a complementary metal oxide semiconductor (CMOS), or a PSP (also sometimes referred to as an indirect acquisition modality). Film images also can be digitized using a flatbed scanner or CCD/CMOS-based cameras mounted on a camera stand, with images captured using a frame grabber from a mounted, lighted platform.
CCD-based solid-state sensors were used extensively in endodontics initially. However, the earlier generation sensors had a smaller active area and limited x-ray absorption and conversion efficiency, in addition to being bulky. Sensors use an array of radiation-sensitive elements that generate electric charges proportionate to the amount of incident radiation. To reduce the amount of radiation needed to capture an image, a light-sensitive array was developed that uses a scintillation layer laid on top of the CCD chip or added with a fiberoptic coupling. The generated charge is read out in a “bucket brigade” fashion and transferred to an analog-to-digital converter in the frame grabber assembly of the workstation. The digital information is processed, and an image is formed. CMOS-based sensors, on the other hand, have an active transistor at each element location. The area available for signal generation is relatively less, and there is a fixed pattern noise. These sensors are less expensive to manufacture and have been shown to be equally useful for specific diagnostic tasks. Unlike the CCD, the CMOS chip requires very little electrical energy; therefore, no external power supply is needed to support USB utilization, and wireless applications are feasible. Wireless sensors are available ( Fig. 2-2 ). However, radiofrequency interference may be a problem with these sensors. The current WiFi sensor is less bulky and has a wire attached to it that enables transmission via 802.11 b/g standard. It uses a lithium-ion polymer battery that can last for approximately 100 exposures.

Yet another type of sensor uses PSPs for image capture. PSP technology is also referred to as computed radiography (CR). Unlike the CCD and CMOS sensors, PSP sensors are wireless. The phosphor is activated by a process called doping, which enables charges to be generated and stored when exposed to radiation. A latent image is stored in the sensor, and a PSP reader with a laser beam of specific wavelength is used to read out the image. Previously captured images can be erased by exposing the PSP sensor to white light. PSP plates can be damaged easily by scratching, but they are not as expensive as CCD or CMOS sensors. Incomplete erasure of the image can lead to ghost images when the plate is reused, and delayed processing can result in a decrease in image clarity. PSP-based sensors are used in high-volume scenarios. Spatial resolution is lower with this type of sensor, but it has a wider dynamic range. These sensors can tolerate a wider range of exposures to produce a diagnostically useful image.
Radiation dose continues to be a concern with all imaging studies. The lowest possible dose must be delivered for each study. Most dental offices would not be in compliance with the latest recommendations of the National Council for Radiation Protection (NCRP) on reducing the radiation dose from intraoral radiographs ( Box 2-1 ). Two terms have been specifically defined in the NCRP’s report. The terms shall and shall not indicate that adherence to the recommendation would be in compliance with the standards of radiation safety. The terms should and should not indicate prudent practice and acknowledge that exceptions may be made in certain circumstances. In addition, the report establishes nine new recommendations for image processing of conventional film.
- 1.
Dentists must examine their patients before ordering or prescribing x-ray images (this is not a new guideline).
- 2.
The use of leaded aprons on patients shall not be required if all other recommendations in this report are rigorously followed (read full Report #145).
- 3.
Thyroid shielding shall be used for children and should be provided for adults when it will not interfere with the examination (e.g., panoramic imaging).
- 4.
Rectangular collimation of the beam, which has been recommended for years, shall be routinely used for periapical radiographs. Each dimension of the beam, measured in the plane of the image receptor, should not exceed the dimension of the image receptor by more than 2% of the source-to-image receptor distance. Similar collimation should be used, when feasible, for bitewing radiographs.
- 5.
Image receptors of speeds slower than ANSI speed Group E films shall not be used for intraoral radiography. Faster receptors should be evaluated and adopted if found acceptable. For extraoral radiography, high-speed (400 or greater) rare earth screen-film systems or digital-imaging systems of equivalent or greater speed shall be used.
- 6.
Dental radiographic films shall be developed according to the film manufacturer’s instructions using the time-temperature method. In practical application, this means that sight development (reading wet x-ray films at the time of the procedure) shall not be used.
- 7.
Radiographic techniques for digital imaging shall be adjusted for the minimum patient dose required to produce a signal-to-noise ratio sufficient to provide image quality to meet the purpose of the examination.
- 8.
Clinicians designing new offices or remodeling existing locations will need shield protection to be provided by a qualified expert.
A strong argument can be made for clinicians to switch to a direct digital radiography (DDR) system to avoid all the drastic changes necessary to ensure compliance with the new recommendations. Even though restriction of an intraoral dental x-ray beam is mandated by federal law to a circle no greater than 7 cm, rectangular collimation has been proven to significantly reduce the radiation dose to the patient.
The American Dental Association (ADA) Council on Scientific Affairs has made the following statement:
Tissue area exposed to the primary x-ray beam should not exceed the minimum coverage consistent with meeting diagnostic requirements and clinical feasibility. For periapical and bitewing radiography, rectangular collimation should be used whenever possible because a round field beam used with a rectangular image receptor produces … unnecessary radiation exposure to the patient.
Image Characteristics and Processing
Spatial resolution achieved with current generation digital sensors is equally good or better than that of conventional intraoral radiographic film. Intraoral film has a resolution of 16 line pairs per millimeter (lp/mm) as measured using a resolution tool, and it increases to 20 to 24 lp/mm with magnification. Spatial resolution is defined as the ability to display two objects that are close to each other as two separate entities. Contrast resolution is defined as the ability to differentiate between areas on the image based on density. Most diagnostic tasks in endodontics require a high-contrast resolution. However, image quality is not just a function of spatial resolution. The choice of appropriate exposure parameters, sensor properties, the image processing used, and viewing conditions and modalities directly affect diagnostic accuracy.
Postprocessing of images may be carried out to alter image characteristics. Radiographs need not be reexposed if image quality is not adequate. Diagnostic information can be teased out of the image if appropriate image processing is used. However, the original image must be acquired with optimal exposure parameters to accomplish meaningful image processing. Suboptimally exposed images cannot be processed to yield diagnostic information, which may lead to a reduction in the diagnostic accuracy of the image. Image enhancement must be task specific. Signal-to-noise ratio (SNR) must be optimized to extract necessary information from the image. The bit-depth of images also has a direct relationship to image quality. It indicates the number of shades of gray that the sensor can capture for display. For example, an 8-bit image can depict 256 shades of gray. Most sensors are 12 or 14 bits in depth, capturing 4,096 or 65,536 shades of gray, respectively. If the sensor captures several thousand shades of gray, the image can be manipulated through enhancement techniques to display those shades of gray that best depict the anatomy of interest. The human visual system is limited in the number of shades of gray that can be read at any point in time. Therefore, image enhancement is a must for all images, so as to delineate signals of interest through manipulation of the grayscale. Most endodontic tasks require a high contrast and thus a shorter grayscale.
Digital radiographs can be saved in different file formats. Several file formats are available: DICOM ( D igital I maging and C ommunications in M edicine); tiff ( t agged i mage f ile f ormat); jpeg ( j oint p hotographic e xperts g roup); gif ( g raphics i nterchange f ormat); BMP (Windows’ bitmap image file); PNG ( p ortable n etwork g raphics); and so on. There also are several proprietary formats. “Lossy” and “lossless” compression schemes can be used for saving images, although lossless compression is preferred.
Digital Imaging and Communications in Medicine (DICOM)
DICOM is a set of international standards established in 1985 by the American College of Radiology (ACR) and the National Electrical Manufacturers Association (NEMA) to address the issue of vendor-independent data formats and data transfers for digital medical images. The ADA has promoted the interoperability of dental images through the efforts of its Working Group 12.1.26. DICOM serves as a standard for the transferal of radiologic images and other medical information between computers, allowing digital communication between systems from various manufacturers and across different platforms (e.g., Apple iOS or Microsoft Windows). The DICOM standard provides for several hundred attribute fields in the record header, which contains information about the image (e.g., pixel density, dimensions, and number of bits per pixel), in addition to relevant patient data and medical information. Although earlier versions did not specify the exact order and definition of the header fields, each vendor is required to publish a DICOM conformance statement, which gives the location of pertinent data. The big hurdle is to support medical and dental consultations between two or more locations with different imaging software. With DICOM in place, dental clinicians can change vendors and maintain database interoperability. Most software vendors are striving to achieve full DICOM compliance, and some have achieved at least partial compliance. However, proprietary DICOM images are still produced in different systems, with the capability to export in universal DICOM format as needed. Diagnostic images are best saved as DICOM files to preserve image fidelity or as tiff files with no compression. Diagnosis suffers when images undergo lossy compression.
Based on the DICOM model, the ADA Standards Committee on Dental Informatics has identified four basic goals for electronic standards in dentistry: (1) interoperability, (2) electronic health record design, (3) clinical workstation architecture, and (4) electronic dissemination of dental information. The dental profession must continue to promote DICOM compatibility so that proprietary software and file types do not hinder communication and risk making data obsolete.
Diagnostic Tasks in Endodontics
Working Length Determination
Digital imaging systems perform as well as intraoral film or better for working length determination. No significant difference was noted between measurements made on digital images. Older studies compared the early generation digital sensors with limited bit-depth to D-speed films, and the films showed better performance. It is important to analyze the type of sensor used, software, processing, video card and monitor, and viewing conditions to determine whether the sensor is good for a specific diagnostic task. Calibration improves the diagnostic accuracy. Likewise, the use of optimal processing parameters improves image quality to the extent of making a significant difference in the diagnostic outcome. For instance, density plot analysis was shown to help with endodontic file measurements. The major advantage of direct digital radiography (CCD, CMOS) is that the dose is significantly less compared with that required for film. The use of DDR, therefore, is justified when its performance is comparable to that of film with no statistically significant differences.
The three types of measurement generally available with digital imaging software are (1) linear measurement, the distance between two points in millimeters ( Fig. 2-3 ); (2) angle measurement, the angle between two lines; and (3) area measurement, the area of the image or a segment of the image. Because magnification and distortion errors play a significant role in the accuracy of two-dimensional (2D) radiographic measurement, both film and digital systems are subject to parallax error. However, a study that compared endodontic file length images of human teeth taken with a custom jig suggested that “measurement error was significantly less for the digital images than the film-based images.” This was true even though, as the authors pointed out, the measurement differences may not have been clinically significant. Sophisticated calibration algorithms are under development, and accurate measurement of parallel images should be more feasible in the future.

Diagnosis and Healing
Image enhancement of direct and indirect digital radiographs based on the diagnostic task at hand has been shown to increase diagnostic accuracy compared to film-based images, which cannot be enhanced. Posttreatment endodontic evaluation of healing of apical radiolucent areas is a challenge. Early changes indicating healing and bone fill are difficult to detect on conventional or digital radiographs. However, bone fill can be detected using more sensitive techniques, such as digital subtraction radiography, in which two images, separated in time but acquired with the exact same projection geometry and technique factors, can be subtracted from one another to tease out subtle changes in the periodontium and surrounding bone. Subtraction techniques are difficult to carry out in routine clinical practice because they are technique sensitive and can yield incorrect information if not performed accurately. Several studies have shown the usefulness of subtraction radiography using digital sensors.
Three-Dimensional Imaging
Computed tomography (CT) was introduced by Sir Godfrey Hounsfield in the 1970s. Tomography refers to “slice imaging,” in which thin slices of the anatomy of interest are captured and synthesized manually or using an algorithm. CT makes use of automated reconstruction. Medical-grade CT used a translate-rotate image acquisition scheme as the technology developed, but the modality always resulted in higher radiation dose delivery because of redundancy of data capture, in addition to longer scan times with the potential for motion artifact. Multiple detectors and x-ray sources were used in later generations of CT units to reduce scanning times. The increased radiation dose, artifacts from metallic restorations, cost of scanning, long acquisition times, and lack of adequate dental-specific software have been drawbacks limiting the use of the technology in dentistry until recently. The advent of cone beam volumetric computed tomography (CBVCT) introduced a faster, low-dose, low-cost, high-contrast imaging modality that could capture information in three dimensions using a limited field of view.
CBVCT, or cone beam CT (CBCT), is a relatively new diagnostic imaging modality that has been recently added to the endodontic imaging armamentarium. This modality uses a cone beam instead of a fan-shaped beam in multidetector computed tomography (MDCT), acquiring images of the entire volume as it rotates around the anatomy of interest. Compared with MDCT images, CBCT offers relatively high-resolution, isotropic images, allowing effective evaluation of root canal morphology and other subtle changes within the root canal system. Even though the resolution is not as high as that of conventional radiographs (18 microns), the availability of three-dimensional (3D) information, the relatively higher resolution, and a significantly lower dose compared to MDCT make CBCT the imaging modality of choice in challenging situations demanding localization and characterization of root canals.
The adoption of advanced imaging modalities such as CBCT for select diagnostic tasks is becoming popular with clinicians performing endodontic procedures. Two-dimensional grayscale images, whether conventional film based or digital, cannot accurately depict the full 3D representation of the teeth and supporting structures. In fact, traditional images are poor representations of even the pulpal anatomy. They grossly underestimate canal structure and often cannot accurately visualize periapical changes, especially where there is thick cortical bone, as in the presence of anatomic obstructions ( Fig. 2-4, A ). CBCT, however, allows the clinician to view the tooth and pulpal structures in thin slices in all three anatomic planes: axial, sagittal, and coronal. This capability alone allows visualization of periapical pathoses and root morphology previously impossible to assess ( Fig. 2-4, B and C ). Several tools available in CBCT, such as the ability to change the vertical or horizontal angulation of the image in real time, in addition to thin-slice, grayscale data of varying thicknesses, will never be available for conventional or even digital radiography. Furthermore, the use of CBCT data to view the region of interest in three anatomic planes of section at very low x-ray doses has never been as easy or accessible as it is today.

Microcomputed tomography (micro-CT) has also been evaluated in endodontic imaging. Comparison of the effects of biomechanical preparation on the canal volume of reconstructed root canals in extracted teeth using micro-CT data was shown to assist with characterization of morphologic changes associated with these techniques. Peters et al. used micro-CT to evaluate the relative performance of nickel-titanium (Ni-Ti) instruments after the shaping of root canals of varying preoperative canal geometry (for examples, see Chapter 6 ). A study to examine the potential and accuracy of micro-CT for imaging of filled root canals showed it to be a highly accurate and nondestructive method for the evaluation of root canal fillings and its constituents. The qualitative and quantitative correlations between histologic and micro-CT examination of root canal fillings were high. However, it is important to note that micro-CT remains a research tool and cannot be used for human imaging in vivo.
This chapter discusses the principles, applications, imaging attributes, image artifacts, and potential liability of adopting CBCT technology for endodontic procedures. Given this information, the student of endodontics will begin to realize the significant advantages, limitations, and diagnostic and treatment planning capabilities of this radiographic imaging modality.
Principles of Cone Beam Computed Tomography
Three important parameters of cone beam imaging are described in the following sections:
- •
Voxel size
- •
Field of view (FOV)
- •
Slice thickness/measurement accuracy
Voxels and Voxel Sizes
Voxels are cuboidal elements that constitute a 3D volume, unlike pixels, which are 2D. Data are acquired and represented in three dimensions using voxels. Unlike with medical computed tomography (MDCT), cone beam units acquire x-ray information using low kV and low mA exposure parameters in a single pass from 180 to 360 degrees of rotation around the anatomy of interest. Medical scanners use higher voltages of 120 kV or more and current of about 400 mA. Several units used in maxillofacial imaging use significantly lower exposure parameters ( Figs. 2-5 to 2-7 ). The x-ray dose for all cone beam units is significantly lower than the dose received from a MDCT unit. Image attributes are also different in that volumes are reconstructed from isotropic voxels; that is, the images are constructed from volumetric detector elements that are cubical in nature and have the same dimensions of length, width, and depth. These voxel sizes can be as small as 0.076 to 0.6 mm. By comparison, MDCT slice data are 0.5 mm to 1 cm thick. Fig. 2-8 illustrates the difference between a pixel and a voxel, the difference between an anisotropic pixel of MDCT and an isotropic pixel (voxel) of CBCT, and how the pixel data are acquired from both modalities.




The patient is positioned on a gantry in an MDCT unit, and images are acquired multiple slices at a time, which prolongs the acquisition time. The number of slices acquired is a direct function of the sensor array configuration. Spiral CT uses continuous translator motion of the gantry as images are acquired, thus shortening the acquisition time. This results in significantly higher absorbed x-ray doses for the patient. A typical CBCT examination would expose the patient to only about 20 to 500 µ Sv in a single study, whereas a typical medical examination of the head would approach 2100 µ Sv because the image data are gathered one section at a time. Therefore, soft tissue imaging is better with MDCT because the signal intensity is higher. However, this is not a requirement for dental diagnostic tasks because hard tissue visualization is more important. Consequently, CBCT data have a much higher resolution than MDCT data for hard tissue visualization because of the smaller voxel sizes that medical-grade scanners are incapable of achieving at a significantly lower dose. Increased noise is observed as a result of volumetric acquisition, but the SNR is maintained at a desirable level that facilitates adequate diagnosis based on hard tissue signals.
Field of View
The field of view (FOV) ( Figs. 2-9 and 2-10 ) ranges from as small as a portion of a dental arch to an area as large as the entire head. The selection of the FOV depends on several factors. Among the most important are the following:
- •
Diagnostic task
- •
Type of patient
- •
Spatial resolution requirements


Diagnostic Task
The diagnostic task is the single most important determinant of the FOV in any imaging study. Based on the outcome of the clinical assessment, history, and evaluation of previous and other available imaging studies, a segment of the jaw or a larger area may need to be imaged using an appropriate FOV. If systemic conditions or generalized disorders are suspected, a larger FOV is sometimes required. For most endodontic purposes, a limited FOV can be used, if no signs or symptoms of systemic conditions are reported or noted. Under no circumstances should a screening study be done using a large FOV in the absence of signs and symptoms justifying the procedure. Several multifunctional cone beam units are available that allow the clinician to acquire several image types. Image quality has a direct impact on the diagnostic outcome; therefore, the choice of an FOV should be made carefully. Figure 2-11 illustrates the advantages of using multiple image types for an endodontic case.

Additional benefits of CBCT imaging software include allowing the clinician to format the volume to generate an image that looks like a panoramic radiograph. Conventional panoramic machines, although not commonly used by endodontists, use the focal trough, or zone of sharpness, to position patients so as to minimize distortion along multiple axes. All inherent problems associated with panoramic imaging, including distortion, magnification, blurring, ghost shadows, and other artifacts, can be expected on the resulting image if patient positioning is not accurate. With CBCT, such artifacts are not generated, resulting in a distortion-free panoramic reconstruction ( Fig. 2-12 ). However, it must be noted that CBCTs should not be generated in patients requiring a panoramic radiograph alone, because of dose concerns.

Newer hybrid units, such as the CS 9300 3D Extraoral Imaging System (Carestream Dental, Atlanta, Georgia), have a wide range of FOV choices for a variety of diagnostic tasks, in addition to a conventional panoramic imaging option ( Fig. 2-13 ). The CS 9000 unit offers the lowest voxel size of 76 microns, whereas the CS 9300 can resolve down to 90 microns, with a range extending to 500 microns for larger FOV studies. Likewise, the Morita 3D Accuitomo 80 (J. Morita USA, Irvine, California) generates isotropic voxels of 80 microns. Although not necessary for use in every case, this technology, when appropriate, improves visualization and ultimately leads to better care in select situations. A record of exposure and doses must be maintained for each patient.

Type of Patient
Patient size and thus the amount of regional anatomy captured in the study also help determine the FOV. The smallest possible FOV must be chosen for the task at hand. Just because a clinician owns a cone beam machine does not mean that every patient should be exposed to a cone beam study. If previous studies are available, they need to be evaluated first in a recall patient. Use of imaging in children must be minimized. Cone beam machines with smaller FOVs can somewhat limit the radiation dose to critical organs and tissues of the head and neck in these cases.
Spatial Resolution Requirements
All endodontic imaging procedures require high spatial resolution. Assessment of canal structure, canal length, and lesions of endodontic origin (LEOs) showing apical change, in addition to an understanding of possible revision cases, are important tasks requiring minute detail. If CBCT is used, the data acquisition should be performed at the smallest voxel size: the smaller the voxel size, the higher the spatial resolution. Many of the larger stand-alone cone beam machines, such as the i-CAT (Imaging Sciences International, Irvine, California), default to a 0.4 mm voxel size. This voxel size is inadequate for high spatial detail. However, these units often have a voxel size selection option that allows smaller voxel sizes to be used during the image acquisition. The absolute maximum voxel size for endodontic imaging should be 0.2 mm. Units typically use voxel sizes of 0.076 to 0.16 mm for their native image capture ( Fig. 2-14 ).

Imaging Tasks Improved or Simplified by Cone Beam Volumetric Computed Tomography
The Executive Opinion of the American Academy of Oral and Maxillofacial Radiology and, later, the position paper on the use of CBCT in endodontics jointly developed by the American Association of Endodontists (AAE) and the American Academy of Oral and Maxillofacial Radiology (AAOMR), list indications for potential use in selected cases, including evaluation of the anatomy and complex morphology, differential diagnosis of complex pathoses with certain qualifiers, intraoperative or postoperative assessment of endodontic treatment, dentoalveolar trauma, resorption, presurgical case planning, and dental implant case planning. Use of CBCT must be determined on a case-by-case basis only. These indications do not in any way mandate the use of CBCT for every case that falls into one of the preceding categories. For endodontic treatment and assessments, there are at least five primary imaging tasks in which CBCT scans have a distinct advantage over traditional 2D radiographs. These tasks include evaluation of the following factors:
- 1.
Differential diagnosis
- a.
Lesions of endodontic origin
- b.
Lesions of nonendodontic origin
- c.
Diagnosis of endodontic treatment failures
- d.
Vertical root fractures
- a.
- 2.
Evaluation of anatomy and complex morphology
- a.
Anomalies
- b.
Root canal system morphology
- a.
- 3.
Intraoperative or postoperative assessment of endodontic treatment complications
- a.
Overextended root canal obturation material
- b.
Separated endodontic instruments
- c.
Calcified canal identification
- d.
Localization of perforation
- a.
- 4.
Dentoalveolar trauma
- 5.
Internal and external root resorption
- 6.
Presurgical case planning
- 7.
Dental implant case planning
- 8.
Assessment of endodontic treatment outcomes
Differential Diagnosis
Lesions of Endodontic Origin
Clinical endodontic diagnosis relies on subjective and objective information collected during patient examinations. Diagnosis of the pulpal status of the teeth can sometimes be challenging if adequate radiographic information is unavailable. It is fundamental to understand that lesions of endodontic origin arise secondary to pulpal breakdown products and form adjacent to canal portals of exit. These radiolucent lesions, formed as a result of loss of bone mineralization, can and do form three dimensionally anywhere along the root surface anatomy. A 30% to 40% mineral content loss is needed for these lesions to be visualized on conventional radiographs. Furthermore, the thickness of the cortical plate covering the lesion may significantly affect the radiographic appearance of the lesion on a conventional image. In a comparative investigation of the use of CBCT and periapical (PA) radiography in the evaluation of the periodontal ligament (PDL), Pope et al. showed that necrotic teeth examined with CBCT had widened PDLs, but healthy, vital teeth showed significant variation. They called for further investigation to determine whether health and disease can be appropriately judged by the use of CBCT in epidemiologic investigations.
Digital subtraction radiography (DSR) has been observed to increase diagnostic capability; observers identified incipient periapical lesions in more than 70% of the cases. Before the advent of CBCT, clinicians were unable to routinely visualize the presence, specific location, and extensiveness of periapical bone loss using conventional radiography. This was especially true in areas with superimposition of anatomic structures. Visual obstruction from anatomic features, such as buccal bone and the malar process over the apices of maxillary roots, simply “disappears” when the examiner can scroll through the slices of the bone from facial to palatal in 0.1 mm sections while also changing axial orientations. CBCT showed significantly higher rates of detection of periapical lesions in maxillary molars and premolars compared to PA radiography.
Lesions of Nonendodontic Origin
Differential diagnosis of periapical pathology is crucial to endodontic treatment planning. Substantial evidence in the literature points to a significant chance that lesions of the tooth-supporting structures are nonendodontic in origin, such as periapical cemento-osseous dysplasia; central giant cell granulomas; simple bone cysts; odontogenic cysts, tumors, or malignancies; and neuropathic pain ( Fig. 2-15 ). *

* References .
Neuropathic orofacial pain or atypical odontalgia (AO), also known as chronic continuous dentoalveolar pain (CCDAP) and persistent idiopathic facial pain ( PDAP), is related to a tooth, teeth, or pain at an extraction site where no clinical or radiographic pathosis is evident. Two systematic reviews of AO showed the incidence of persistent pain of more than 6 months’ duration after nonsurgical and surgical endodontic treatment, excluding local inflammatory causes, was 3.4%. The pathophysiology of this pain is uncertain, but it is hypothesized to involve deafferentation of peripheral sensory neurons in predisposed patients. The diagnosis of AO is challenging and depends on the patient history and clinical examination findings, in addition to the absence of radiographic findings. In some cases the symptoms from AP and AO are closely related. Pigg et al. conducted a study of 20 patients with AO. All of the patients had at least one tooth in the region of discomfort that had undergone invasive treatment; 21 of 30 teeth had undergone endodontic treatment. These researchers found that 60% had no periapical lesions, and among those who did, CBCT showed 17% more periapical lesions than conventional radiography. This study demonstrated that CBCT may be a useful supplement to 2D radiography (see Fig. 2-15 ).
The 3D radiographic appearance of a periapical lesion provides additional information about the lesion’s relationship to the tooth and other anatomic structures (e.g., the vascular bundle) and about the aggressiveness of the lesion. This information, along with pulp sensitivity testing, is useful for adequate treatment planning and management of these conditions.
Diagnosis of Endodontic Treatment Failures
Failure of previous endodontic therapy can be attributed to various factors, such as procedural errors, missed canals, or persistent periapical pathosis. Knowledge of the cause of failure is pertinent to the treatment of these cases because it allows the cause to be adequately rectified. With the advent of CBCT, in select cases of retreatment in which the cause of failure is otherwise undetectable, adequate information may be collected to apply to the treatment plan ( Fig. 2-16 ). The technology is most useful in detecting uninstrumented and unfilled canals, extension of the root canal filling, and the presence and extent of periradicular bone loss. The sensitivity of CBCT and PA radiographs for diagnosing strip perforations in root-filled teeth has been shown to be low. Radiopaque filling materials in the root canals of endodontically treated teeth can produce streak artifacts, which can mimic fracture lines or perforations.

Vertical Root Fractures
Vertical root fractures (VRFs) that run along the long axis of a tooth are often difficult to diagnose clinically. The prevalence of VRF in endodontically treated teeth has been reported to range from 8.8% to 13.4%. These fractures typically run in the buccolingual direction and are confined to the roots, making it difficult to visualize the fracture. Visualizing the fracture on a conventional radiograph is possible when the x-ray beam is parallel to the plane of the fracture. Challenges in diagnosis with regard to the extent and exact location of the fracture often lead to unwarranted extraction of teeth. Since the introduction of CBCT to dentistry, various reports of the application of the technology to detect vertical root fractures have been published. The reported sensitivity for detection of VRFs has ranged from 18.8% to 100% ; by comparison, conventional radiographs have a reported sensitivity of approximately 37% ( Fig. 2-17 ). CBCT has been used to visualize VRFs in controlled clinical studies in which clinical diagnosis was difficult. Vertical root fractures were successfully detected at a spatial resolution ranging from 76 to 140 microns. However, only a limited number of units provide such high resolution. A comparison of various CBCT units for the detection of VRFs demonstrated that the units with flat panel detectors (FPDs) were superior to the image intensifier tube/charge-coupled device (IIT/CCD)–based detectors; the smaller FOV and the ability to view axial slices also improved detection of VRFs. Continued improvement of sensor technology, including the use of FPDs, has resulted in enhanced resolution. Voxel dimensions are smaller in these units. Detection of vertical root fractures with thickness ranging from 0.2 to 0.4 mm was found to be more accurate with CBCT than with digital radiography. The presence of root canal filling in the teeth lowers the specificity of CBCT in detecting vertical root fractures ; this has been attributed to the radiopaque material causing streak artifacts that mimic fracture lines.

Evaluation of Anatomy and Complex Morphology
The precise location and visualization of dental anomalies, root morphology, and canal anatomy are vastly improved with CBCT data. Root curvature, additional roots, and anomalies within the canals themselves (e.g., obstructions, narrowing, bifurcation) are made more apparent when all three anatomic planes of section are available for review, especially with the capability of narrowing the slice thickness to as little as 0.076 mm. Visual obstruction from anatomic features such as buccal bone and the malar process over the apices of maxillary roots simply will “disappear” when you can scroll through the slices of the bone from facial to palatal in 0.076 mm sections while also changing axial orientations ( Fig. 2-18 ).

Dental Anomalies
The use of CBCT technology has been reported in the diagnosis and treatment planning of various dental anomalies (e.g., dens invaginatus) that often have complex morphologic presentations. The prevalence of dens invaginatus was as high as 6.8% in the adolescent Swedish population studied. The complex nature of the anomaly presents a diagnostic challenge when conventional radiographs are used. In case reports in which CBCT was used for diagnosis and treatment planning, treatment options included conservative endodontic treatment of the invagination, surgical treatment of the periapical pathology, and complete revascularization of the dens after removal of the invagination ( Fig. 2-19 ).

Root Canal System Morphology
As the adage goes, nature seldom makes a straight line and never makes two of the same. This statement is dramatically illustrated in the evaluation of root canal system morphology. With ever-present unusual and atypical root shapes and numbers, there is sometimes a need to look further than what a clinician can see or imagine with 2D radiography ( Fig. 2-20 ). Variations in root canal morphology have been studied using various in vitro techniques. The results of these studies point to the fact that there is significant variation in the root canal morphology among various ethnic population groups. CBCT has been reported to be comparable to canal staining and clearing techniques for identification of the root canal morphology, and CBCT studies report variation in the root canal morphology among various ethnic groups.

Intraoperative or Postoperative Assessment of Endodontic Treatment Complications
Materials Extending Beyond the Root Canal
CBCT scans provide the opportunity to map endodontic treatment complications through the examination of 3D representations of the teeth and supporting structures in different planes. Few high-level studies related to the effects of endodontic treatment complications have been published in the endodontic literature. However, it is generally recognized that overfilling of the root canal, causing damage to vital structures such as the inferior alveolar neurovascular bundle (IAN) ( Fig. 2-21 ) or the maxillary sinus, can cause significant morbidity.

Endodontic therapy undertaken in close proximity to the IAN should receive special attention because direct trauma, mechanical compression, chemical neurotoxicity, and an increase in temperature greater than 10° C may cause irreversible damage. Scolozzi et al. reported that sensory disturbances can include pain, anesthesia, paresthesia, hypoesthesia, and dysesthesia. The IAN is located in the cribriform bone-lined mandibular canal and courses obliquely through the ramus of the mandible and horizontally through the mandible body to the mental foramen and the incisive foramen. There are many anatomic variations of the IAN, including the anterior loop and bifid mandibular canals. Kovisto et al. used CBCT measurements from 139 patients to show that the apices of the mandibular second molar were closest to the IAN. In females, the mesial root of the second molar was closer than in males, and the distances in all roots measured increased with the age of the patient. There was a high correlation between the measurements from left to right side in the same patient; an average distance of 1.51 to 3.43 mm in adults. Procedures involving the mandibular second molar were most likely to cause nerve damage. Further research is required to clarify the risks and benefits of CBCT when endodontic treatment is contemplated on teeth with a proximal relationship between the IAN and root apices. Porgrel treated 61 patients with involvement of the IAN after root canal therapy during a 7-year period. Eight patients were asymptomatic; 42 patients were seen for mild symptoms or were examined more than 3 months postoperatively, with only 10% experiencing improvement. Five patients underwent surgical treatment before 48 hours elapsed and recovered completely. Six patients underwent surgery between 10 days and 3 months, with four experiencing partial recovery; the remaining two had no improvement.
New imaging technologies, such as high-resolution magnetic resonance imaging (MRI-HR) and magnetic resonance neurography (MRN), promise to improve isolation of the IAN from the neighboring artery and vein contained within the inferior alveolar bony canal. MRN studies have documented the ability to demonstrate nerve continuity and localize extraneural nerve compression before surgical nerve exploration. Postoperative periapical radiographs should be exposed on the day of endodontic treatment completion or a suspected iatrogenic event, and any suspected compromise of the IAN or other vital structures should be evaluated immediately. In all cases in which trauma to the IAN is suspected from periapical or panoramic radiography or by the report of symptoms consistent with nerve injury, exposure of a CBCT image volume should be considered. It is generally accepted that immediate surgical debridement should be attempted to maximize recovery. With the introduction of MRI algorithms for dental diagnostic purposes, it is expected that this imaging modality will be increasingly used in diagnostic and treatment planning. MRI has the capability to demonstrate vascularity to the tooth of interest, in addition to the presence of inflammatory exudates in the apical regions, without exposing the patient to ionizing radiation. Receiver coils are being developed to enhance the image quality of maxillofacial and dental magnetic resonance studies.
The accidental introduction of root canal instruments, irrigating solutions, obturation material, and root tips into the maxillary sinus has been reported. Serious consequences associated with the intrusion of foreign bodies into the maxillary sinus include pain, paresthesia, and aspergillosis, a rare but well-documented complication of endodontic treatment. Guivarc’h et al. reported that the overextension of heavy metal–containing root canal sealers, such as zinc oxide eugenol cement, may promote fungal infection in immunocompromised patients, leading to bone destruction and damage to adjacent structures. This case report described the use of computed tomography to assess the patient before surgery and at 6 months. The use of CBCT as an aid in the localization and retrieval of an extreme overextension of thermoplasticized injectable gutta-percha into the sinus and contiguous soft tissues has been described by Brooks and Kleinman.
Fractured Instruments
Instrument fracture can occur at any stage of endodontic treatment, and in any canal location. The incidence of this complication, reported in clinical studies on a per canal or per tooth basis, ranges from 0.39% to 5.0%. Molars are predominantly affected by instrument fracture, with the highest incidence found in the apical third of mandibular molars.
A systematic review and meta-analysis showed that when endodontic treatment was performed at a high technical standard, instrument fracture did not significantly reduce the prognosis. More specifically, when no initial radiographic periapical lesion was present initially, 92.4% of cases remained healthy; when a periapical lesion was present initially, 80.7% of periapical lesions showed radiographic healing. However, the presence or absence of periradicular lesions on preoperative and postoperative examinations was based on planar radiographic assessments, which calls these findings into question. Other studies showed that the chances of endodontic failure increased if the canal system could not be thoroughly disinfected, if a periradicular periodontitis was present, or if technical standards were compromised. Use of CBCT to triangulate the retained instrument and assess the canal shape, especially in cases in which the operating microscope does not allow direct visualization, can be helpful in formulating a removal strategy. If the fractured instrument is lodged in the lingual aspect of a ribbon-shaped canal, for example, an instrument may be inserted toward the buccal to bypass and remove the imbedded instrument without forcing the fragment further apically. Without the use of CBCT, intracanal instruments can be reliably removed or bypassed in 85.3% of cases if straight-line access is possible; however, reliable removal or bypass is possible in only 47.7% of cases if the instrument is not visible ( Fig. 2-22 ). When a separated instrument is lodged in the apical third of a root canal, the chances of retrieval are the lowest, but the apical terminus may be adequately sealed by treatment of an anastomosing canal, if present. The possibility of instrument removal based on CBCT triangulation has not been published to date.
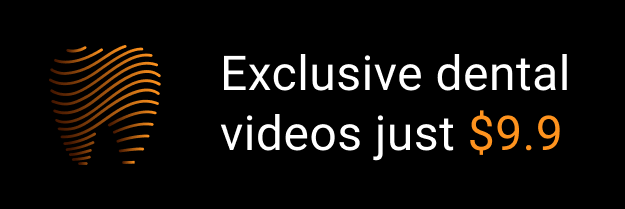