Introduction
Identification of pubertal growth peak is of great importance for the orthopedic treatment of Class II malocclusion. Our previous work demonstrated that vitamin D binding protein (DBP) and serotransferrin (TF) in gingival crevicular fluid (GCF) could be candidate biomarkers of pubertal growth peak. This research aimed to preliminarily validate TF and DBP in subjects with Class I and Class II malocclusion, to compare their diagnostic accuracy, and to construct a statistic model to help the diagnosis of skeletal pubertal peak.
Methods
Sixty-six circumpubertal subjects were recruited, including 32 subjects with Class I malocclusion and 34 subjects with Class II malocclusion. All subjects were divided into prepubertal, pubertal, and postpubertal groups according to their cervical vertebral maturation stages. GCF samples were collected, and the concentration of DBP and TF were detected by enzyme-linked immunosorbent assay.
Results
Percentage of TF in GCF was significantly higher in pubertal than in prepubertal and postpubertal groups, in subjects with Class I and Class II malocclusion, whereas the difference observed in DBP was less significant. The diagnostic accuracy of TF was better than DBP and chronological age. The most optimal thresholds of maxillary and mandibular TF in distinguishing pubertal from nonpubertal subjects were 4.20% and 4.09%, respectively. The combination of TF and age exhibited the best diagnostic accuracy.
Conclusions
TF in GCF could be considered as a potential biomarker of pubertal peak and can assist the diagnosis of skeletal pubertal peak.
Highlights
- •
TF in the GCF was significantly higher in pubertal than in nonpubertal subjects.
- •
The optimal threshold of maxillary TF distinguishing pubertal subjects was 4.20%.
- •
TF combined with chronological age could achieve acceptable diagnostic accuracy.
It is well known that pubertal growth peak plays a vital role in decision making of orthodontic treatment timing, especially for subjects with Class II malocclusion with retrusive mandible. Traditional x-ray methods were usually applied to identify skeletal maturation stage and to determine the most appropriate treatment timing, such as hand wrist method and cervical vertebral maturation method (CVM). However, the hand wrist method has its limitation as patients have to suffer from extra radiation exposure. The CVM stage can be evaluated on a lateral cephalogram, which is a part of routine pretreatment examination. In addition, CVM was proved to be a reliable method to identify the pubertal peak. Reproducibility of CVM assessment was also satisfying in the circumstance that the observer had accepted a professional training course.
However, because of the qualitative nature of the CVM method, researchers have been trying to explore new quantitative indicators as alternatives to predict pubertal growth peak, among which biomarkers in body fluids such as serum , and saliva turn out to be the most attractive. Gingival crevicular fluid (GCF) was considered as an ideal source of biomarkers for its simple and noninvasive collection procedure. , In our previous work, we collected GCF samples from 20 pubertal and 20 postpubertal subjects and applied liquid chromatography-tandem mass spectrometry combined with tandem mass tags labeling to compare the whole GCF proteome between pubertal and postpubertal subjects. Through gene ontology analysis, we found that vitamin D binding protein (DBP) was the most significantly enriched protein ( P <0.0001). Meanwhile, serotransferrin (TF) in terms of “iron ion homeostasis” exhibited a similar function and was reported to be enriched in the serum of pubertal subjects. Therefore, TF and DBP were chosen as candidate GCF biomarkers indicating pubertal peak.
TF was synthesized in the liver and can bind to and transport iron ions in circulation between sites of absorption, storage, and use. Anttila et al reported that serum TF was significantly higher in pubertal boys than in nonpubertal boys. Misaki et al also found that in adolescent boys, the rate of growth in height was significantly correlated with serum TF levels. Malecki et al created an animal model of hypotransferrinemia and found that lack of TF would compromise the mechanical properties of the femur and that TF was important for normal bone mineralization. However, whether TF level in GCF was associated with puberty remains unclear. DBP transports 80%-90% of serum 25-hydroxy vitamin D and 1,25-dihydroxy vitamin D and can promote intestinal calcium absorption and regulate maturation and mineralization of bone. , A previous study showed that 1,25-dihydroxy vitamin D levels peak during puberty to meet the high demand for calcium in this critical phase of bone development. More evidence is still needed to reveal the relationship between DBP level and pubertal growth peak.
Our study aimed to primarily validate TF and DBP as biomarkers for pubertal growth peak in subjects with Class I and Class II malocclusion, to compare their diagnostic accuracy, and to explore their most optimal threshold in distinguishing pubertal subjects from nonpubertal subjects. Ultimately, we combined chronological age with GCF biomarkers to construct a statistic model to help in the diagnosis of pubertal peak.
Material and methods
Subjects were collected from patients who sought treatment at the Department of Orthodontics, Peking University School and Hospital of Stomatology from March 2017 to August 2019. This research was approved by the Biomedical Ethics Committee of Peking University Health Science Center (approval no. PKUSSIRB-201735069) and was performed in accordance with the ethical standard in the 1964 Declaration of Helsinki. Informed consents were received from patients and their parents.
We recruited subjects according to the inclusion criteria as follows: (1) aged between 6 and 18 years; (2) healthy periodontal status; (3) skeletal Class I (0 < ANB < 5°) or Class II (ANB > 5°) malocclusion; (4) no history of orthodontic treatment; (5) no systematic disease; (6) not taken any medication in the past 3 months; and (7) Mongolian.
All subjects took lateral cephalograms as part of routine pretreatment examination to determine the sagittal skeletal relationship and CVM stages. Lateral cephalograms were analyzed using Huazheng System (version 1.2; Peking University School of Stomatology, Beijing, China).
ANB angle (°), overjet (mm), and molar relationship were used to distinguish subjects with Class I and Class II malocclusion. Subjects with ANB > 5°, overjet > 3 mm, and distal molar relationship were included in the Class II malocclusion group, whereas subjects with 0 < ANB < 5°, 0 < overjet < 3 mm, and neutral molar relationship were included in the Class I group.
CVM stage was determined according to the method proposed by Baccetti et al. Subjects at CVM stages 1 and 2 were included in the prepubertal group. In contrast, subjects at CVM stages 3 and 4 were included in the pubertal group, and subjects at CVM stages 5 and 6 were included in the postpubertal group.
The interobserver agreement of the CVM stage was tested by 2 different orthodontists (Y.G. and X.W.). Intraobserver agreement of CVM stage was determined by the same orthodontist (X.W.) initially and 1 week later. Two orthodontists were both blinded to the basic information of studied subjects.
The minimum sample size was calculated on the basis of the formula applying to a 1-way ANOVA 2-tailed test proposed by Chow et al. The type I error probability was set as 0.05, and type II error probability was 0.2. According to the result of our preliminary study, for DBP, the value of μA-μB and σ were 0.11 and 0.17, respectively, and hence the minimal sample size should be 50. For TF, the value of μA-μB and σ were 3.0 and 5.2, respectively, and hence, the minimal sample size should be 63.
Periodontal examination was performed on each subject before GCF collection. Only those with probing depth ≤ 3 mm, bleeding index ≤ 3, and no attachment loss were recruited. All subjects accepted ultrasonic supragingival scaling and oral hygiene instruction 1 week before GCF collection.
GCF sample was collected between 8 am and 10 am . Before collection, the tooth surface was carefully dried, and cotton rolls were used to avoid contamination of saliva. GCF was collected in mesiolabial and distolabial sites of both maxillary and mandibular central incisors. Paper points (no. 30; Tianjin Dayading Medical Treatment Appliance Company, Tianjin, China) were gently inserted into the gingival sulcus until minimal resistance was felt and then were held in situ for 60 seconds. Paper points contaminated by blood would be discarded. After 2 minutes, the collection procedure was repeated using new paper points to ensure adequate volume of GCF samples were collected.
GCF samples from maxillary and mandibular incisors were separated into different 1.5 mL centrifuge tubes (Axygen, Corning Company, Union City, Calif). Each tube contained 8 paper points. The tips of paper points were cut from where they were visibly wetted by GCF and were incubated in 50 μL phosphate buffered solution. After vortexed for 1 minute, GCF samples were centrifuged at 5000 rpm for 10 minutes at 4°C. The supernatant was collected. The same procedure was repeated 3 times, and the elution solution was pooled and stored at −80°C for further analysis.
A bicinchoninic acid kit was used to determine the total protein concentration of GCF samples (Beyotime Biotechnology, Beijing, China). Standard samples were prepared according to the instruction of the manufacturer. An El×808 spectrophotometer (BioTek, Colchester, Vt) was used to detect the optical densities and calculate the total protein concentration.
Commercially available enzyme-linked immunosorbent assay kits of human DBP and TF (Qisong Company, Beijing, China) were applied to determine the concentration of DBP and TF in each GCF sample according to the instruction of the manufacturer. Each sample had 3 replicates, and the mean value was calculated for further statistical analysis.
To further evaluate the diagnostic accuracy of TF and DBP in distinguishing pubertal from nonpubertal subjects, all samples were regrouped. Subjects at CVM stages 1-2 or 5-6 were included in the nonpubertal group, whereas the rest were included in the pubertal group. Thus, the dependent variable was transformed into a binary variable pubertal peak , for which at peak or not were defined as 1 and 0 , respectively. Independent variables, including GCF biomarkers and chronological age, were continuous variables. Hence, logistic regression (LR) model analysis was performed to quantificationally describe the relationship between pubertal peak and its influencing factors (version 3.6.1; R Foundation for Statistical Computing, Vienna, Austria). Through the LR model, the predictive probability of subjects being at pubertal peak could be calculated. Backward stepwise method was used to screen for significant independent variables. The basic form of the LR model was as follows:
Predictive probability=eβ0+β1×1+β2×2+…1+eβ0+β1×1+β2×2+…
In our study, there were 5 potential influencing factors that could have an impact on pubertal peak , including TF, DBP, chronological age, sex, and skeletal type. Specifically, chronological age exhibited parabolic distribution relative to pubertal peak and entered the model in the form of (β 0 + β 1 x 2 + β 2 x), whereas the sex and skeletal type entered as interactive variables with chronological age. To separately evaluate and compare their diagnostic accuracy, LR models were constructed using different combinations of independent variables. They were listed as follows:
- (1)
Model 1: Maxillary TF (Max-TF).
- (2)
Model 2: Mandibular TF (Md-TF).
- (3)
Model 3: Maxillary DBP (Max-DBP).
- (4)
Model 4: Mandibular DBP (Md-DBP).
- (5)
Model 5: Chronological age.
- (6)
Model 6: Comprehensive model including Max-TF, Md-TF, Max-DBP, Md-DBP, age, sex, and skeletal type.
Receiver operator characteristic (ROC) curve were used to evaluate and compare the diagnostic accuracy of 6 models mentioned above. For each model, the true positive rate (TPR) and false positive rate (FPR) of each candidate threshold were calculated. ROC curves were first calculated using back substitution method, which was also applied in some of the previous studies. , However, this method used the same samples for training models and verification, which might exaggerate the final result. Therefore, we further developed a 10-fold cross-validation algorithm using R software to test the diagnostic accuracy of GCF biomarkers. All samples were randomly divided into the training group and verification group, and the regression model learned from the training group was used to predict the verification group. This procedure were repeated 10 times until all samples had been predicted. ROC curves by 10-fold cross-validation method were drawn by R software as well.
Through LR models mentioned earlier, the predictive probability of a subject being at pubertal peak could be calculated, and an optimal threshold was needed to classify this subject into the pubertal or nonpubertal group. Youden index was defined as Sensitivity + Specificity-1 and was calculated on the basis of the ROC curve to determine the most optimal threshold. When the Youden index reached the maximum, the corresponding predictive probability could be used to reversely calculate the value of GCF biomarkers through the formula of LR models, and this value of GCF biomarkers was considered as the most optimal threshold in distinguishing pubertal from nonpubertal subjects.
Statistical analysis
To reduce the error caused by different GCF volume in each sampling procedure, we normalized the concentration of DBP and TF by total protein concentration, which was calculated by dividing DBP or TF concentration by GCF total protein concentration, and the results were presented as percentage of DBP or TF in GCF total protein.
Kappa values were used to evaluate the interobserver and intraobserver agreement of CVM stages. The normality of all variables was tested by the Shapiro-Wilk test. If the variables were normally distributed, independent sample t tests (for 2 independent samples) and 1-way ANOVA (for 3 independent samples) were used to analyze the difference between groups. If variables were not normally distributed, then they were analyzed using the Kruskal-Wallis H test (for 2 independent samples) and Mann-Whitney U test (for 3 independent samples).
Differences with P <0.05 were considered statistically significant. All statistical analysis was accomplished using SPSS (version 19.0; IBM, Armonk, NY) and R software. All figures were created using GraphPad Prism 5 (version 5.01; GraphPad Software, San Diego, Calif) and R software.
Results
A total of 66 patients were recruited, including 32 subjects with Class I malocclusion (mean age, 11.8 ± 2.4 years) and 34 subjects with Class II malocclusion (mean age, 12.2 ± 2.4 years). Detailed information was shown in Table I and Supplementary Figure . Kappa values of CVM stages for interobserver and intraobserver reliability are 0.84 and 0.96, respectively.
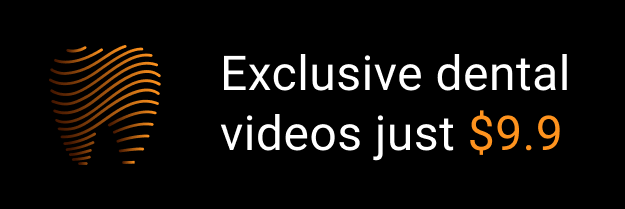