Abstract
Objectives
Methods used to measure and predict clinical biological responses to dental materials remain controversial, confusing, and to some extent, unsuccessful. The current paper reviews significant issues surrounding how we assess the biological safety of materials, with a historical summary and critical look at the biocompatibility literature. The review frames these issues from a U.S. perspective to some degree, but emphasizes their global nature and universal importance.
Methods
The PubMed database and information from the U.S. Food and Drug Administration, International Standards Organization, and American National Standards Institute were searched for prominent literature addressing the definition of biocompatibility, types of biological tests employed, regulatory and standardization issues, and how biological tests are used together to establish the biological safety of materials. The search encompassed articles published in English from approximately 1965–2011. The review does not comprehensively review the literature, but highlights significant issues that confront the field.
Results
Years ago, tests for biological safety sought to establish material inertness as the measure of safety, a criterion that is now deemed naive; the definition of biocompatibility has broadened along with the roles for materials in patient oral health care. Controversies persist about how in vitro or animal tests should be used to evaluate the biological safety of materials for clinical use. Controlled clinical trials remain the single best measure of the clinical response to materials, but even these tests have significant limitations and are less useful to identify mechanisms that shape material performance. Practice-based research networks and practitioner databases are emerging as important supplements to controlled clinical trials, but their final utility remains to be determined.
Significance
Today we ask materials to play increasingly sophisticated structural and therapeutic roles in patient treatment. To accommodate these roles, strategies to assess, predict, and monitor material safety need to evolve. This evolution will be driven not only by researchers and manufacturers, but also by patients and practitioners, who want to use novel materials in new ways to treat oral disease.
1
Introduction
We have spent much effort and money to design tests that predict the clinical biocompatibility of dental materials. Yet even today, the fundamental problem with these tests is that they do not work as well and cannot be as broadly applied as we would like. For several decades, the U.S. Food and Drug Administration, other international governing bodies, researchers in the biocompatibility field, and standards organizations have subscribed to a stepwise paradigm of in vitro , animal, and usage (clinical) tests to predict the clinical biological performance of new materials ( Fig. 1 ) . Originally proposed in 1970 by Autian , this paradigm was adopted to ensure the safe introduction of new biomaterials for patient care while accommodating the need for an efficient, ethically sound, and financially viable assessment process. The tests employed within the paradigm each have strengths and weaknesses (see Section 3 ), but a key tenet of the stepwise strategy has been that each level of test will eliminate unsuitable materials from further testing, hence the pyramidal shape in Fig. 1 . In this manner, high-risk materials should be ‘screened out’ early in the process using faster, less expensive tests, thereby saving time, money, and potential pain and suffering of animals and humans.

In spite of the potential utility and advantages of the stepwise paradigm of Fig. 1 , evidence suggests that it does not work well at all. Early studies demonstrated unequivocally that in vitro , animal, and usage tests did not function as intended within the stepwise paradigm using dental materials relevant to that time . Silicate cements, which tested favorably in in vitro tests , were consistently irritating to the pulp in usage tests. Conversely, zinc-oxide eugenol cement, which was uniformly cytotoxic in vitro , performed favorably in usage tests and via clinical experience. These data spurred others to modify animal or especially in vitro tests to be more clinically relevant and therefore predictive. For over 30 years, the quest for better in vitro tests has embraced the philosophy espoused by Langeland in 1978: if we knew enough and developed better in vitro tests, the stepwise paradigm could work .
We have made progress. We have modified both in vitro and animal test conditions to better mimic clinical use. Early efforts explored different types of in vitro or animal tests . The concept of the barrier test was developed and refined to include dentin barriers . Strategies for aging materials have been applied to in vitro tests . Subcellular mechanisms , inflammatory responses , mutagenic responses , oxidative stress and lower, more clinically relevant concentrations of released chemicals have been investigated . More relevant cell lines for testing dental materials have been developed . And there are many other areas of progress far too numerous to reiterate here. Yet in spite of our progress, the fundamental problem remains: we have little evidence that in vitro or animal tests can reliably predict which materials will be successful in clinical use. The stepwise paradigm introduced over 30 years ago seems substantively no more viable today than it was then.
So how are we to assess and predict the biological response to a new material in a reliable, economically viable, and ethical way? What tools do we have? What new tools do we need? All would agree that we cannot regress to the period when our first use of a material was to try it in a patient. The goals of the current review are to first present concepts that are central to biocompatibility assessment, then to critically discuss the types of tests we currently use to measure biocompatibility. Finally, an alternative paradigm will be presented on how we might progress in our 30+ year quest to adequately assess the biological risks and benefits of a new dental material for clinical use.
2
Principles and basic ideas about biological responses to materials
2.1
A definition for biocompatibility
The most-cited definition for biocompatibility was proposed in 1987 by Williams :
“ ability of a material to perform with an appropriate host response in a specific situation .”
Although this definition seems vague and unhelpful at first glance, it represented a quantum leap forward at the time of its introduction. Prior to this definition, the prevailing view was that successful materials played largely inert roles the body. A long list of ‘non-properties’ had evolved for ‘successful’ biomaterials: non-toxic, non-immunogenic, non-thrombogenic, non-carcinogenic, and so forth . The above definition required that materials not only provide some function, but also recognized that the interface created by introduction of the material will elicit a biological response. Thus, the idea that the material could be truly inert was essentially rejected with the adoption of this definition. Given today’s level of understanding of our bodies as sophisticated, complex biological environments, the idea that one could place a foreign material without some sort of response seems naive.
Recognition of an active interface between biomaterials and biological systems led to several important basic ideas about biocompatibility. These ideas persist today and comprise the core ‘dogma’ of biocompatibility assessment. The first idea is that the interactions at the material–tissue interface occur for both ; the material elicits a response from the body and the body elicits a response from the material. All materials will be changed at some level by their introduction into a biological environment—either via corrosion, chemical modification, deposition of substance, degradation, or other mechanism. This exchange of responses leads to a second idea: that the material–tissue interface is dynamic . As the material and biological tissue are modified by each other, the changes themselves may trigger other changes. Thus, the interface is not static, but is changing over its lifetime. Furthermore, because we are always changing—we age, develop systemic or local diseases, adopt new activities, eat differently, etc.—any equilibrium established at a material–tissue interface is subject to perturbation. A third idea is that reactions at the material–tissue interface are a function of the tissue where the interface is created . For example, implantation of a material next to dentin creates a different interface than one created in bone or soft tissue. Consequently the effect of the tissue on the material and vice versa will be fundamentally different in each environment. Therefore, favorable material-biological responses in one environment do not assure the same in other environments. Put simply, a material that functions well to restore tooth structure lost through caries may not be a good endosseous implant. And a material that serves well as an endosseous implant may not serve well as a partial denture framework or casting.
A fourth idea about biological–tissue interfaces recognizes the nearly obvious, but often forgotten fact that the materials we use do not belong there . Biomaterials are foreign bodies, and biological responses to these materials are characterized by foreign body responses . Most evidence supports the concept that materials promote nonspecific protein absorption, which then triggers formation of foreign body giant cells from monocytes and macrophages that mediate a collagenous fibrous a-vascular barrier between the material and body . Avoiding or limiting the foreign body response has been a major goal of material development in the past decade .
Finally, the most recent idea about biocompatibility is that it is possible to customize interactions at the material–tissue interface . As we ask materials to play more sophisticated, longer-term roles in tissues, we seek to customize and optimize the material–tissue interface to assure the best long-term clinical outcomes. We may modify the surface of a material to limit nonspecific protein absorption, add peptide sequences to encourage native protein or cell interactions, or provide a three-dimensional structure to encourage matrix formation. We may place a material that degrades by design over time, but not before it directs tissue responses via embedded cells, proteins or drugs. To accommodate the bioactive dimension of materials described above, Williams recently (2008) updated his original definition of biocompatibility :
“ ability of a biomaterial to perform its desired function with respect to a medical therapy, without eliciting any undesirable local or systemic effects in the recipient or beneficiary of that therapy, but generating the most appropriate beneficial cellular or tissue response to that specific situation, and optimising the clinically relevant performance of that therapy. ”
2.2
Drugs vs. devices
The U.S. Food and Drug Administration (FDA) recognizes several broad types of substances that are used to promote human health; the two most common of these are drugs and devices. Most dental biomaterials are classified as devices, including filling materials, diagnostic aids, cements, bonding agents, and implants. The FDA defines a device as :
“ an instrument, apparatus, implement, machine, contrivance, implant, in vitro reagent, or other similar or related article, including a component part, or accessory which is:
(1) recognized in the official National Formulary, or the United States Pharmacopoeia, or any supplement to them, (2) intended for use in the diagnosis of disease or other conditions, or in the cure, mitigation, treatment, or prevention of disease, in man or other animals, or intended to affect the structure or any function of the body of man or other animals, and (3) which does not achieve any of it’s primary intended purposes through chemical action within or on the body of man or other animals and which is not dependent upon being metabolized for the achievement of any of its primary intended purposes. ”
The European Union (EU) directive 93/42 (MDD) defines a medical device as a material that :
“ achieves its principal way of action not by pharmacological, immunological, or metabolic means, but which may be assisted in its function by such means. ”
Put simply, drugs are distinct from devices because drugs use chemical actions for their primary effect whereas devices do not. Drugs and devices are regulated by different branches of regulatory agencies, and approval of drugs requires that they show both safety and efficacy for their intended chemical action. Devices, on the other hand, must show only safety in their intended use to cure, treat, or prevent human disease. According to FDA regulations, if the device emits radiation of any type (including blue light), it must also comply with section 531 of the Federal Food Drug & Cosmetic Act .
The FDA classifies devices as Class I, II, or III types, with Class III reserved for materials that pose the greatest risks to human health ; classification is based on both the intended use and indications for use . The complexity of the approval process and regulations post-approval depend on classification, Class III materials being scrutinized the most both pre- and post-approval. Class III devices require a rigorous and expensive pre-market approval (PMA) process to gain FDA approval. The Class I or II designations make it possible for companies to use the so-called 510(k) process to introduce ‘new’ materials relatively rapidly onto the market by claiming they are substantially equivalent to a material already on the market (see Section 3.5 ). If Class I and II devices are ‘exempt’, then even the 510(k) requirement may be waived .
Most dental devices are Class I or II, including casting alloys, amalgam (with special consideration), implants, composites, cements, and bonding agents ( Table 1 ). The classification of devices holds importance for manufacturers because it directly determines the costs and time required to get a material to market. Post-market surveillance also depends on the classification. Thus, the ability to introduce new materials in dental practice is significantly facilitated because of their classification as devices, and their classification as Type I or Type II devices.
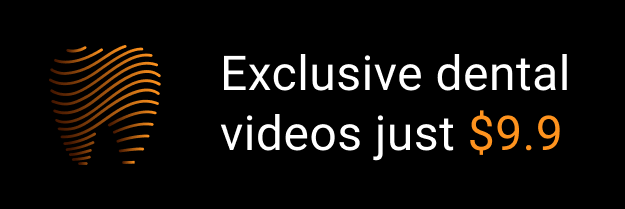