Abstract
Objectives
This overview is intended to highlight connections between monomer structure and the development of highly crosslinked photopolymer networks including the conversion dependent properties of shrinkage, modulus and stress.
Methods
A review is provided that combines the polymer science and dental materials literature along with examples of relevant experimental results, which include measurements of reaction kinetics, photorheology as well as polymerization shrinkage and stress.
Results
While new monomers are continually under development for dental materials applications, mixtures of dimethacrylate monomers persist as the most common form of dental resins used on composite restorative materials. Monomer viscosity and reaction potential is derived from molecular structure and by employing real-time near-infrared spectroscopic techniques, the development of macromolecular networks is linked to the evolution of polymerization shrinkage (measured by linometer), modulus (measured by photorheometer), and stress (measured by tensometer). Relationships between the respective polymer properties are examined.
Significance
Through a better understanding of the polymer network formation and property development processes using conventional dimethacrylate monomer formulations, the rational design of improved materials is facilitated with the ultimate goal of achieving dental polymers that deliver enhanced clinical outcomes.
1
Introduction
Polymer network formation is highly dependent on the structure of the monomer or comonomers used as well as the polymerization conditions employed. In direct dental restorative applications, the monomers are typically selected to yield densely crosslinked, glassy polymer networks that provide high modulus and strength, resistance to swelling and staining, and when used with inorganic fillers, composite wear rates that are comparable to that of sound enamel. Since direct placement restorations require that polymerization processes be conducted at near-ambient conditions, the use of pressure and heat, which can be applied in laboratory-processed indirect materials, leave photopolymerization as the preferred curing mode. Photopolymerization provides the distinct advantage of one-part materials that can be cured on demand with the reaction rate readily manipulated through the combination of the photoinitiator choice and concentration, as well as the irradiance and wavelength range introduced by the light source. The ability to spatially localize the irradiation and alter the photo flux over a very wide range offers both physical and chemical approaches to control polymer network development including the evolution of properties and the final properties achieved. Visible light activation over the range of 400–500 nm, primarily based on camphorquinone and tertiary amine photoinitiation , remains the convention used in dentistry to avoid the potential for biological damage to both patients and practitioners. The shorter UV wavelengths compared with visible light also limit the light penetration depth, particularly in highly scattering materials such as composites . However, the lower energy levels of the photons associated with the longer visible light wavelengths also mean that direct photodecomposition of the initiator to produce active radicals is not typically energetically allowed. This means that two- and three-component photoinitiating systems are used with visible light polymerizations that are reliant on reductive or in some cases, oxidative transitions of the photosensitizer component to generate the initiating radicals . Acyl and bisacyl phosphine oxide initiators can be utilized as single component visible light alpha-cleavage (or Norrish type I) initiators at wavelengths generally below 450 nm. While particular aspects of selected photoinitiators or irradiation conditions will be covered in the subsequent discussion here, the visible light photopolymerization process as applied to dental materials, which includes a variety of irradiation modes , has been well covered in recent reviews , and is not a primary topic in this report. This paper is intended to provide a general overview of dimethacrylate photopolymer network development and describe how monomer choice and curing conditions can affect the network structure and polymer properties. The aim is to integrate literature in the field of basic polymer science with relevant recent dental materials studies while augmenting this with a number of applicable examples from the author’s laboratory.
In free radically induced dimethacrylate photopolymerizations, which represent the large majority of all commercial resins used in dental composite restorative materials , there are several distinct stages to the polymerization process as the reaction progresses from a liquid pre-gel regime to a rubbery gelled phase and finally reaches a glassy state. Under ambient conditions, the limiting conversion due to vitrification of polymeric networks in dental resins based on commonly used dimethacrylate monomers can range from less than 50% to more 80% . The initial liquid monomeric state of the resin is based on mixtures of comonomers selected to fulfill a variety of material property demands prior to polymerization as well as in the final polymer. Monomers are typically selected on the basis of their viscosity, refractive index, hydrophilic/hydrophobic character, reactivity, and potential contribution to the eventual crosslink density of the polymer network. Structure and included functionality (other than the reactive methacrylate groups) of the monomers determines these properties, which are largely but not exclusively, additive in nature when comonomer mixtures are formulated. In particular, polymerization reaction rates can be synergistically enhanced by appropriate combinations of monomers and certain monomer structures contribute unusually high rates of polymerization .
2
Monomeric viscosity
The size and shape of a liquid monomer molecule as well as its potential for intermolecular interactions determines bulk viscous flow behavior. In liquids, the individual molecules are mutually attracted through van der Waal’s forces that range from very weak to relatively strong interactions. Short chain branching within the molecular structure can reduce viscosity for equivalent molecular weight monomers since branching enhances intermolecular interactions that do not directly affect viscosity. Dipole–dipole interactions, of which hydrogen bonding is an important example, represent the strongest interactions between neutral molecules. The strength of the intramolecular interactions is important since these effectively make small molecules behave as larger structures. Unlike many photocured industrial coating materials, dental resins tend to make relatively little use of high molecular weight reactive oligomers, such as the one developed for Kalore (GC America). Small molecule monomers such as triethylene glycol dimethacrylate (TEGDMA) or hexanediol dimethacrylate (HDDMA), that also lack hydrogen bond donor functionality present very low viscosities. On the other hand, BisGMA {2,2-bis[p-(2′-hydroxy-3′-methacryloxypropoxy)phenylene]propane}, which is an interesting example of a monomer molecule designed specifically for use in dental materials (and now is found in a diverse range of high-performance, polymer-based material applications), exhibits dramatically high viscosities. The range of viscosity of BisGMA (approximately 700 to more than 1300 Pa s) is based on small differences in the degree of oligomerization that occurs during the synthetic procedure. The extreme room temperature viscosity in BisGMA is derived from the relatively strong hydrogen bonding between hydroxyl groups, which is exclusively intermolecular in this monomer due to the rigid aromatic core structure . The weaker, but still significant contribution of π–π aromatic ring stacking interactions in BisGMA is demonstrated by comparison of its viscosity with those of ethoxylated bisphenol A dimethacrylate (BisEMA; 3 Pa s) and TEGDMA (0.05 Pa s) . Another perspective on this comes from the comparison of BisGMA with its essentially half-sized monovinyl analog prepared by the reaction of 4-tert-butylphenyl glycidyl ether with methacrylic acid. With a single hydroxyl group, the analog does not engage in the extended hydrogen bonding interactions and it has a viscosity several orders of magnitude lower than that of BisGMA .
Hydrogen bonding interactions associated with urethane functionality, such as is found in urethane dimethacrylate (UDMA; 1,6-bis(methacryloxy-2-ethoxycarbonylamino)-2,4,4-trimethylhexane) are significantly weaker than those based on hydroxyl groups as shown by the conversion of BisGMA to a series of urethane-containing dimethacrylate monomers with greatly reduced viscosities ( Fig. 1 ) . That prior study also highlighted another interesting attribute of BisGMA. In spite of the much lower degree of conversion achieved during the homopolymerization of BisGMA relative to the lower viscosity (higher molecular mobility) urethane derivatives, the mechanical strength was greatest for the BisGMA polymer as a result of the strong hydrogen bonding interactions that serve as non-covalent physical crosslinks in the network. The hydrogen bond strength of BisGMA-containing resins was also found to be unaffected by polymerization whereas the interactions involving urethane groups in UDMA-based polymers were modestly reduced compared to the monomeric state . Hydrogen bonding is transient in nature with cycling rates comparable to small molecule diffusion rates and well in excess of molecular diffusion processes in polymeric systems. Therefore, hydrogen bonding can serve a significant role in the reinforcement of polymeric network structures, especially in processes involving high strain rates. The practical approach of using reactive diluent comonomers to adjust a formulated resin viscosity to a specific target is readily predictable, although the viscosity of the mixture varies in logarithmic fashion with the composition rather than as a simple arithmetic mean of the individual constituent viscosities ( Fig. 2 ) . A similar logarithmic dependence is found between temperature and viscosity of a resin . The choice of monomers also alters the handling properties of a resultant composite paste. Obviously, the size, shape and composition of the filler or mixed fillers used, as well as the surface treatment applied to the fillers will also affect the paste rheology, but differences in monomer composition in terms of viscosity and functionality are factors in composite paste cohesion and how the paste adheres or releases from various surfaces at different shear rates. Studies have recently begun to focus on how subtle compositional variations significantly influence composite handling properties .
2
Monomeric viscosity
The size and shape of a liquid monomer molecule as well as its potential for intermolecular interactions determines bulk viscous flow behavior. In liquids, the individual molecules are mutually attracted through van der Waal’s forces that range from very weak to relatively strong interactions. Short chain branching within the molecular structure can reduce viscosity for equivalent molecular weight monomers since branching enhances intermolecular interactions that do not directly affect viscosity. Dipole–dipole interactions, of which hydrogen bonding is an important example, represent the strongest interactions between neutral molecules. The strength of the intramolecular interactions is important since these effectively make small molecules behave as larger structures. Unlike many photocured industrial coating materials, dental resins tend to make relatively little use of high molecular weight reactive oligomers, such as the one developed for Kalore (GC America). Small molecule monomers such as triethylene glycol dimethacrylate (TEGDMA) or hexanediol dimethacrylate (HDDMA), that also lack hydrogen bond donor functionality present very low viscosities. On the other hand, BisGMA {2,2-bis[p-(2′-hydroxy-3′-methacryloxypropoxy)phenylene]propane}, which is an interesting example of a monomer molecule designed specifically for use in dental materials (and now is found in a diverse range of high-performance, polymer-based material applications), exhibits dramatically high viscosities. The range of viscosity of BisGMA (approximately 700 to more than 1300 Pa s) is based on small differences in the degree of oligomerization that occurs during the synthetic procedure. The extreme room temperature viscosity in BisGMA is derived from the relatively strong hydrogen bonding between hydroxyl groups, which is exclusively intermolecular in this monomer due to the rigid aromatic core structure . The weaker, but still significant contribution of π–π aromatic ring stacking interactions in BisGMA is demonstrated by comparison of its viscosity with those of ethoxylated bisphenol A dimethacrylate (BisEMA; 3 Pa s) and TEGDMA (0.05 Pa s) . Another perspective on this comes from the comparison of BisGMA with its essentially half-sized monovinyl analog prepared by the reaction of 4-tert-butylphenyl glycidyl ether with methacrylic acid. With a single hydroxyl group, the analog does not engage in the extended hydrogen bonding interactions and it has a viscosity several orders of magnitude lower than that of BisGMA .
Hydrogen bonding interactions associated with urethane functionality, such as is found in urethane dimethacrylate (UDMA; 1,6-bis(methacryloxy-2-ethoxycarbonylamino)-2,4,4-trimethylhexane) are significantly weaker than those based on hydroxyl groups as shown by the conversion of BisGMA to a series of urethane-containing dimethacrylate monomers with greatly reduced viscosities ( Fig. 1 ) . That prior study also highlighted another interesting attribute of BisGMA. In spite of the much lower degree of conversion achieved during the homopolymerization of BisGMA relative to the lower viscosity (higher molecular mobility) urethane derivatives, the mechanical strength was greatest for the BisGMA polymer as a result of the strong hydrogen bonding interactions that serve as non-covalent physical crosslinks in the network. The hydrogen bond strength of BisGMA-containing resins was also found to be unaffected by polymerization whereas the interactions involving urethane groups in UDMA-based polymers were modestly reduced compared to the monomeric state . Hydrogen bonding is transient in nature with cycling rates comparable to small molecule diffusion rates and well in excess of molecular diffusion processes in polymeric systems. Therefore, hydrogen bonding can serve a significant role in the reinforcement of polymeric network structures, especially in processes involving high strain rates. The practical approach of using reactive diluent comonomers to adjust a formulated resin viscosity to a specific target is readily predictable, although the viscosity of the mixture varies in logarithmic fashion with the composition rather than as a simple arithmetic mean of the individual constituent viscosities ( Fig. 2 ) . A similar logarithmic dependence is found between temperature and viscosity of a resin . The choice of monomers also alters the handling properties of a resultant composite paste. Obviously, the size, shape and composition of the filler or mixed fillers used, as well as the surface treatment applied to the fillers will also affect the paste rheology, but differences in monomer composition in terms of viscosity and functionality are factors in composite paste cohesion and how the paste adheres or releases from various surfaces at different shear rates. Studies have recently begun to focus on how subtle compositional variations significantly influence composite handling properties .
3
Network development
Prior to polymerization, monomers typically exist as homogeneous liquid solutions. While the glass transition temperature (Tg) of the final polymer network that is ultimately formed as a consequence of the crosslinking polymerization reaction is a critical parameter, it can be informative to look at how the Tg and modulus development evolves during the polymerization. These details then can help direct the design of new materials and process controls that will potentially deliver materials with improved properties and clinical performance. As already mentioned, individual monomers exhibit widely varied viscosities, and in a related manner, they also differ considerably in their monomeric Tg’s. Some monomers will undergo crystallization when cooled below room temperature; however, the majority of dimethacrylate monomers commonly utilized in dental resins become amorphous glassy structures upon cooling in bulk. TEGDMA, which has a low viscosity, also has a relatively low monomeric Tg: −80 °C; as determined by differential scanning calorimetry . In line with the progressively higher viscosities of BisEMA, UDMA and BisGMA, their monomeric Tg’s were likewise established at −42, −38 and −10 °C, respectively. For comonomer mixtures, the monomeric Tg is linearly additive since most monomer combinations yield homogeneous amorphous glasses upon cooling based on the principle that like dissolves like. However, some combinations that represent greater structural divergence between the monomers, such as BisGMA and long-chain aliphatic structures , can undergo phase separation as the temperature is reduced. These sorts of marginally thermodynamic compatible monomer formulations have potential application as resins in which polymerization-induced phase separation occurs to intentionally create heterogeneous polymer networks that display reduced degrees of polymerization shrinkage and stress development .
When a monomer or resin is photocured, the viscosity, modulus and glass transition temperature all increase as the proportion of free monomer is steadily reduced with respect to the growing polymer phase, which is composed of polymer backbone chains, crosslinks and pendant reactive groups in ratios that also vary dependent upon the degree of conversion. A demonstration of the progression in glass transition temperature profiles as a function of conversion is shown in Fig. 3 . Dynamic mechanical analysis (DMA) was used to obtain the tan δ plots of a series of partially cured BisGMA/TEGDMA polymers that are static representations of various extents of network formation. A photo-iniferter (XDT) was used in place of a conventional free radical initiator since the iniferter produces a thermally stable, dormant chain-end group after the photopolymerization is discontinued . Therefore, unlike free radically cured polymer networks that contain persistent trapped radicals , there is no thermally induced post-cure in the iniferter-based photopolymers when probed by the DMA technique. This gives a clear example of how the Tg, taken as the maximum of the tan δ peak, and polymer heterogeneity, taken as the breadth of the tan δ peak, evolve during the polymerization. For a polymer-based direct restorative material to be clinically successful, its operational Tg must exceed not only the imposed cure temperature but also the temperature excursions encountered in a moisture-rich oral environment that includes periodic high stress conditions. It is evident that the polymer Tg advances significantly beyond the cure temperature primarily as a result of the polymer network heterogeneity . Dimethacrylate network heterogeneity is well established with initial formation of independent microgel domains advancing ahead of the surrounding matrix structure that subsequently fills in with progressively denser network structure. This creates microscopic localized gradient regions of higher and lower crosslink density that vary in proportion throughout the polymerization process such that there are glassy regions at relatively low levels of conversion as well as rubbery regions even at the limiting conversion when the Tg is well above the curing temperature.
Gelation can be determined by a variety of techniques with photorheology being a particularly useful experimental technique to apply to measure not only gelation, but also the full range of viscoelastic property development that occurs during polymerization. Several groups have implemented related versions of photorheometers that have been modified to permit simultaneous real-time conversion monitoring . This coupling of analytical techniques allows the gel point conversion to be accurately assigned as long as the issue of oxygen inhibition, which creates substantial edge effect problems in the rotational shear methodology of a parallel plate rheometry, is addressed. The photorheology indicates that the gel point is achieved at approximately 5% conversion in the homopolymerization of BisEMA under the reported conditions ( Fig. 4 ). The choice of monomer(s) and curing condition both have the potential to affect the gel point to modest degrees. A dimethacrylate monomer with a connecting group structure that is relatively compact and presents significant flexibility is more likely to engage in cyclization reactions than is a monomer that conveys a more rigid and extended linkage. Cyclization reactions entail a propagating chain radical reacting with a pendant reactive group either already attached to that same chain (primary cycle) or attached to another chain that has previously been connected to the first by a crosslink (secondary cycle). These cyclization reactions produce ineffective crosslinks and so their occurrence delays the gel point conversion compared with monomers that more efficiently form effective crosslinks. As an example, TEGDMA would be expected to have a higher gel point conversion than BisGMA since the former incorporates approximately three-fold more cyclization into the polymer structure . The number of reactive functional groups on a monomer also influences the gel point. In selecting a comonomer as a reactive diluent, the inclusion of a higher functionality monomer, such as trimethyloylpropane trimethacrylate rather than TEGDMA, would tend to promote an earlier gel point conversion due to the greater statistical likelihood of crosslink formation in the former. Use of a monomethacrylate does not avoid the potential for crosslink formation since chain transfer events involving both the monomeric and polymeric states can introduce crosslink formation, although at much lower concentrations than in dimethacrylate systems. A recent study using radical trapping agents provides evidence for radical production on multi-ethylene glycol segments of monovinyls and similar behavior involving the crosslinking segments of monomers such as TEGDMA and BisEMA may be likely as well.
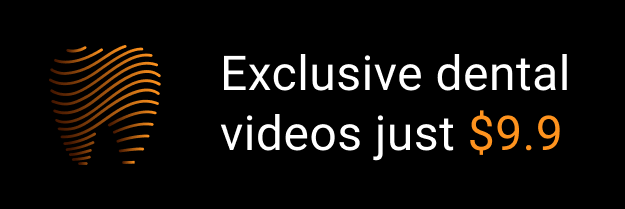