Graphical abstract

Highlights
- •
CHX-loaded nanoparticles showed desirable physicochemical and antibacterial characteristics.
- •
CHX-loaded PLGA nanoparticles proved the ability to gradually release CHX up to 28 days.
- •
Carried on aqueous solution, nanoparticles infiltrate through the dentinal tubules.
- •
Nanoparticles were closely associated to resin tags after bonding resins infiltration
Abstract
Objective
To characterize and deliver fabricated CHX-loaded PLGA-nanoparticles inside micron-sized dentinal-tubules of demineralized dentin-substrates and resin-dentin interface.
Methods
Nanoparticles fabricated by emulsion evaporation were assessed in-vitro by different techniques. Delivery of drug-loaded nanoparticles to demineralized dentin substrates, interaction with collagen matrix, and ex-vivo CHX-release profiles using extracted teeth connected to experimental setup simulating pulpal hydrostatic pressure were investigated. Furthermore, nanoparticles association/interaction with a commercial dentin-adhesive applied to demineralized dentin substrates were examined.
Results
The results showed that the formulated nanoparticles demonstrated attractive physicochemical properties, low cytotoxicity, potent antibacterial efficacy, and slow degradation and gradual CHX release profiles. Nanoparticles delivered efficiently inside dentinal-tubules structure to sufficient depth (>10 μm) against the simulated upward pulpal hydrostatic-pressure, even after bonding-resins infiltration and were attached/retained on collagen-fibrils. These results verified the potential significance of this newly introduced drug-delivery therapeutic strategy for future clinical applications and promote for a new era of future dental research.
Significance
This innovative drug-delivery strategy has proven to be a reliable method for delivering treatments that could be elaborated for other clinical applications in adhesive and restorative dentistry.
1
Introduction
Resin-dentin bonding is achieved through dentin demineralization to expose the underlying collagen-fibrils that facilitate resin-permeation into the filigree of mineral-deficit collagen-meshwork forming the transitional resin-dentin hybrid-interface and resin-tags inside dentinal-tubules . Several endogenous dentin-bound matrix metalloproteinases (MMPs) and/or bacterial enzymes were identified to participate in the breakdown of naked collagen-fibrils, drastically affecting the mechanical and structural integrity . Attempts targeting inhibition of MMPs and elimination of bacteria were undertaken, aiming to improve the longevity of resin-dentin bonded restorations . Accordingly, the potential of various MMPs inhibitors and antibacterial agents have been investigated for use in restorative procedures . Several studies concluded that the direct application of MMPs inhibitors and/or antibacterial agents to demineralized dentin collagen-matrix prior to application of dentin-adhesives to be a rational approach for enhancing the stability of resin-dentin interface . However, drawbacks emerged pertaining to the clinically irrelevant application-time and question of retention and maintenance of efficient therapeutic concentrations of the active drug/agent over prolonged periods, owing to continuous flow of dentinal-fluid across the structure of dentinal-tubules in the absence of an effective drug-releasing source over relatively longer time-periods.
Such challenges provide rationale for development of engineered nano-size drug-delivery carriers with tremendous potential for dose-dependent drug-release to demineralized dentin-substrates . In our previous work, CHX, a biguanide antimicrobial agent and non-specific MMPs inhibitor, encapsulated in PCL, was intended for drug-delivery to demineralized dentin substrates through micron-sized dentinal-tubules under pulpal-pressure simulated by an ex-vivo custom-built device . We have postulated, that the encapsulated drug following release from nano-carrier, will be directed by pulpal hydrostatic-pressure, toward the dentin collagen-fibrils and resin-dentin adhesive-interface through micron-sized dentinal-tubules and nano-sized collagen interfibrillar-spaces for prolonged exposure of drug to interact with the dentin-collagen matrix ( Fig. 1 Aa and Ab). However, in the current study, biocompatible and biodegradable polymer nanoparticles formed of poly-(lactic- co -glycolic acid) (PLGA), with smaller particle size, were investigated as CHX nanocarriers aiming to optimize nanoparticles infiltration and drug loading/release characteristics for potential applications in adhesive dentistry. Therefore, this study aimed to synthesize and characterize CHX-loaded/PLGA nanoparticles, for potential applications in restorative dentistry, in terms of physico-chemical characteristics, morphology, spectral features, thermal behavior, cytotoxicity, antibacterial susceptibility and in-vitro drug release kinetics. In addition, to investigate the delivery of the drug-loaded nanoparticles through dentinal-tubules of demineralized dentin substrates, their interaction with collagen matrix, and the ex-vivo CHX-release profiles using extracted teeth connected to experimental setup simulating pulpal pressure ( Fig. 1 Ca and Cb). Finally, the nanoparticles association/interaction with a commercially available dentin-adhesive, applied to demineralized dentin substrates, were investigated to scrutinize the potential for further clinical investigations.

2
Materials and methods
2.1
Materials and reagents
Chlorhexidine base (>99.5%), poly (lactic- co -glycolic acid) (PLGA; 75:25; Mw ∼66,000–110,000), dichloromethane, phosphate buffered saline (PBS), sodium hydroxide and polysorbate 80 were purchased from Sigma–Aldrich (St. Louis, MO, USA) and used without further purification. Brain-Heart infusion broth and agar were purchased from Thermo-Scientific (Oxoid Limited, Hampshire, UK). Adper Scotchbond™ Etchant (35% phosphoric acid gel), Adper™ Singlebond dentin adhesive system, restorative resin composite (Filtek Z350 XT A3) and micro-brushes were purchased from 3M ESPE (St. Paul, MN, USA). Omnipore Membrane Filter (pore size: 0.1 μm) was purchased from Merck (Millipore, USA). All chemicals were used without further purifications.
2.2
Preparation of nanoparticles
The specifications of fabrication of unloaded (Nano-PLGA/Blank) and chlorhexidine-loaded (Nano-PLGA/CHX) nanoparticles are illustrated in Fig. 1 Ba. The nanoparticles were prepared by emulsion evaporation. Briefly, organic phase consisting of PLGA and specified amounts of CHX (10, 25 and 50 mg) dissolved in dichloromethane was added drop-wise to the aqueous polysorbate 80 solution (0.5% w/v ) under homogenization. Subsequently, nanoparticles were concentrated by solvent evaporation and purified by centrifugation. Nano-PLGA/Blank nanoparticles were prepared using the same aforedescribed procedure, without the incorporation of CHX. Following recovery, dried nanoparticle powders were obtained and stored at 4 °C for further characterization.
2.3
Physico-chemical features
The synthesized nanoparticles were characterized, in-vitro , by different techniques as described in Fig. 1 Bb–Bd. The Nano-PLGA/Blank and Nano-PLGA/CHX (at PLGA/CHX ratios of 125:10, 125:25 and 125:50) were subjected to dynamic light scattering (DLS) (Malvern Mastersizer Nano ZS, UK) for determination of z -average particle-diameter, zeta-potential (ζ), size-distribution and polydispersity-index (PDI). Nanoparticles diluted in distilled water (1:100 wt/v ) were analyzed at 37 °C with a scattering angle of 90° (n = 13/group).
2.4
Morphological characterization of the nanoparticles
The morphological features, uniformity of particle-shape and size of Nano-PLGA/Blank and Nano-PLGA/CHX nanoparticles were verified by scanning (SEM) (Joel FESEM JSM-6700F, Japan) and transmission electron microscopy (TEM) (JEOL-JSM-1010, Japan). For SEM imaging, dried nanoparticles fixed on adhesive carbon tapes were gold sputter-coated (BAL-TEC, SCD 005 Sputter Coater, Scotia, NY, USA) and imaged at an accelerating voltage of 10 kV. For TEM examination, a droplet of aqueous nanoparticle dispersion (1:100 wt/v ) evaporated onto formvar/carbon-coated copper grids were proceeded for viewing. The 2D surface topography of dried nanoparticles fixed on silicon-wafers was assessed by Dimension ® Icon™ atomic force microscopy (AFM) (Bruker AXS, Germany) equipped with a proprietary ScanAsyst™ automatic. A silicon nitride cantilever with a resonance frequency of about 40–90 kHz and a spring constant of 0.4 N/m was used.
2.5
Chlorhexidine content
The percentage (%) of encapsulation-efficiency (EE) and drug-loading (DL) of nanoparticles was obtained indirectly by determining the amount of free non-encapsulated CHX in the dispersion medium. The non-encapsulated CHX was separated from loaded-CHX by centrifugation at 12,000 rpm at 25 °C for 15 min. The amount of free CHX was determined spectrophotometrically (UV-1700 Pharma Spec UV–vis Spectrophotometer, Shimadzu, Japan) (n = 15) at 289 nm. The %EE, %DL and % nanoparticle recovery were determined.
2.6
Spectral analysis
FTIR spectra of nanoparticles were recorded using PerkinElmer Spectrum RX-I (Waltham, MA) to detect structural features and confirm inclusion of CHX into PLGA nanoparticles. Dried nanoparticles (n = 7/group) were pelletized using KBr powder and spectra were acquired over a range of 4000–400 cm −1 at a resolution of 4 cm −1 . X-ray powder diffraction (XRD) analysis was carried out to confirm the crystalline phase of powdered nanoparticles (Rigaku Miniflex X-ray diffractometer, Tokyo, Japan) using Cu-Kα radiation (kα1 = 1.542 A°). Spectra were received over 2θ range with a step size of 0.02°. For analysis of surface elemental composition of nanoparticles by X-ray photoelectron spectroscopy (XPS), one drop of aqueous nanoparticle dispersion (1:100 w/v ) evaporated on clean silicon substrate was analyzed using Theta Probe Angle-Resolved X-ray Photoelectron Spectrometer System (Thermo Fisher Scientific, UK) equipped with a monochromatic Al Kα X-ray source and pass energy of 50 eV.
2.7
Thermal analysis
The relative change in percentage (%) weight of pure CHX, Nano-PLGA/Blank and Nano-PLGA/CHX nanoparticles with respect to changes in temperature was studied by thermogravimetric analysis (TGA) using TA instrument Discovery TGA Thermogravimetric analyzer (New Castle, DE, USA). About 10 mg of dry nanoparticles (n = 7/group) were heated in alumina crucibles from 30 to 800 °C at a heat flow rate of 10 °C/min under nitrogen spurge. Differential Scanning Calorimetry (DSC) was performed using a Mettler Toledo (DSC-1 system, Switzerland) to investigate the thermal property of dispersed drug inside polymer matrix. About 5 mg of samples were loaded onto a standard aluminum pan and then heated from 25 °C to 250 °C at a heat flow rate of 10 °C/min.
2.8
Cytotoxicity testing
Dental pulp stem cells (DPSCs; All-cells, Alameda, California, USA) cultured in DMEM (Dulbecco’s Modified Eagle’s Medium) were used for cytotoxicity studies. Cells were seeded at 1 × 10 4 cells/well (passage 4) in a 96-well plate, incubated overnight and exposed to 25, 50 and 75 μg/mL of Nano-PLGA/Blank and Nano-PLGA/CHX (at PLGA/CHX ratios of 125:10, 125:25 and 125:50) nanoparticles for 24, 48 and 72 h (n = 7/group at each concentration and incubation time). Untreated DPSCs without any treatment were used as control. The MTS [(3-(4,5-dimethylthiazol-2-yl)-5-(3-carboxymethoxyphenyl)-2-(4-sulfophenyl)-2H-tetrazolium)] reagent (CellTiter 96 AQueous One Solution Assay, Promega, USA) prepared in DMEM was added to each well followed by a 2 h incubation in 37 °C with 5% CO2. Absorbance was measured at λ = 490 nm using a microplate reader.
2.9
Antibacterial susceptibility
The antibacterial susceptibility of Nano-PLGA/Blank and Nano-PLGA/CHX nanoparticles were investigated by agar-disk diffusion method. The effect on three bacterial strains; Enterococcus faecalis (ATCC 29212), Streptococcus salivarius (ATCC 25975) and Streptococcus mutans (ATCC UA159) were investigated. The bacterial suspensions were prepared from the inoculum of overnight cultures and adjusted to OD 600 of 0.5 McFarland turbidity (∼10 8 bacteria/mL). The surface of Brain-Heart infusion (BHI) agar-plates was completely swabbed with bacterial suspensions and dried for 20 min. Sterile filter paper-disks impregnated with 25 μL of Nano-PLGA/Blank or Nano-PLGA/CHX (at PLGA/CHX ratios of 125:10, 125:25 and 125:50) nanoparticles (n = 9), were evenly-spaced on agar-plates and incubated at 37 °C for 48 h following which the diameter of growth inhibition-zones was recorded. The respective concentrations of CHX were used as corresponding positive-control.
2.10
Characterization of nanoparticles interaction with collagen-scaffolds
To study the attachment and interaction between collagen and Nano-PLGA/CHX nanoparticles, collagen-scaffold specimens were synthesized in-vitro using Collagen type-I solution (Rat Tail, Gibco InvitrogenTM; Lot # 423349; NY, USA) (4 mg/mL) supplemented with DMEM and 13–15 μL of 1 M sodium hydroxide, incubated at 37 °C overnight followed by desiccation for 2 d. Cell culture inserts (Greiner Thincert™, Germany) were used as a base for scaffold attachment and experiments were carried out in 24-well plates (Corning ® Costar ® , Flat bottom, NY, USA).
Nanoparticles suspended in distilled water (10% w/v ) relating to a total CHX content of 2.12% (Nano-PLGA/CHX 125:25) and 3.97% (Nano-PLGA/CHX 125:50) accounted from the drug loading into nanoparticles. About 25 μL of nanoparticle/water suspension (2/1 wt/v ), was added drop-wise for 60 s followed by application of gentle air-stream for 2 s. Specimens were left undisturbed and air-dried for 24 h for subsequent characterization. The morphology of nanoparticle-seeded collagen-scaffolds was examined by EDX/SEM elemental composition analysis (Joel JSM-6700F, Japan). The air-dried scaffold specimens were fixed on double-coated adhesive carbon tapes, gold sputter-coated (BAL-TEC, SCD 005 Sputter Coater, Scotia, NY, USA) under argon atmosphere and imaged at an accelerating voltage of 10 kV. Attenuated total reflectance Fourier transform infrared (ATR-FTIR) spectra of the nanoparticle-seeded collagen scaffolds (PerkinElmer Spectrum 2000 FTIR, Waltham, MA) were performed over a range 600–4000 cm −1 at a resolution of 4 cm −1 to investigate possible interactions between Nano-PLGA/CHX nanoparticles and collagen scaffolds.
2.11
Delivery of nanoparticles to demineralized dentin specimens
Sound human molars (21–35 years) were (NUS-IRB approval #2644) used in the ex-vivo experimental setup for investigating delivery of nanoparticles to demineralized dentin substrates through micron-sized dentinal-tubules ( Fig. 1 Cb and Cc). Following extraction, teeth were stored in 0.2% sodium azide at 4 °C to inhibit microbial growth and were used within 2 months from the time of extraction. Dentin specimens were prepared for nanoparticle-treatment as described previously . Following preparation, specimens were randomly grouped to be treated with Nano-PLGA/CHX nanoparticles (at PLGA/CHX ratios of 125:25 and 125:50) carried on distilled water at a nanoparticles/carrier ratio of 2/1 ( w/v ) for further investigations.
The exposed outer dentin-surfaces were etched with 35% phosphoric acid gel for 15 s, rinsed with distilled water for 15 s and dried with air-syringe for 2 s leaving the dentin surface slightly moist. The custom-built ex-vivo device was connected to simulate positive pulpal hydrostatic pressure (∼20 cm H 2 O) . The specifications of the experimental custom-built device and nanoparticles delivery is schematically demonstrated in Fig. 1 Ca–Cc. A drop-wise application of 25 μL of nanoparticles/carrier suspension to each dentin specimen for 60 s was followed by surface rubbing for 5 s with a micro-brush applicator. Following nanoparticles application, the dentin surface was left undisturbed for 15 s, gently air-blown for 3 s and blot-dried by absorbent paper to remove excess water. The dentin specimens were then prepared for SEM/TEM examination and resin/dentin bonding procedure.
2.12
Resin/Dentin bonding and SEM investigation
Following nanoparticles delivery to demineralized dentin-substrates, a 2-step etch-and-rinse dentin bonding system was applied according to manufacturer’s instructions ( Fig. 1 Ce). Subsequently, each tooth specimen was restored to a 4-mm resin composite crown in equal increments with each increment light-cured for 20 s (Curing Light 2500; 3M ESPE, MN, USA). The restored teeth specimens were stored in distilled water for 24 h at 37 °C to accelerate polymerization reaction followed by occluso-gingival sectioning into 1 mm slabs using a low-speed diamond saw under running water. Each obtained resin-dentin slab was polished with increasingly fine diamond pastes (2 μm and 1 μm) and cleaned ultrasonically for 10 min. Further, the slabs were air-dried for 48 h, gold sputter-coated and viewed by FESEM (Joel JSM-6700F, Japan).
2.13
CHX-release profiles characterization
For in-vitro cumulative CHX-release from different formulations of Nano-PLGA/CHX, nanoparticles, 15 mg of nanoparticles were suspended in 5 mL PBS buffer (pH 7.4) at 37 °C under slow stirring conditions. At pre-determined intervals, specimens were centrifuged at 12,000 rpm for 10 min and supernatant was analyzed spectrophotometrically (UV-1700, Shimadzu, Japan) at 289 nm using PBS as blank control. For ex-vivo study of CHX-release from Nano-PLGA/CHX nanoparticles (at formulations of 125:25 and 125:50) delivered to demineralized dentin-substrates, filter papers (pore size: 0.1 μm) were cut into (8 mm × 5 mm) oval-shaped strips and placed over acid-etched dentin surface of each dentin specimen. The areas of dentin specimen not covered by filter paper were sealed with nail varnish to standardize the dentin surface area through which CHX-release was monitored. The entire setup, carried on a glass support, was placed inside a container filled with 10 mL PBS (pH 7.4) ( Fig. 1 Cd). The amount of CHX-released from nanoparticles, delivered to dentin specimens, through filter paper to the outer PSB media was detected by analyzing the PBS spectrophotometrically as described previously . For both in-vitro and ex-vivo studies, the cumulative CHX-release was characterized at pre-determined intervals up to 28 d (n = 11).
2.14
Statistical analysis
Data are presented in terms of mean ± standard deviation values. Data were analyzed statistically (SPSS v.15) using one-way ANOVA followed by Tukey–Kramer post-hoc test at a chosen significance-level of P < 0.05.
2
Materials and methods
2.1
Materials and reagents
Chlorhexidine base (>99.5%), poly (lactic- co -glycolic acid) (PLGA; 75:25; Mw ∼66,000–110,000), dichloromethane, phosphate buffered saline (PBS), sodium hydroxide and polysorbate 80 were purchased from Sigma–Aldrich (St. Louis, MO, USA) and used without further purification. Brain-Heart infusion broth and agar were purchased from Thermo-Scientific (Oxoid Limited, Hampshire, UK). Adper Scotchbond™ Etchant (35% phosphoric acid gel), Adper™ Singlebond dentin adhesive system, restorative resin composite (Filtek Z350 XT A3) and micro-brushes were purchased from 3M ESPE (St. Paul, MN, USA). Omnipore Membrane Filter (pore size: 0.1 μm) was purchased from Merck (Millipore, USA). All chemicals were used without further purifications.
2.2
Preparation of nanoparticles
The specifications of fabrication of unloaded (Nano-PLGA/Blank) and chlorhexidine-loaded (Nano-PLGA/CHX) nanoparticles are illustrated in Fig. 1 Ba. The nanoparticles were prepared by emulsion evaporation. Briefly, organic phase consisting of PLGA and specified amounts of CHX (10, 25 and 50 mg) dissolved in dichloromethane was added drop-wise to the aqueous polysorbate 80 solution (0.5% w/v ) under homogenization. Subsequently, nanoparticles were concentrated by solvent evaporation and purified by centrifugation. Nano-PLGA/Blank nanoparticles were prepared using the same aforedescribed procedure, without the incorporation of CHX. Following recovery, dried nanoparticle powders were obtained and stored at 4 °C for further characterization.
2.3
Physico-chemical features
The synthesized nanoparticles were characterized, in-vitro , by different techniques as described in Fig. 1 Bb–Bd. The Nano-PLGA/Blank and Nano-PLGA/CHX (at PLGA/CHX ratios of 125:10, 125:25 and 125:50) were subjected to dynamic light scattering (DLS) (Malvern Mastersizer Nano ZS, UK) for determination of z -average particle-diameter, zeta-potential (ζ), size-distribution and polydispersity-index (PDI). Nanoparticles diluted in distilled water (1:100 wt/v ) were analyzed at 37 °C with a scattering angle of 90° (n = 13/group).
2.4
Morphological characterization of the nanoparticles
The morphological features, uniformity of particle-shape and size of Nano-PLGA/Blank and Nano-PLGA/CHX nanoparticles were verified by scanning (SEM) (Joel FESEM JSM-6700F, Japan) and transmission electron microscopy (TEM) (JEOL-JSM-1010, Japan). For SEM imaging, dried nanoparticles fixed on adhesive carbon tapes were gold sputter-coated (BAL-TEC, SCD 005 Sputter Coater, Scotia, NY, USA) and imaged at an accelerating voltage of 10 kV. For TEM examination, a droplet of aqueous nanoparticle dispersion (1:100 wt/v ) evaporated onto formvar/carbon-coated copper grids were proceeded for viewing. The 2D surface topography of dried nanoparticles fixed on silicon-wafers was assessed by Dimension ® Icon™ atomic force microscopy (AFM) (Bruker AXS, Germany) equipped with a proprietary ScanAsyst™ automatic. A silicon nitride cantilever with a resonance frequency of about 40–90 kHz and a spring constant of 0.4 N/m was used.
2.5
Chlorhexidine content
The percentage (%) of encapsulation-efficiency (EE) and drug-loading (DL) of nanoparticles was obtained indirectly by determining the amount of free non-encapsulated CHX in the dispersion medium. The non-encapsulated CHX was separated from loaded-CHX by centrifugation at 12,000 rpm at 25 °C for 15 min. The amount of free CHX was determined spectrophotometrically (UV-1700 Pharma Spec UV–vis Spectrophotometer, Shimadzu, Japan) (n = 15) at 289 nm. The %EE, %DL and % nanoparticle recovery were determined.
2.6
Spectral analysis
FTIR spectra of nanoparticles were recorded using PerkinElmer Spectrum RX-I (Waltham, MA) to detect structural features and confirm inclusion of CHX into PLGA nanoparticles. Dried nanoparticles (n = 7/group) were pelletized using KBr powder and spectra were acquired over a range of 4000–400 cm −1 at a resolution of 4 cm −1 . X-ray powder diffraction (XRD) analysis was carried out to confirm the crystalline phase of powdered nanoparticles (Rigaku Miniflex X-ray diffractometer, Tokyo, Japan) using Cu-Kα radiation (kα1 = 1.542 A°). Spectra were received over 2θ range with a step size of 0.02°. For analysis of surface elemental composition of nanoparticles by X-ray photoelectron spectroscopy (XPS), one drop of aqueous nanoparticle dispersion (1:100 w/v ) evaporated on clean silicon substrate was analyzed using Theta Probe Angle-Resolved X-ray Photoelectron Spectrometer System (Thermo Fisher Scientific, UK) equipped with a monochromatic Al Kα X-ray source and pass energy of 50 eV.
2.7
Thermal analysis
The relative change in percentage (%) weight of pure CHX, Nano-PLGA/Blank and Nano-PLGA/CHX nanoparticles with respect to changes in temperature was studied by thermogravimetric analysis (TGA) using TA instrument Discovery TGA Thermogravimetric analyzer (New Castle, DE, USA). About 10 mg of dry nanoparticles (n = 7/group) were heated in alumina crucibles from 30 to 800 °C at a heat flow rate of 10 °C/min under nitrogen spurge. Differential Scanning Calorimetry (DSC) was performed using a Mettler Toledo (DSC-1 system, Switzerland) to investigate the thermal property of dispersed drug inside polymer matrix. About 5 mg of samples were loaded onto a standard aluminum pan and then heated from 25 °C to 250 °C at a heat flow rate of 10 °C/min.
2.8
Cytotoxicity testing
Dental pulp stem cells (DPSCs; All-cells, Alameda, California, USA) cultured in DMEM (Dulbecco’s Modified Eagle’s Medium) were used for cytotoxicity studies. Cells were seeded at 1 × 10 4 cells/well (passage 4) in a 96-well plate, incubated overnight and exposed to 25, 50 and 75 μg/mL of Nano-PLGA/Blank and Nano-PLGA/CHX (at PLGA/CHX ratios of 125:10, 125:25 and 125:50) nanoparticles for 24, 48 and 72 h (n = 7/group at each concentration and incubation time). Untreated DPSCs without any treatment were used as control. The MTS [(3-(4,5-dimethylthiazol-2-yl)-5-(3-carboxymethoxyphenyl)-2-(4-sulfophenyl)-2H-tetrazolium)] reagent (CellTiter 96 AQueous One Solution Assay, Promega, USA) prepared in DMEM was added to each well followed by a 2 h incubation in 37 °C with 5% CO2. Absorbance was measured at λ = 490 nm using a microplate reader.
2.9
Antibacterial susceptibility
The antibacterial susceptibility of Nano-PLGA/Blank and Nano-PLGA/CHX nanoparticles were investigated by agar-disk diffusion method. The effect on three bacterial strains; Enterococcus faecalis (ATCC 29212), Streptococcus salivarius (ATCC 25975) and Streptococcus mutans (ATCC UA159) were investigated. The bacterial suspensions were prepared from the inoculum of overnight cultures and adjusted to OD 600 of 0.5 McFarland turbidity (∼10 8 bacteria/mL). The surface of Brain-Heart infusion (BHI) agar-plates was completely swabbed with bacterial suspensions and dried for 20 min. Sterile filter paper-disks impregnated with 25 μL of Nano-PLGA/Blank or Nano-PLGA/CHX (at PLGA/CHX ratios of 125:10, 125:25 and 125:50) nanoparticles (n = 9), were evenly-spaced on agar-plates and incubated at 37 °C for 48 h following which the diameter of growth inhibition-zones was recorded. The respective concentrations of CHX were used as corresponding positive-control.
2.10
Characterization of nanoparticles interaction with collagen-scaffolds
To study the attachment and interaction between collagen and Nano-PLGA/CHX nanoparticles, collagen-scaffold specimens were synthesized in-vitro using Collagen type-I solution (Rat Tail, Gibco InvitrogenTM; Lot # 423349; NY, USA) (4 mg/mL) supplemented with DMEM and 13–15 μL of 1 M sodium hydroxide, incubated at 37 °C overnight followed by desiccation for 2 d. Cell culture inserts (Greiner Thincert™, Germany) were used as a base for scaffold attachment and experiments were carried out in 24-well plates (Corning ® Costar ® , Flat bottom, NY, USA).
Nanoparticles suspended in distilled water (10% w/v ) relating to a total CHX content of 2.12% (Nano-PLGA/CHX 125:25) and 3.97% (Nano-PLGA/CHX 125:50) accounted from the drug loading into nanoparticles. About 25 μL of nanoparticle/water suspension (2/1 wt/v ), was added drop-wise for 60 s followed by application of gentle air-stream for 2 s. Specimens were left undisturbed and air-dried for 24 h for subsequent characterization. The morphology of nanoparticle-seeded collagen-scaffolds was examined by EDX/SEM elemental composition analysis (Joel JSM-6700F, Japan). The air-dried scaffold specimens were fixed on double-coated adhesive carbon tapes, gold sputter-coated (BAL-TEC, SCD 005 Sputter Coater, Scotia, NY, USA) under argon atmosphere and imaged at an accelerating voltage of 10 kV. Attenuated total reflectance Fourier transform infrared (ATR-FTIR) spectra of the nanoparticle-seeded collagen scaffolds (PerkinElmer Spectrum 2000 FTIR, Waltham, MA) were performed over a range 600–4000 cm −1 at a resolution of 4 cm −1 to investigate possible interactions between Nano-PLGA/CHX nanoparticles and collagen scaffolds.
2.11
Delivery of nanoparticles to demineralized dentin specimens
Sound human molars (21–35 years) were (NUS-IRB approval #2644) used in the ex-vivo experimental setup for investigating delivery of nanoparticles to demineralized dentin substrates through micron-sized dentinal-tubules ( Fig. 1 Cb and Cc). Following extraction, teeth were stored in 0.2% sodium azide at 4 °C to inhibit microbial growth and were used within 2 months from the time of extraction. Dentin specimens were prepared for nanoparticle-treatment as described previously . Following preparation, specimens were randomly grouped to be treated with Nano-PLGA/CHX nanoparticles (at PLGA/CHX ratios of 125:25 and 125:50) carried on distilled water at a nanoparticles/carrier ratio of 2/1 ( w/v ) for further investigations.
The exposed outer dentin-surfaces were etched with 35% phosphoric acid gel for 15 s, rinsed with distilled water for 15 s and dried with air-syringe for 2 s leaving the dentin surface slightly moist. The custom-built ex-vivo device was connected to simulate positive pulpal hydrostatic pressure (∼20 cm H 2 O) . The specifications of the experimental custom-built device and nanoparticles delivery is schematically demonstrated in Fig. 1 Ca–Cc. A drop-wise application of 25 μL of nanoparticles/carrier suspension to each dentin specimen for 60 s was followed by surface rubbing for 5 s with a micro-brush applicator. Following nanoparticles application, the dentin surface was left undisturbed for 15 s, gently air-blown for 3 s and blot-dried by absorbent paper to remove excess water. The dentin specimens were then prepared for SEM/TEM examination and resin/dentin bonding procedure.
2.12
Resin/Dentin bonding and SEM investigation
Following nanoparticles delivery to demineralized dentin-substrates, a 2-step etch-and-rinse dentin bonding system was applied according to manufacturer’s instructions ( Fig. 1 Ce). Subsequently, each tooth specimen was restored to a 4-mm resin composite crown in equal increments with each increment light-cured for 20 s (Curing Light 2500; 3M ESPE, MN, USA). The restored teeth specimens were stored in distilled water for 24 h at 37 °C to accelerate polymerization reaction followed by occluso-gingival sectioning into 1 mm slabs using a low-speed diamond saw under running water. Each obtained resin-dentin slab was polished with increasingly fine diamond pastes (2 μm and 1 μm) and cleaned ultrasonically for 10 min. Further, the slabs were air-dried for 48 h, gold sputter-coated and viewed by FESEM (Joel JSM-6700F, Japan).
2.13
CHX-release profiles characterization
For in-vitro cumulative CHX-release from different formulations of Nano-PLGA/CHX, nanoparticles, 15 mg of nanoparticles were suspended in 5 mL PBS buffer (pH 7.4) at 37 °C under slow stirring conditions. At pre-determined intervals, specimens were centrifuged at 12,000 rpm for 10 min and supernatant was analyzed spectrophotometrically (UV-1700, Shimadzu, Japan) at 289 nm using PBS as blank control. For ex-vivo study of CHX-release from Nano-PLGA/CHX nanoparticles (at formulations of 125:25 and 125:50) delivered to demineralized dentin-substrates, filter papers (pore size: 0.1 μm) were cut into (8 mm × 5 mm) oval-shaped strips and placed over acid-etched dentin surface of each dentin specimen. The areas of dentin specimen not covered by filter paper were sealed with nail varnish to standardize the dentin surface area through which CHX-release was monitored. The entire setup, carried on a glass support, was placed inside a container filled with 10 mL PBS (pH 7.4) ( Fig. 1 Cd). The amount of CHX-released from nanoparticles, delivered to dentin specimens, through filter paper to the outer PSB media was detected by analyzing the PBS spectrophotometrically as described previously . For both in-vitro and ex-vivo studies, the cumulative CHX-release was characterized at pre-determined intervals up to 28 d (n = 11).
2.14
Statistical analysis
Data are presented in terms of mean ± standard deviation values. Data were analyzed statistically (SPSS v.15) using one-way ANOVA followed by Tukey–Kramer post-hoc test at a chosen significance-level of P < 0.05.
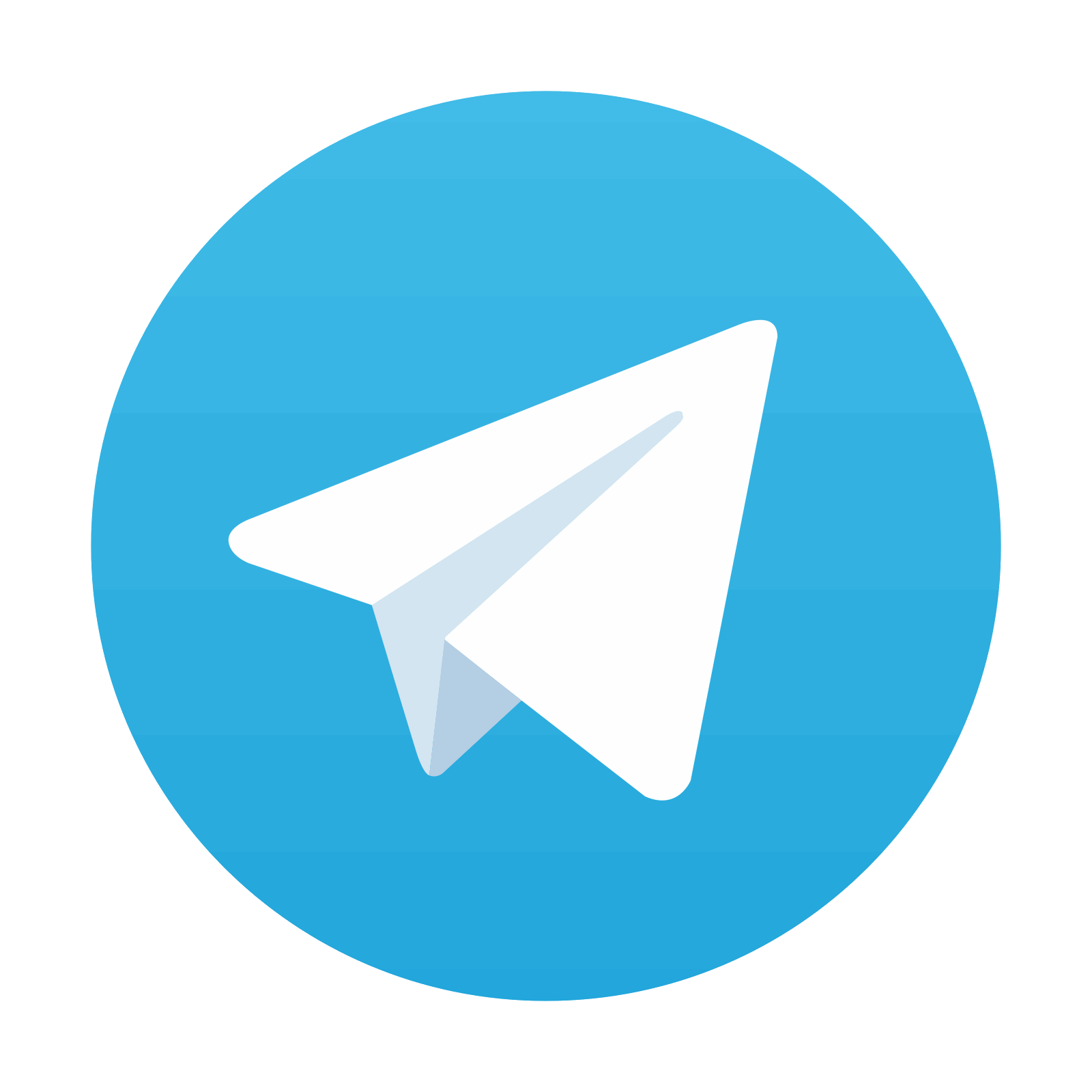
Stay updated, free dental videos. Join our Telegram channel

VIDEdental - Online dental courses
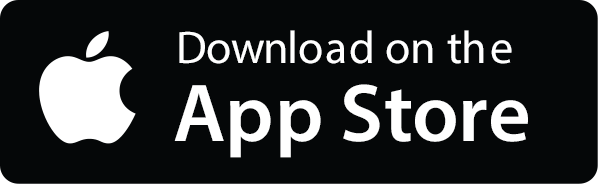
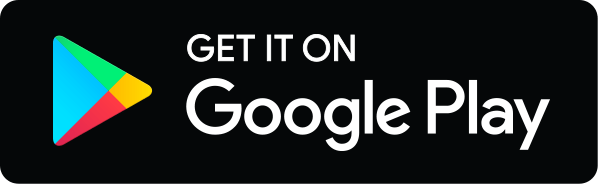