Graphical abstract

Highlights
- •
Triclosan nanoparticles can be incorporated into halloysite nanotube fillers.
- •
Halloysite incorporation (10 wt%) do not alter physicochemical properties.
- •
Halloysite nanotube fillers promote mineral deposition.
Abstract
Objective
This study aimed to assess the degree of conversion, microhardness, solvent degradation, contact angle, surface free energy and bioactivity (e.g., mineral precipitation) of experimental resin-based materials containing, pure or triclosan-encapsulated, aluminosilicate-(halloysite) nanotubes.
Methods
An experimental resin blend was prepared using bis-GMA/TEGDMA, 75/25 wt% (control). Halloysite nanotubes (HNT) doped with or without triclosan (TCN) were first analyzed using transmission electron microscopy (TEM). HNT or HNT/TCN fillers were incorporated into the resin blend at different concentrations (5, 10, and 20 wt%). Seven experimental resins were created and the degree of conversion, microhardness, solvent degradation and contact angle were assessed. Bioactive mineral precipitation induced by the experimental resins was evaluated through Raman spectroscopy and SEM-EDX.
Results
TEM showed a clear presence of TCN particles inside the tubular lumen and along the outer surfaces of the halloysite nanotubes. The degree of conversion, surface free energy, microhardness, and mineral deposition of polymers increased with higher amount of HNTs. Conversely, the higher the amount (20 wt%) of TCN-loaded HNTs the lower the microhardness of the experimental resins.
Significance
The incorporation of pure or TCN-loaded aluminosilicate-(halloysite) nanotubes into resin-based materials increase the bioactivity of such experimental restorative materials and promotes mineral deposition. Therefore, innovative resin-based materials containing functional halloysite-nanotube fillers may represent a valuable alternative for therapeutic minimally invasive treatments.
1
Introduction
Dental caries represents one of the main reasons for placement and replacement of resin composite restorations . Minimally invasive treatment of carious lesions in dentin requires selective removal of infected tissue, leaving as much caries-affected dentin as possible for therapeutic remineralization .
The advent of the latest generation of adhesive systems have radically influenced modern operative management of dental caries in minimally invasive dentistry (MID), in particular during atraumatic restorative treatments (ART) of deep carious lesions. Moreover, the application of adhesive systems on demineralized dentin (e.g. H 3 PO 4 -etched dentin), followed by placement of esthetic light-curing resin composites represents the main clinical procedure performed daily by many general dentists .
The main concern in MID/ART may be associated to the “poor” durability of the resin–dentin interface. The reason is that such esthetic restorations have no therapeutic antibacterial and/or remineralization effect on caries-affected dentin . Hence, it is still necessary to generate new types of resin-based restorative materials with enhanced therapeutic properties at the resin–dentin interface, which can also prevent the recurrence of secondary caries .
Triclosan (TCN) is a typical agent used in several antibacterial products such as toothpastes, mouth-rinse solutions and detergents. TCN seems to be particularly active against gram-positive microorganisms, such as Streptococcus mutans ; one of the main pathogens, along with S. aureus , Lactobacillus spp., and Actinomyces spp., involved in dental caries process . Because of its antimicrobial effectiveness, small size and easy processing, the use of TCN has constantly increased over the last 30 years .
The development of polymer-based adhesives with advanced physicochemical and biological properties represents a key target for researchers and dental manufacturers in order to develop innovative restorative materials that can increase the durability of the resin–dentin interface and impede the formation of secondary caries . It is well known that the inclusion of inorganic fillers into the polymeric matrix decreases the polymer ratio, leading to an improvement in the mechanical properties of resin composite materials and to the reduction of the polymerization stress and shrinkage in such restorative materials . Nevertheless, the excessive incorporation of fillers into resin-based materials may result in an interference with the penetration of light energy during light-curing procedures, thus hindering chain mobility in the propagation phase and therefore jeopardizing their final degree of conversion (DC) .
Halloysite nanotubes (HNTs), aluminosilicate nanoclay (Al 2 Si 2 O 5 (OH) 4 · n H 2 O) fillers, may be easily incorporated into different co-monomer resin blends . This unique filler is characterized by an outer surface made of Si–O bonds along with few silanols at the crystal defect sites. The inner walls of HNTs are mainly characterized by aluminols. Unlike traditional silica fillers, HNTs are able to promote a better polymerization rate of methacrylates , due to a special coordination between the carbonyl groups of the monomers and the electron deficient atom of aluminum (Al) in the nanoclay .
HNTs have hollow tubules with an inner and outer diameter in the range of 10–40 and 40–70 nm, respectively . Therefore, many chemically and biologically active substances such as tetracycline, khellin and nicotinamide adenine dinucleotide have been loaded into HNTs by soaking in a saturated drug solution under vacuum . The drug released from the HNTs can last 30–100 times longer than the drug alone or in other carriers .
The objective of this study was to perform a series of tests to assess the physicochemical properties of seven experimental dental resin-based materials containing different concentrations of aluminosilicate-(halloysite) nanotubes doped with or without triclosan. Moreover, the bioactivity of such experimental materials was also assessed through Raman spectroscopy and SEM-EDX. The first hypothesis tested in this study was that the incorporation of different amounts (%wt) of HNT/TCN or HNT would confer bioactive properties to the experimental resin-based materials. The second hypothesis was that the incorporation of different amounts (%wt) of HNT/TCN or HNT would not alter the degree of conversion, microhardness, solvent degradation, contact angle and surface free energy of the experimental resin-based materials tested in this study.
2
Materials and methods
2.1
Preparation of TCN-loaded HNTs and transmission electron microscopy (TEM)
Halloysite nanoclay (HNT: Al 2 Si 2 O 5 (OH) 4 ·2H 2 O) was purchased (Sigma–Aldrich, St. Louis, MO, USA), sieved (<150 μm), and then immersed into a 95% acetone solution containing 5 wt% 3-metacryloxypropyltrimetoxysilane (FGM, Joinville, SC, Brazil) for 24 h at 110 °C. Subsequently, the silanized halloysite filler was mixed (ratio 1:1) with an antimicrobial 2,4,4-trichloro-2-hydroxydiphenyl ether (TCN: triclosan, Fagron, Rotterdam, SH, Netherlands). This treated filler was then dispersed (0.03 mg/mL) in absolute ethanol at 30 °C for 1 h under continuous sonication and subsequently dehydrated for 10 days in a desiccator at 30 °C.
The pure halloysite (HNT) and the TCN-loaded HNT (HNT/TCN) fillers were dispersed at 5% (0.03 mg/mL) in absolute ethanol for 24 h at 25 °C and finally stirred for 15 min. Three specimens were created by dispersing 20 μL and 10 μL of uranyl acetate on 400 mesh square copper grids (Electron Microscopy Sciences, Hatfield, PA, USA). These HNT and HNT/TCN specimens were imaged using the TEM (JEM 1200 EXll, JEOL, Tokyo, Japan) at 80–100 kV and a magnification ranged between 20,000× and 500,000×.
2.2
Preparation of experimental resin-based materials
A control resin blend was created by mixing 75 wt% bis-GMA (2,2-bis-[4-(hydroxyl-3-methacryloxy-propyloxy)phenyl] propane) and 25 wt% triethylene glycol dimethacrylate (TEGDMA) (Sigma–Aldrich, St. Louis, MO, USA) for 30 min under continuous sonication. Camphorquinone (CQ), ethyl 4-dimethylaminobenzoate (EDAB), and diphenyliodonium hexafluorophosphate (DPIHFP; Milwaukee, MI, USA) were added at 1 mol% to obtain a light-curable resin-based material. The structures of monomers and antibacterial agent employed in this study are shown in Fig. 1 .
Four different amounts (0%, 5%, 10%, and 20%, w/w) of pure HNT or HNT/TCN were incorporated (stirred for 1 h under continuous sonication) into the resin bland to create six experimental resins along with a control resin containing no filler; seven groups of materials were generated for this experimental study.
2.3
Degree of conversion (DC)
The degree of conversion (DC) of each experimental resin-based material was evaluated using Fourier Transform Infrared Spectroscopy (FTIR) spectrometer (Vertex 70, Bruker Optics, Ettlingen, Germany) equipped with Attenuated Total Reflectance (ATR), a horizontal diamond crystal, and a 45° mirror angle. Three specimens for each group were analyzed by directly applying the resin (3 μL) onto the crystal. The entire analysis was performed at a standardized room temperature of 23 ± 1 °C and 60 ± 1% relative humidity.
DC was calculated as described in a previous study based on the intensity of the C C stretching vibrations (peak height) at 1635 cm −1 and using the symmetric ring stretching at 1608 cm −1 from the polymerized and non-polymerized specimens. Light-curing activation was performed for 40 s using a light-emitting diode source (Radii SDI, Bayswater, VIC, Australia) with an irradiance value of 700 mW/cm 2 (determined with a digital radiometer: RD-7, Ecel, São Paulo, SP, Brazil). A custom-made support was used to hold the light-curing system at a standardized distance (2 mm) from the surface of the specimen, as described by Sauro et al. .
The IR-Solution software was used to set the standard parameters and to check the scanning in Happ-Genzel apodization function mode in a range of 4000–800 cm −1 , resolution of 4 cm −1 , and scanning time of 40 s. This setup allowed the acquisition of each single scan, every 0.7 s during resin photo-activation. The data was plotted and a sigmoidal curve fitting method was applied using linear regression. DC (%) × time (s) was plotted for each amount of HNT and HNT/TCN.
2.4
Microhardness assessment
Cylindrical resin specimens with standardized dimensions (thickness: 2 mm; diameter: 8 mm) were created using a polyvinylsiloxane mold . In brief, each tested resin was inserted into a mold and the outer surface was covered with a plastic strip and pressed with a glass slide to squeeze out any excess of resin before the light-curing procedures. The specimens were then light-cured for 20 s, removed from the mold, and finally hand-polished using 400#, 600# and 1200#-grits SiC abrasive papers under continuous water irrigation (30 s each paper-step).
Ten specimens were used for each experimental resin-based material. Half of the specimens from each group were stored for 24 h (dry storage) and the other half of the specimens were immersed in absolute alcohol for 2 h at 37 °C. Subsequently, all the specimens were subjected to the Knoop microhardness (KHN) assessment as described in previous investigations . KHN measurements were taken on both specimen’s surfaces using an indenter (HMV-2, Shimadzu, Tokyo, Japan) under a load of 490 N (50 gf) for 15 s. Ten KHN readings were obtained from each specimen; five indentations were performed on the upper and five on the bottom surface. The Knoop microhardness values (KHN: kg/mm 2 ) were recorded as the average of the ten indentations obtained from each specimen. The initial (KHN 1 ) values and those obtained after post-conditioning (KHN 2 ) were processed as KHN% by calculation the Δ between KHN 1 and KHN 2 .
2.5
Contact angle and surface free energy (SFE) measurement
Three specimens were created for each tested resin as described in the microhardness section. These were analyzed using an optical tensiometer (Theta, Biolin Scientific, Stockholm, Sweden) to measure the mean contact angle ( θ ) to distilled water (as a polar liquid) and to α-bromonaphtalene (as a dispersive liquid). The drop out size, drop rate, displacement rate, and speed dispersion of water or α-bromonaphtalene liquid were, respectively: 5.00 μL, 2.0 μL/s, 20.0 μL/s, and 50 mm/min; and 2.0 μL, 1.0 μL/s, 20.0 μL/s, and 50 mm/min. The test was performed over a period of 20 s and the static contact angle ( θ ) between the droplet and the solid surface was measured at a specific period time (10 s). The SFE calculation was performed following the OWRK/Fowkes* method (in mN/m), with the One-Attension software (Biolin Scientific, Stockholm, Sweden).
* γ l s = σ l + σ s − 2 ( σ l D ⋅ σ s D + σ l P ⋅ σ s P )
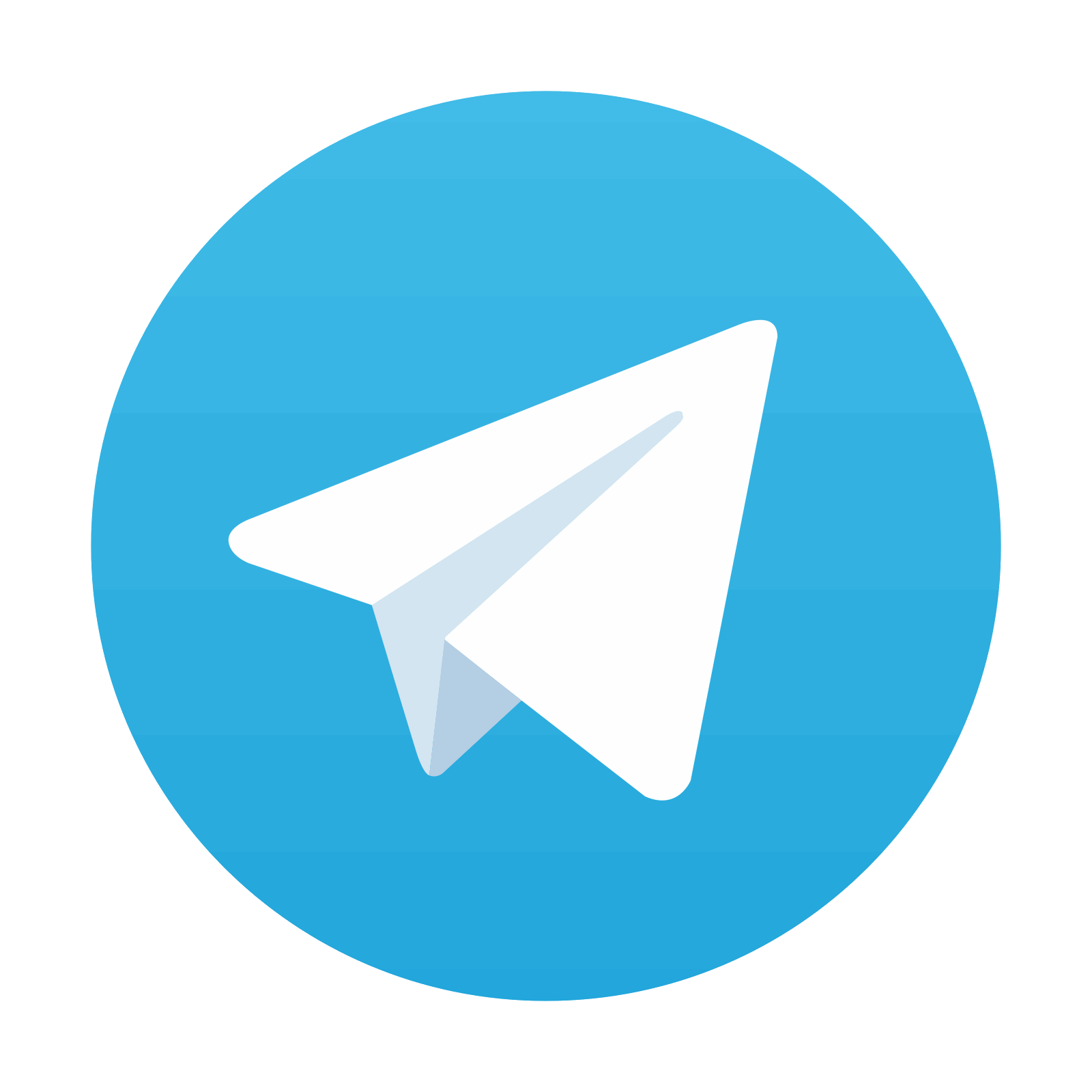
Stay updated, free dental videos. Join our Telegram channel

VIDEdental - Online dental courses
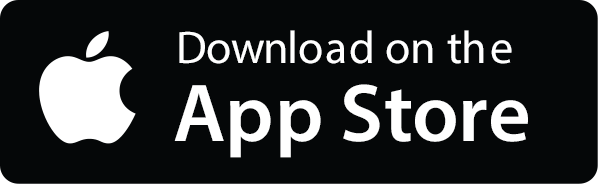
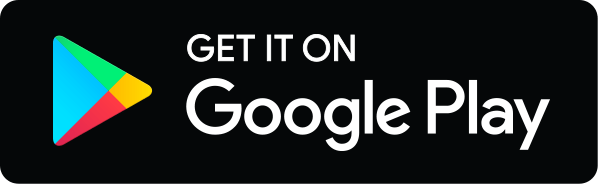