Introduction
The aim of this prospective randomized clinical trial was to quantitatively measure and compare the locations, dimensions, and volume of root resorption craters in human premolars after the application of controlled light and heavy rotational orthodontic forces over a 28-day (4-week) period.
Methods
Fifteen patients requiring bilateral extraction of maxillary first premolars as part of their orthodontic treatment were recruited for this study. Each patient received a heavy (225 g) rotational force on 1 premolar and a light (25 g) rotational force on the contralateral premolar. Orthodontic rotational forces were applied over 28 days with buccal and palatal cantilever springs; 0.016-inch beta-titanium molybdenum alloys were used to apply the light force and 0.018-inch stainless steel was used for the heavy force. After the 28-day experimental period, the upper first premolars were extracted under stringent protocols to prevent root surface damage. The samples were then scanned using a microcomputed tomography (micro-CT) scan x-ray system (SkyScan 1072, Skyscan, Aartselaar, Belgium), and analyzed using convex hull algorithm (CHULL2D; University of Sydney, Sydney, Australia) software to obtain direct volumetric measurements.
Results
The mean volume of resorption craters was 0.42 in the light force group and 0.51 in the heavy force group ( P = 0.013). When separated at the root level, the difference in volume of root resorption craters between the 2 groups was significantly different only at the midlevel ( P = 0.001). Root resorption craters were consistently detected at the boundaries between the buccal and distal surfaces and the mesial and lingual surfaces. The result supports our hypothesis that positive areas develop significantly more root resorption craters at all 3 levels, as compared with minimal areas (paired t test <0.001).
Conclusions
Heavy rotational forces caused more root resorption than light rotational forces and compression areas (buccal-distal and lingual-mesial surfaces in this study) showed significantly higher root resorption than other areas at all levels of the root.
Root resorption is intimately associated with the biological processes that occur during orthodontic tooth movement. This phenomenon is commonly known as orthodontically induced inflammatory root resorption (OIIRR) and is often unpredictable; it is an inevitable pathologic consequence of orthodontic tooth movement that compromises the success of orthodontic treatment.
The etiology of OIIRR is multifactorial. The causative factors may be of biological or mechanical origin and may act either individually or synergistically. Some mechanical factors include the magnitude of applied force, duration of force application, direction of force application, type of tooth movement induced, and type of orthodontic appliance used. These mechanical factors can be controlled to some extent by either the clinician or the patient, or both. Biological factors, however, are not within the control of the clinician; clinicians can only screen for high-risk patients. Such biological factors include systemic factors, gender, age, nutrition, previous trauma, and genetics and ethnicity.
Force magnitude is an important determining factor in the occurrence of root resorption. Magnitude of force is related to characteristics of the force system and the surface area of the periodontal ligament over which it is dissipated. An optimal force, from an orthodontic perspective, is characterized by a maximal cellular response (apposition and resorption of supporting tissues) while maintaining the vitality of the tissues. The use of light forces in order to reduce the risk of OIIRR has been advocated by Reitan. It has been proposed that if the force that induces orthodontic movement is greater than the partial pressure of the periodontal capillaries (26 g/cm 2 ), periodontal ischemia will occur,- which will lead to root resorption. King and Fischlschweiger reported, after a study of rat samples, that light forces cause minimal root resorption and rapid tooth movement, as opposed to heavy forces, which tend to result in slower tooth displacement and a substantial amount of root resorption.
The type and direction of tooth movement have a considerable role in OIIRR. Many studies have investigated tissue reaction after different directions of orthodontic tooth movement. Orthodontic movements such as intrusion, tipping, and torquing tend to cause root resorption as the root surface is directly compressed against the alveolar bone. Previous research of rotated teeth have mainly consisted of histologic studies to analyze tissue rearrangement and the fibrillar component of periodontal ligament using the light microscope. Orthodontic rotational corrections have been described as the most unstable and at highest risk of relapse after treatment. Histologic studies have shown that contraction of supragingival fibers is the primary cause of relapse of rotated teeth. Periodontal fibers tend to contract and rearrange themselves if the tooth is retained for a period of 2 to 3 months; however, supragingival fibers tend to continue contraction for an extended period of time. Teeth subject to rotational forces therefore need to be treated early and retained for a longer period of time. However, this exposes the tooth to a longer duration of orthodontic forces, potentially resulting in a greater amount of root resorption.
The investigation of root resorption has mainly been achieved through radiographs, light microscopy, and scanning electron microscopy (SEM). These methods use 2-dimensional measurement techniques that have inherent flaws, such as magnification error, and furthermore lack the function to quantify root resorption craters volumetrically. As root resorption is a 3D phenomenon, more accurate quantitative measurements may be achieved via the use of 3D techniques including SEM measurements and x-ray microtomography (micro-CT).
To date, there have been no studies that quantify, through 3D measurements, the volume of root resorption after the application of controlled orthodontic rotational forces.
This study employed a micro-CT system (SkyScan1072, Skyscan, Aartselaar, Belgium), which is designed for nondestructive and noninvasive 3D imaging of dental microstructures with high spatial resolution. It allows reconstruction of the teeth in 3 dimensions and can reproduce slices that are 5-μm thick.
The aim of the study was to quantitatively analyze the locations, dimensions, and volume of root resorption craters of human premolars after the application of controlled light (25 g) and heavy (225 g) rotational orthodontic forces over 28 days.
Material and methods
Thirty maxillary first premolar teeth (15 bilateral pairs) were collected from 15 adolescent patients who required extraction for orthodontic purposes. Patients were recruited from the Orthodontic Department of the Faculty of Dentistry from the Ondokuz Mayis University for full fixed orthodontic appliance therapy. Ethics approval was obtained from the Human Ethics Committee at the University of Sydney (The Human Ethics Review Committee-CSHAS) and Ondokuz Mayis University prior to commencement of the investigation. The adolescent patients (5 males and 10 females; age mean 14.15 years; age range 11.9 to 16.9 years) met the selection criteria as previously described. Written informed consent was obtained from all subjects.
Prior to the commencement of the study, standard orthodontic records were attained from all subjects. These included extraoral photographs (frontal, left and right profile, smiling, 45° degree left and right profiles), intraoral photographs (frontal, left and right buccal, maxillary and mandibular occlusal), and radiographs (orthopantomography, lateral cephalometry, periapical radiographs of upper first premolars). Each patient had an alginate impression taken of the maxillary arch. The impressions were poured in plaster for appliance construction.
The teeth samples were divided bilaterally into 2 groups (15 teeth in 1 group, with 15 corresponding contralateral teeth in the other group). Each left and right maxillary first premolar was exposed exclusively to either light (group 1, 25 g) or heavy (group 2, 225 g) controlled orthodontic rotational forces over the 28-day trial period. The clinical setup was performed indirectly in the laboratory and transferred to the patient in-clinic. The subjects’ teeth were bonded with SPEED brackets with a 0.022-in slot (Strite Industries, Cambridge, Ontario, Canada) on the buccal and lingual sides of the maxillary first premolar. A modified transpalatal arch was bonded with occlusal acrylic coverage on the maxillary first molars. Each acrylic stop had a SPEED bracket with a 0.022-in slot (Strite Industries) added on both the buccal and palatal surface. This served to provide anchorage support as well as disengage the occlusion to prevent possible disturbances arising from occlusal forces on the experimental teeth, thus allowing the subject to receive pure rotational forces and prevent nonrotational distortion of the spring. Self-ligating brackets were chosen to allow for standardized ligation of the experimental teeth to eliminate force decay that results from the use of elastomeric ligations. A 0.017-in × 0.025-in stainless steel wire was shaped to match the arch form and placed in the lingual SPEED brackets on the acrylic stops , to act as a transpalatal arch ( Fig 1 ).
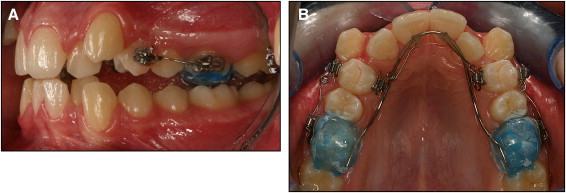
For the side receiving light (25 g) rotational forces, 2 0.016-in cantilever springs of beta-titanium–molybdenum alloy (TMA) were constructed on the model to provide a couple to rotate the tooth. The 0.016-in TMA (12.5 g) was placed from the palatal SPEED bracket to the transpalatal arch (TPA) and secured using composite resins. Another 0.016-in TMA (12.5 g) cantilever spring was placed from the SPEED bracket on the buccal surface of the acrylic stop to the premolar buccal SPEED bracket. The horizontal auxiliary slot of the SPEED bracket was used to stabilize the spring and prevent rotation in the slot. These 2 springs provided 2 forces of equal magnitude, applying parallel but noncolinear lines of force in opposite directions. This mechanical setup induces tooth rotation around its long axis. The side receiving heavy (225 g) controlled rotational forces had attachments set up the same way as the light-force side; however, cantilever arms made of 0.018-in stainless steel were used on each side to apply the higher force. The force magnitude was secured to the desired force levels with a strain gauge (Dentaurum, Ispringen, Germany).
One operator treated all patients during the experimental period of 28 days. Throughout this period no springs were reactivated. After the experimental period, the subjects were referred to oral surgeons for extraction of the upper first premolars. The surgeons were provided with comprehensive instructions in alignment with previously established protocols to avoid surgical trauma and to prevent damage to the root cementum. After extraction, the teeth were placed in individually marked containers of deionized water (Mille Q, Millipore, Bedford, Mass), which had previously been established to be an appropriate storage medium. The teeth were transferred to an ultrasonic bath for a period of 10 minutes to loosen all traces of residual periodontal ligament (PDL) and soft tissue fragments. A dampened gauze swab was used to remove all traces of residual PDL and soft tissue using a consistent rubbing motion, until all visible signs of the PDL were removed. The teeth were then disinfected in 70% alcohol for 30 minutes and restored in the container of deionized water. Prior to analysis, the teeth were bench dried for a minimum of 48 hours. Finally, root surfaces of the teeth were scanned using SkyScan 1072 desktop x-ray micro-tomography (SkyScan).
X-ray microtomography is a variant of the medical CT system that allows nondestructive imaging of the interior microstructure of materials; the images produced are of high spatial resolution. The associated software enables visual reconstructions of the complete internal microstructure of teeth and can reproduce complete 3D data sets with isotropic sample spacing down to a minimum space of approximately 5 μm. The x-ray detector consists of a 1024 × 1024 pixel 16-bit charged coupled device camera with fiberoptic cables coupled to an x-ray scintillator. All teeth were scanned from the cementoenamel junction to the tooth apex. Scanning was conducted with a 360° rotation around the vertical axis and a single rotation step of 45°. A total of 420 x-ray absorption radiographic images were acquired for each tooth and saved in 16-bit tagged image file format (TIFF) ( Fig 2 , A).
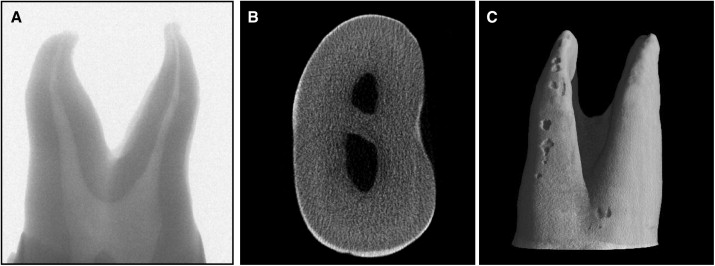
After acquisition of the images, an axial slice-by-slice reconstruction was performed with the designated software, NRecon (version 1.4.2, SkyScan) ( Fig 2 , B). Two-dimensional images were generated as 1024 × 1024 pixel bitmap (BMP) images having an 8-bit grayscale dynamic range. The axial 2D slices were then collated to form a 3D reconstruction of the images using VG Studio Max software (version 1.2, Volume Graphics, GmbH, Heidelberg, Germany) ( Fig 2 , C). The axial slice images of each isolated resorption crater were consolidated and exported to the convex hull 2D software (CHLL2D, University of Sydney, Sydney, Australia) to calculate resorption crater volume. The analysis follows the same methodology as that described in a previous study.
The volume measurement software (CHULL2D) is based on the application of a 2D convex hull that is generated for each axial slice of the crater data set. The convex hull algorithm assumes that the surface of the tooth is convex. When a crater is present, this assumption is not met and a break in the convexity is detected, thereby allowing detection so that the volume can be estimated. The convex hull algorithm (CHULL2D) reconstructs the loss of convexity by estimating a new line of closure that effectively connects the 2 points at the edge of the break, thus creating a closed subvolume for each axial slice of the crater. The total crater volume is calculated as the sum of the subvolumes for individual closed cross sections.
The 8 surfaces of the teeth (mesiobuccal, buccal, distobuccal, distal, distolingual, lingual, mesiolingual, mesial) were all analyzed in the study. Each surface was divided into thirds (cervical, middle, apical). Hence the root resorption craters are categorized into 24 surfaces. All the root resorption craters were acquired and recorded separately based on their location on the root surface. Each crater was measured individually, and the sum of the root resorption volumes for each tooth and surface type was obtained.
Statistical analysis
Analysis of variance (ANOVA) was used to identify significant differences between the 2 groups (light force group and heavy force group). Univariate analysis of variance was performed to investigate the effect of covariates (age and sex) and the volume of root resorption craters against root levels (cervical, middle, and apical). A paired t test was performed to evaluate the rotational hypothesis; surfaces where root resorption was expected to be high were compared to areas where root resorption was expected to be minimal. This was also analyzed at the 3 levels. The cube root of the volumetric readings was used to create a model for statistical analysis. This replaces each volume with the radius of an equivalent hemispheric crater. This approach has also been used in previous studies.
Results
Univariate ANOVA was performed with the Statistical Package for Social Sciences (SPSS, Chicago Ill). Similar to the previous study by Chan and Darendeliler, the cube root of volumetric readings was used to create a model for statistical analysis. This replaces each volume with the radius of an equivalent hemisphere crater. Factors evaluated in the analysis of variance model for amount of root resorption were (1) subject as a random factor, (2) force, surface types, and root division in thirds as fixed factors, and (3) amount of resorption as a response factor. The box plot in Figure 3 summarizes these results.
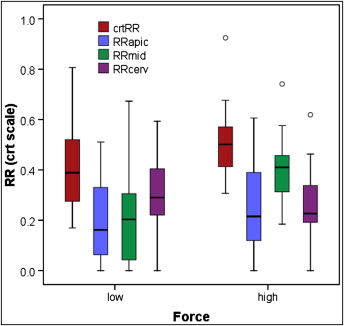
In the model comparing total root resorption craters in the heavy- and light-force groups, there was a statistically significant difference between the two groups ( P = 0.013). The mean volume of resorption craters in the heavy-force group was 0.51 and in the light-force group was 0.42 ( Table I ). When separated by root levels, the calculations indicate that a significant difference between the light- and heavy-force groups occurred at only the middle level (apical, P = 0.44; middle, P = 0.001; cervical, P = 0.40) ( Table II ). Age and sex variables did not change the results and were not assessed to be statistically significant with P values of 0.101 and 0.648, respectively.
Force | Mean | SE |
---|---|---|
Light (25 g) | 0.421 | 0.023 |
Heavy (225 g) | 0.511 | 0.023 |
Root level (thirds) | Mean (heavy force) | Mean (light force) | SE |
---|---|---|---|
Cervical | 0.258 | 0.306 | 0.039 |
Middle | 0.401 | 0.230 | 0.028 |
Apical | 0.250 | 0.208 | 0.038 |
Root resorption craters on all teeth exhibited similar distribution patterns. The craters consistently appeared at the boundaries between the buccal and distal surfaces and between the lingual and mesial surfaces. This appears to be in agreement with our hypothesis that root resorption craters were expected to develop on the mesiolingual, distobuccal, mesiobuccal, and distolingual , corresponding to positive areas of the root. In comparison, the mesial, lingual, distal, and buccal surfaces were expected to have minimal root resorption craters, corresponding to minimal areas of the root. Testing this hypothesis resulted in mean root resorption measures (all using the cube root scale) of 0.33, 0.35, 0.032, and 0.037 for the positive areas and 0, 0.003, 0, and 0.005 for the minimal areas. With a total mean of 0.75 for the positive areas and 0.008 for the minimal areas, the P value for the paired t test was <0.001 ( Table III ). A paired t test was also performed to compare the positive areas to the minimal areas when the root levels were divided into their cervical, middle, and apical thirds. The results showed that positive areas developed significantly more root resorption craters at all 3 levels compared with the minimal areas, with a paired t test value of <0.001 at all levels ( Table IV ).
Surface | Mean | SE |
---|---|---|
MLA + MLMi + MLC | 0.33 | 0.024 |
DBA + DBMi + DBC | 0.35 | 0.038 |
MBA + MBMi + MBC | 0.032 | 0.013 |
DLA + DLMi + DLC | 0.037 | 0.015 |
MA + MMi + MC | 0.000 | 0.000 |
LA + LMi + LC | 0.003 | 0.0028 |
DA + DMi + DC | 0.000 | 0.000 |
BA + BMi + BC | 0.005 | 0.005 |
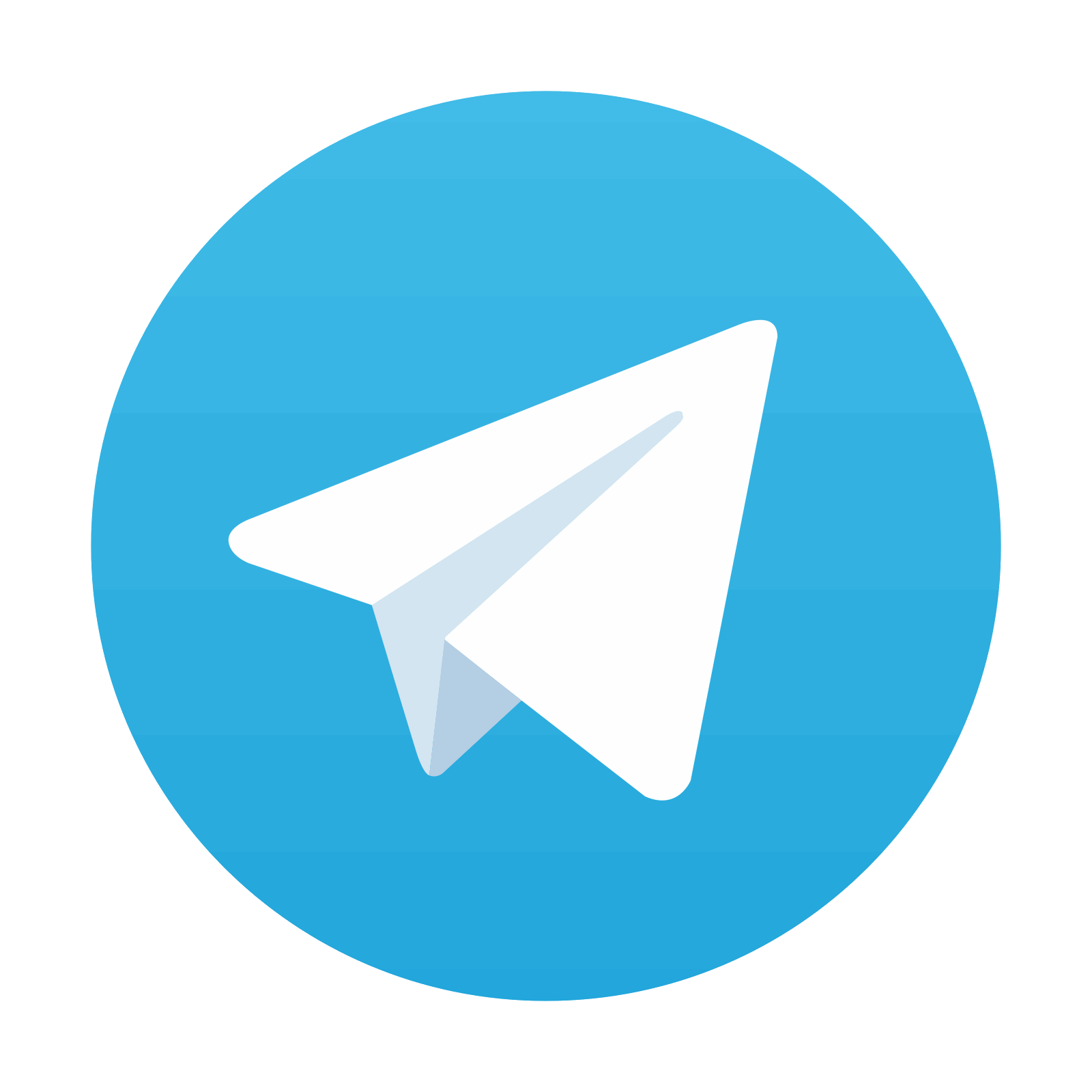
Stay updated, free dental videos. Join our Telegram channel

VIDEdental - Online dental courses
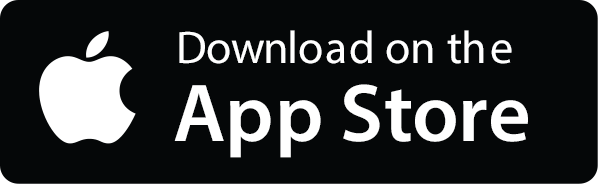
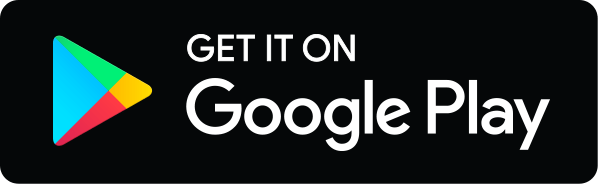
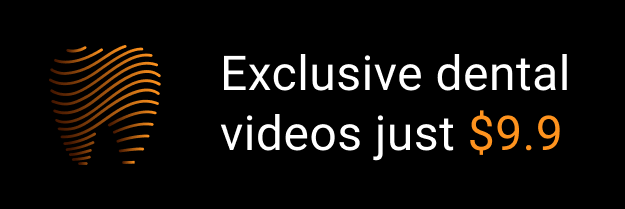