Abstract
Objectives
To distinguish the physical properties of a new silorane-based restorative material in comparison to five methacrylate-based restorative materials – a compomer, giomer, nanocomposite, hybrid and micro-hybrid.
Methods
The following properties were examined per restorative material: compressive strength, diametral tensile strength, flexural strength/modulus, fracture toughness, microhardness, and polymerization shrinkage. The mean and standard deviation were determined per group. A one-way ANOVA/Tukey was performed per property ( α = 0.05).
Results
Significant differences were found between groups per property ( p < 0.001).
Conclusions
Compared to the methacrylate-based restorative materials, the new silorane-based material had the lowest polymerization shrinkage, but an overall mixed mechanical performance. The silorane-based material had relatively higher flexural strength/modulus, fracture toughness, but relatively lower compressive strength and microhardness than the methacrylate-based restorative materials.
1
Introduction
With the constant evolution of tooth-colored restorative materials, evaluation of the material properties serves as a bridge between the fundamental material sciences and clinical applications . Although dental materials have undergone significant improvements, today’s methacrylate-based composites still have shortcomings that limit their applications . Two main motifs of these limitations involve the wear phenomena and polymerization stress . During polymerization, shrinkage may stress the adhesively placed tooth-colored restoration while it functions within the complex oral environment through mastication and temperature fluctuations . With the passage of time, wear, fatigue, and internal stress–strain from thermal contraction and expansion may create plastic deformation and marginal leakage and subsequently increase the risks of cuspal deflection, secondary-caries formation, and pulpal inflammation . Efforts to improve clinical performance and to diminish external deformation and internal stress of methacrylate-based composites have been focused on the development of innovative monomers, such as ring-opening siloranes; and new filler technology, such as nano-sized particles .
The formulation of methacrylate-based composites generally encompasses three main components: the inorganic filler particles, organic-resin matrix, and the coupling agent. The inorganic fillers are typically created from silicon derivatives and consist of particles such as glass, quartz, pyrogenic silicon dioxide, and colloidal silica via a sol–gel process . The organic matrix consists of base monomers, photoinitiators, pigments, and stabilizers. Bisphenol-A glycidyl methacrylate (Bis-GMA) and urethane dimethacrylate (UDMA) are commonly used as dental-base monomers . The coupling agent, usually silane, which is widely used to bond the inorganic filler to the organic-resin polymer, enhances the mechanical properties of the weaker resin polymer matrix and facilitates stress transfer by forming a unitary material . The vast majority of methacrylate-based restorative systems consist of a blend of micro- or nano-sized fillers with larger silicate- or zirconia-filler particles that are classified as either a hybrid, micro-hybrid, or nano-hybrid composite resin . Hybrid composites typically contain a mixture of inorganic filler particles (1–4 μm) and submicron particles (0.04 μm). Micro-hybrids combine smaller filler particles (0.1–1 μm) with the submicron particles. Nano-hybrids combine nanomeric particles (0.02 μm) and small filler particles, similar to micro-hybrids. Finally, nanocomposites utilize nano-sized particles throughout the resin matrix .
A unique nanocomposite recently introduced by 3M ESPE (St. Paul, MN) contains only nanomeric particles and nanoclusters as inorganic fillers . The nanomeric particles are monodispersed, nonagglomerated silica particles 20–75 nm in size. The nanocluster is a spheroidal agglomerate consisting of nano-sized (∼2 to 20 nm) silica and zirconia particles. The micron-sized porous cluster is infiltrated with silane coupling agents to allow chemical bonding with the organic matrix . The manufacturer claims that their new system is designed to produce the polish retention of microfill composites and the mechanical properties of hybrid composites .
Polyacid-modified resin composites or compomers were developed in an attempt to acquire the physical strengths of methacrylate-based composites and to retain the clinically attractive properties (e.g., fluoride release, coefficient of thermal expansion) of glass-ionomers . The majority of the components are identical to methacrylate-based composites, which contain dimethacrylate monomers and inorganic filler particles . However, compomers contain additional “ion-leachable glass” powder as found in glass-ionomer materials and special monomers with structures that are characterized by the presence of acidic functional groups and polymerizable methacrylate-based residues. The primary setting reaction is an addition polymerization. Subsequently, a secondary acid–base reaction occurs after the absorption of moisture into the anhydrous matrix. Thus, the compomer sets by various competing reactions, resulting in complex domains of different hydrophobic and hydrophilic phases, which may result in a reduction in the overall physical properties of these materials .
A giomer is a proprietary product exclusive to Shofu Dental Corporation (San Marcos, CA). Like a traditional methacrylate-based composite, the giomer chemical composition comprises inorganic filler particles and organic-resin matrix . Instead of applying purely glass or quartz as the typical fillers, the giomer incorporates inorganic fillers that are derived from the complete or partial reaction of ion-leachable glasses (e.g., fluoroboroaluminosilicate glasses) with polyalkenoic acids in water before being interfaced with the organic matrix .
Recently, a new silorane-based composite resin has been introduced with a distinctive polymerization characteristic to reduce polymerization shrinkage . The silorane matrix is formed by the cationic ring-opening polymerization of the silorane monomers. The “silorane” molecule represents a hybrid that is made of both siloxane and oxirane structural moieties . The introduction of a silorane-based composite opens new vistas in the quest to reduce polymerization shrinkage and to balance volumetric stress caused by the behavior of polymerization contraction. Even though preliminary findings for the silorane-based matrix indicate significant polymerization shrinkage reduction, research regarding the effect of the silorane monomers on the physical and mechanical characteristics of the cured composites has been limited .
With the development of a new polymerizing matrix, the silorane-based material necessitates classification in the continuum of tooth-colored resin-composite restorative materials. The objective of this study was to determine and to distinguish the unique physical properties (i.e., diametral tensile strength, compressive strength, flexural strength/modulus, fracture toughness, microhardness, polymerization shrinkage) of a new silorane-based restorative material in comparison to five methacrylate-based restorative materials consisting of a compomer, giomer, nanocomposite, hybrid, and micro-hybrid.
2
Methods and materials
Six representative restorative materials were selected as outlined in Table 1 . The materials were selected based on their representation of each of their respective restorative material types. The silorane-based restorative material (Filtek LS, 3M ESPE) is the first commercial prototype with a silorane formula . Beautifil-II (Shofu, San Marcos, CA) is the only available giomer. Filtek Supreme (3M ESPE) represents the only nanocomposite available with nano-sized particles throughout the resin matrix . Dyract eXtra (Dentsply, York, PA) is a representative compomer from a limited number currently available. There are well over 50 commercial hybrid composite resin restorative materials available on the market. The micro-hybrid, Esthet-X (Dentsply), and the hybrid, Filtek Z250 (3M ESPE), were selected based on their relative performance in multiple studies with comparison to various composite resins. Typically, the micro-hybrids demonstrate more moderate mechanical properties compared to hybrid composites . Diametral tensile strength, compressive strength, flexural strength, flexural modulus, fracture toughness, Knoop Hardness Number, and polymerization shrinkage were examined. Ten specimens were fabricated per restorative material for each test type.
Composite | Type | Manufacturer | Resin | Filler | Weight (%) | Volume (%) | Filler size |
---|---|---|---|---|---|---|---|
Beautifil-II | Giomer (G) | Shofu, San Marcos, CA, USA | Bisphenol-A glycidyl methacrylate (Bis-GMA), triethylene glycol dimethacrylate (TEGDMA) | S-PRG: surface reaction type pre-reacted glass-ionomer with fluoroaluminosilicate glass | 83.3 | 68.6 | 0.01–5 μm |
Dyract eXtra | Compomer (C) | Dentsply, York, PA, USA | Bis-GMA, urethane dimethacrylate (UDMA), carboxylic acid modified dimethacrylate (TCB), trimethylolpropane trimethacrylate (TMPTMA), TEGDMA | SAS-FPSG: strontium aluminosodium fluorophosphor silicate glass | 73 | 47 | Average: 0.8 μm |
Esthet-X | Micro-hybrid (MH) | Dentsply, York, PA, USA | Bis-GMA, bisphenol-A ethoxy dimethacrylate (Bis-EMA), TEGDMA | BAFSG: barium aluminofluoroborosilicate glass; silica dioxide | 77 | 60 | 0.02–2.5 μm |
Filtek LS | Silorane (S) | 3M/ESPE, St. Paul, MN, USA | Silorane | Quartz, yttrium fluoride | 76 | 55 | 0.04–1.7 μm |
Filtek Supreme | Nanocomposite (N) | 3M/ESPE, St. Paul, MN, USA | Bis-GMA, Bis-EMA, UDMA, TEGDMA | Zirconia, silica | 78.5 | 59.5 | Nano-particle (20–70 nm), Nanocluster (Average: 0.6 μm, particle: 2–20 nm) |
Filtek Z250 | Hybrid (H) | 3M/ESPE, St. Paul, MN, USA | Bis-GMA, Bis-EMA, UDMA, TEGDMA | Zirconia, silica | 78 | 60 | 0.01–3.5 μm |
2.1
Diametral tensile strength (DTS) and compressive strength (CS)
For DTS testing, an aluminum mold, 3 mm in length and 4 mm in diameter, was placed on a Mylar-strip-covered glass slide. The restorative materials were packed into the mold and completely filled. Then, the top circular surface of the mold was covered with a Mylar strip and glass slide. The top end of the specimen was exposed to a visible-light polymerization unit (Bluephase 16i, Ivoclar Vivadent, Amherst, NY) for 20 s at high irradiance (1600 mW/cm 2 ). The irradiance of the polymerization unit was monitored with a radiometer (LED Radiometer, Demetron, Middleton, WI). Next, the mold was turned, and the opposite end of the specimen was exposed to the light for 20 s. The specimen was removed from the mold and light cured on each longitudinal side of the cylindrical specimen for 20 s each – a total of 80 s for each specimen. The specimens were stored in 37 °C distilled water for 24 h. At 24 h, each specimen was tested by placement on its longitudinal side on the platens of a universal testing machine (Model 5543, Instron, Canton, MA). The specimens were loaded to failure in compression at a crosshead speed of 1.0 mm/min. The DTS was calculated based on peak load, diameter, and length of the specimen. CS testing was similar to DTS explained above except an aluminum mold 6-mm long and 4 mm in diameter was used to prepare the cylindrical specimens. Also, the specimens were loaded in the universal testing machine on the circular end of the cylindrical specimen. The compressive strength was calculated based on peak load and diameter of the specimen.
2.2
Flexural strength (FS) and modulus (FM)
To determine flexural strength and modulus, the restorative material was placed into an aluminum mold (2 mm × 2 mm × 25 mm) on a Mylar-strip-covered glass slide, according to ISO 4049 . The surface of the mold was covered as before and exposed to the light polymerization unit for 20 s each in five separate overlapping increments on both sides. The specimens were stored as before and tested on a three-point bending device using a universal testing machine as before at a crosshead speed of 0.25 mm/min. The flexural strength was calculated based on peak load, length, and area of specimen. Flexural modulus was determined from the slope of the linear region of the load–deflection curve.
2.3
Fracture toughness (FT)
Fracture toughness was determined by a single-edge notched-beam method . The restorative material was placed into a knife-edged split (2 mm × 2 mm × 25 mm) aluminum mold. The specimens were created and tested in a similar manner as flexural strength in a universal testing machine at a crosshead speed of 1 mm/min. The fracture toughness was calculated based on peak load, length, notch depth, and area of specimen.
2.4
Knoop microhardness (KHN)
For surface Knoop-microhardness testing, a polytetrafluoroethylene mold 2 mm in width and 8 mm in diameter was used to prepare each specimen as before. The restorative materials were packed into the mold and the top and bottom surfaces were exposed to the light polymerization unit for 20 s. The specimens were stored as before until testing using a diamond indenter (LM 300AT, Leco, St. Joseph, MI) with a 200-g load and a 10-s dwell time. Three hardness measurements were made for each specimen and averaged.
2.5
Polymerization shrinkage (PS)
To determine polymerization shrinkage, the specimens were shaped into a semi-sphere and placed on the polytetrafluoroethylene pedestal in the light path in a video-imaging device (AcuVol, Bisco, Schaumberg, IL). The specimens were digitized with the video camera and analyzed with the provided image-processing software. The specimens were allowed to rest for 3 min before being light cured for 40 s using the light polymerization unit. Polymerization shrinkage was recorded at 5 min after photoinitiation.
The mean and standard deviation were calculated for each of the six physical property tests for each of the six restorative materials. A one-way ANOVA followed by Tukey’s post hoc pairwise comparison test was used to determine if significant differences existed between the groups per test type at the 0.05 level of significance using statistical software (SPSS, version 10, Chicago, IL). For a one-way ANOVA with six groups and 10 specimens per group, the power was 80% to detect a moderate effect size (0.58 or 1.16 standard deviation difference) among means at the α level of 0.05.
2
Methods and materials
Six representative restorative materials were selected as outlined in Table 1 . The materials were selected based on their representation of each of their respective restorative material types. The silorane-based restorative material (Filtek LS, 3M ESPE) is the first commercial prototype with a silorane formula . Beautifil-II (Shofu, San Marcos, CA) is the only available giomer. Filtek Supreme (3M ESPE) represents the only nanocomposite available with nano-sized particles throughout the resin matrix . Dyract eXtra (Dentsply, York, PA) is a representative compomer from a limited number currently available. There are well over 50 commercial hybrid composite resin restorative materials available on the market. The micro-hybrid, Esthet-X (Dentsply), and the hybrid, Filtek Z250 (3M ESPE), were selected based on their relative performance in multiple studies with comparison to various composite resins. Typically, the micro-hybrids demonstrate more moderate mechanical properties compared to hybrid composites . Diametral tensile strength, compressive strength, flexural strength, flexural modulus, fracture toughness, Knoop Hardness Number, and polymerization shrinkage were examined. Ten specimens were fabricated per restorative material for each test type.
Composite | Type | Manufacturer | Resin | Filler | Weight (%) | Volume (%) | Filler size |
---|---|---|---|---|---|---|---|
Beautifil-II | Giomer (G) | Shofu, San Marcos, CA, USA | Bisphenol-A glycidyl methacrylate (Bis-GMA), triethylene glycol dimethacrylate (TEGDMA) | S-PRG: surface reaction type pre-reacted glass-ionomer with fluoroaluminosilicate glass | 83.3 | 68.6 | 0.01–5 μm |
Dyract eXtra | Compomer (C) | Dentsply, York, PA, USA | Bis-GMA, urethane dimethacrylate (UDMA), carboxylic acid modified dimethacrylate (TCB), trimethylolpropane trimethacrylate (TMPTMA), TEGDMA | SAS-FPSG: strontium aluminosodium fluorophosphor silicate glass | 73 | 47 | Average: 0.8 μm |
Esthet-X | Micro-hybrid (MH) | Dentsply, York, PA, USA | Bis-GMA, bisphenol-A ethoxy dimethacrylate (Bis-EMA), TEGDMA | BAFSG: barium aluminofluoroborosilicate glass; silica dioxide | 77 | 60 | 0.02–2.5 μm |
Filtek LS | Silorane (S) | 3M/ESPE, St. Paul, MN, USA | Silorane | Quartz, yttrium fluoride | 76 | 55 | 0.04–1.7 μm |
Filtek Supreme | Nanocomposite (N) | 3M/ESPE, St. Paul, MN, USA | Bis-GMA, Bis-EMA, UDMA, TEGDMA | Zirconia, silica | 78.5 | 59.5 | Nano-particle (20–70 nm), Nanocluster (Average: 0.6 μm, particle: 2–20 nm) |
Filtek Z250 | Hybrid (H) | 3M/ESPE, St. Paul, MN, USA | Bis-GMA, Bis-EMA, UDMA, TEGDMA | Zirconia, silica | 78 | 60 | 0.01–3.5 μm |
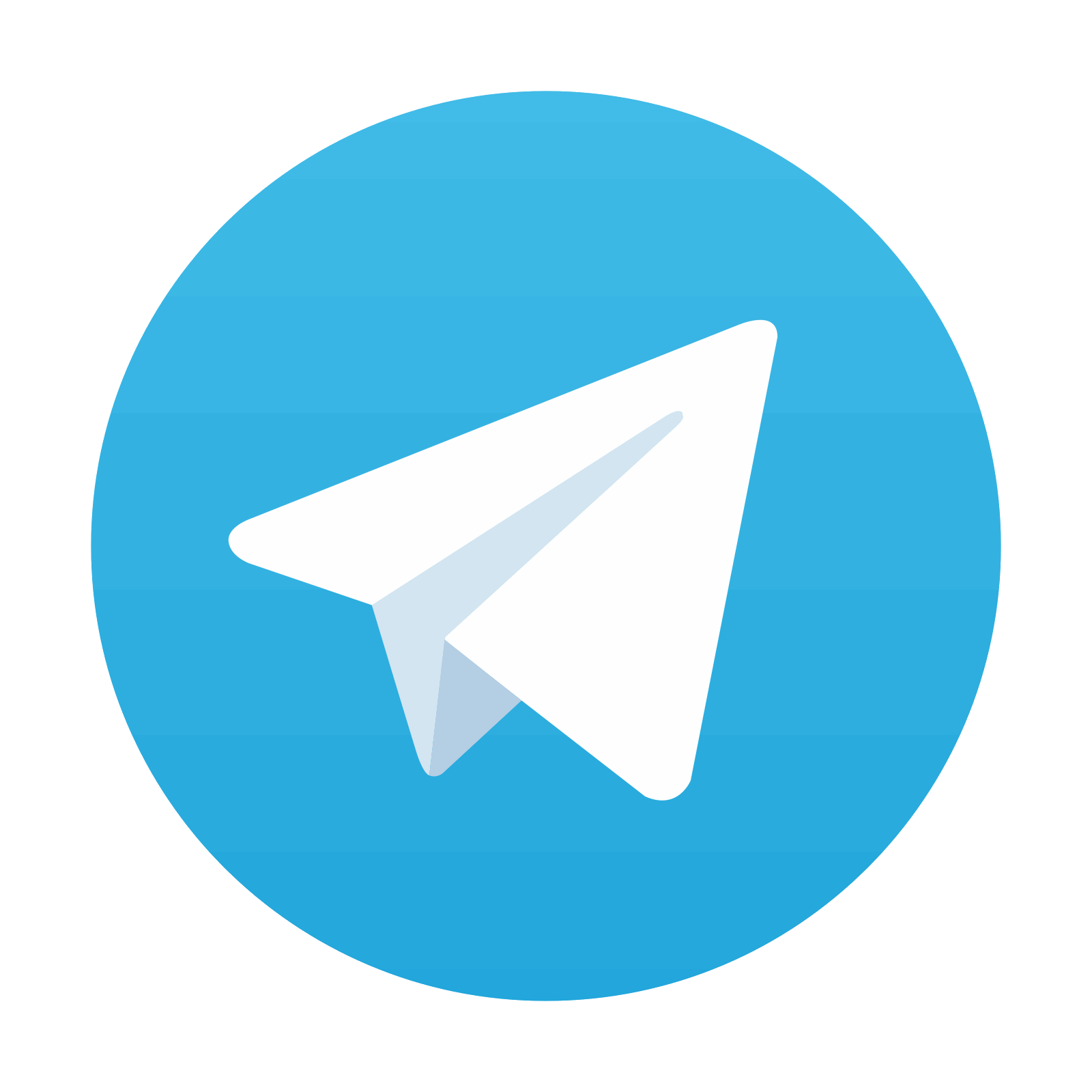
Stay updated, free dental videos. Join our Telegram channel

VIDEdental - Online dental courses
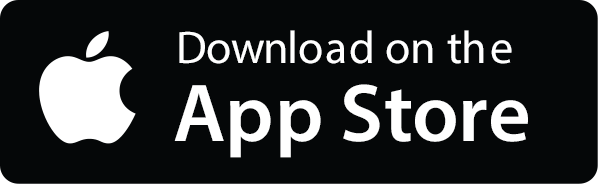
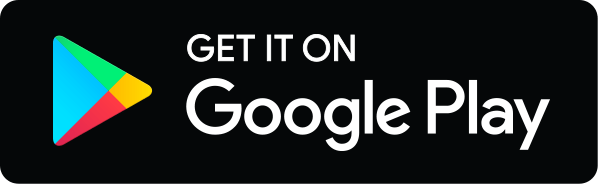
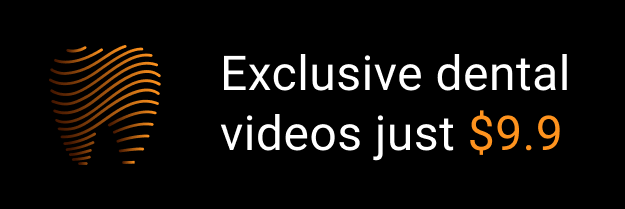